Plasmid Production in E. coli: Methodologies and Applications
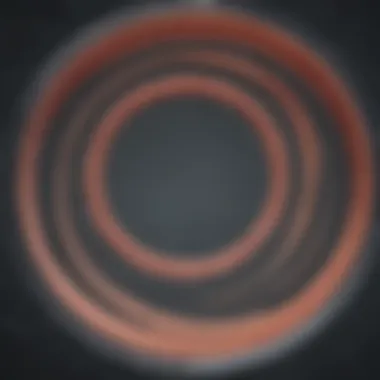
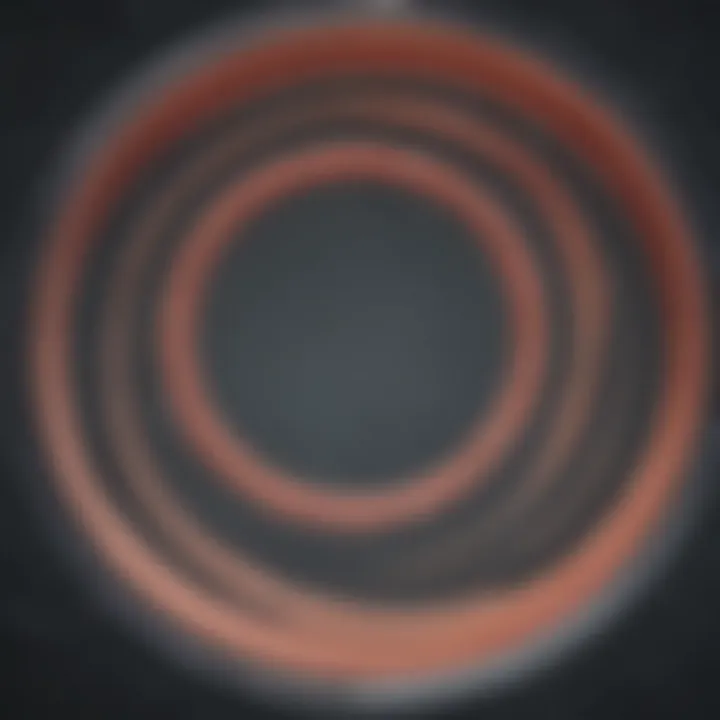
Intro
The subject of plasmid production in Escherichia coli holds significant importance in molecular biology and biotechnology. Plasmids, which are small, circular DNA molecules independent of chromosomal DNA, play a critical role in gene cloning, protein expression, and genetic manipulation. This article will explore methodologies employed for effective plasmid production and the various factors that can influence plasmid yield.
Understanding how E. coli can serve as a host for plasmid replication is crucial. The genetically engineered strains of E. coli are widely used in laboratories due to their resilience and fast growth rate. The ability to harness these microbes for plasmid production has implications that extend beyond basic research. Ultimately, the applications of plasmids touch a range of areas including medicine, agriculture, and environmental science.
The discussion will include key findings from research, implications of these methodologies, and a synthesis of current understanding in this dynamic field. Researchers, students, and professionals alike will benefit from this comprehensive narrative that addresses both theoretical and practical aspects of plasmid production in E. coli.
Foreword to Plasmids
Plasmids are vital components in molecular biology, particularly in the production and manipulation of genetic material. They serve as vectors for gene cloning and expression, enabling researchers to isolate and amplify specific DNA sequences. Understanding plasmids is critical to harnessing their potential in various applications, including biotechnology and genetic engineering. In this article, we focus on how Escherichia coli serves as an effective host for plasmid production.
Plasmids are extrachromosomal circular DNA molecules, which can replicate independently of chromosomal DNA. Their significance lies not only in their structural attributes but also in their ability to carry genes that confer advantageous traits, such as antibiotic resistance. These characteristics make plasmids formidable tools in genetic research and development.
Definition of Plasmids
A plasmid is a small, circular piece of DNA that exists outside the chromosomal DNA in a cell. Plasmids are found naturally in various organisms, including bacteria and yeast. They can be transferred between bacteria via conjugation, allowing for rapid adaptation to environmental changes. Their distinct structure allows them to replicate autonomously, which enhances their utility in cloning and genetic manipulation.
Types of Plasmids
Plasmids can be categorized based on their functionality and characteristics. The primary types include conjugative plasmids, non-conjugative plasmids, and cryptic plasmids.
Conjugative Plasmids
Conjugative plasmids possess the unique ability to transfer themselves from one bacterium to another through a process called conjugation. This feature is significant because it can result in the rapid spread of advantageous genes, such as those for antibiotic resistance, across bacterial populations. The key characteristic of conjugative plasmids is the presence of genes that facilitate this transfer.
Their benefit in research and industrial applications is notable; they are often used as vectors for gene cloning. However, their ability to promote horizontal gene transfer can also result in unintended spread of resistant traits among microorganisms.
Non-conjugative Plasmids
Non-conjugative plasmids lack the necessary genes for self-transfer. Instead, they rely on other mechanisms or plasmids for mobilization. Despite this limitation, they are widely utilized in cloning and expressing proteins in laboratory settings.
Their distinct characteristic is their simplicity, which often allows easier manipulation. The major advantage is their high stability within the host, minimizing the risk of unwanted gene transfer. However, this could also limit their adaptability in certain experimental contexts.
Cryptic Plasmids
Cryptic plasmids are those whose functions are not clearly understood. They do not carry genes that are easily identified as beneficial or detrimental to the host. Their contribution to the overall genetic landscape is still a subject of research.
Although they remain largely unexplored, their key characteristic is their unpredictable nature. This can be an advantage in research, serving as a source of genetic variation. On the downside, this unpredictability can make them less useful in applications requiring specific traits.
The Role of E. coli in Plasmid Production
Escherichia coli, commonly known as E. coli, plays a fundamental role in molecular biology, especially in the context of plasmid production. This bacterium serves as an ideal host organism for various genetic engineering applications. Understanding its role is essential for researchers, students, and professionals working in biotechnology and related fields.
Characteristics of E. coli
E. coli is characterized by several key features that make it a preferred choice for plasmid production. First, it has a relatively short generation time, allowing for rapid growth and high yield of plasmids. The standard laboratory strains, such as Dα and BL21, exhibit robust cloning capabilities. Their well-studied genetics facilitate precise manipulation, which is critical for successful plasmid construction.
Moreover, E. coli is a part of our normal gut flora, which gives it a harmless profile. This safety aspect is particularly significant when considering applications of recombinant DNA technology. In addition, E. coli can grow under both aerobic and anaerobic conditions, providing flexibility in handling various culture setups.
Advantages of Using E. coli
Utilizing E. coli for plasmid production brings numerous advantages to researchers.
- Ease of Genetic Manipulation: E. coli is amenable to various genetic manipulations, including transformation, that make it easier to introduce plasmids into the cells.
- Cost-effectiveness: Culturing E. coli is relatively inexpensive. The growth medium is readily available, and the costs associated with maintenance are low.
- High-Plasmid Yield: E. coli strains have been optimized over the years to maximize plasmid yield. Many strains can amplify plasmid DNA to levels suitable for various applications, including cloning and protein expression.
- Established Protocols: There are extensive protocols and resources available for working with E. coli. This wealth of information streamlines the research process and reduces the learning curve for new investigators.
In summary, the integration of E. coli into plasmid production processes is a cornerstone of biotechnology. Its unique characteristics and numerous advantages make it indispensable in the field of genetic engineering. By understanding these elements, researchers can effectively harness E. coli's capabilities to achieve their scientific objectives.
Genetic Engineering Techniques
Genetic engineering techniques play a critical role in the field of plasmid production, especially when using E. coli as a host. These methodologies enhance the ability to manipulate genetic material, thus enabling the successful cloning, expression, and analysis of plasmids. With the rising demand for genetic modifications in research and applied sciences, understanding these techniques becomes essential for researchers, students, and professionals alike.
One key aspect of genetic engineering in this context is the use of cloning vectors. Cloning vectors are DNA molecules used to transport foreign genetic material into a host cell. They can replicate independently within the host, allowing for the amplification of the genetic material of interest. The effective selection of appropriate vectors is vital for achieving high plasmid yields and function.
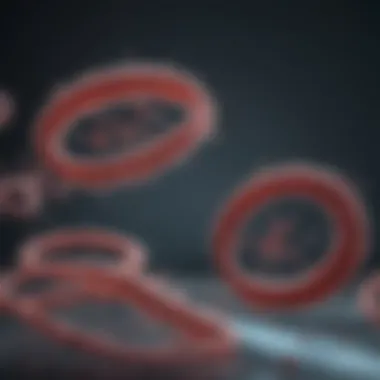
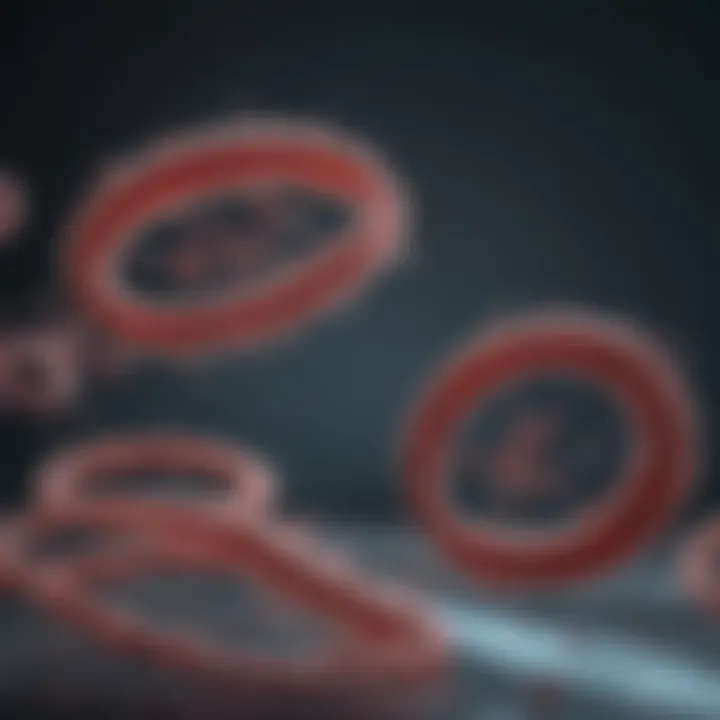
In addition to cloning vectors, transformation methods are crucial to introducing plasmids into E. coli. These methods can vary significantly in terms of efficiency and application, making it important to choose the right method for the specific goals of your research.
Cloning Vectors
Cloning vectors typically include plasmids, bacteriophages, or artificial chromosomes. A practical characteristic of plasmids is their ability to replicate autonomously within the host cell. Many plasmids also contain features such as antibiotic resistance markers, allowing for easy selection of successfully transformed cells. An example of a commonly used vector is the pUC plasmid family, which is favored for its high copy number and multiple cloning sites.
Transformation Methods
Transformation methods are techniques used to introduce foreign DNA into E. coli cells. These methods are essential for successful plasmid production and can be categorized based on the physical or chemical means employed. Here are the three prominent transformation methodologies:
Heat Shock Method
The heat shock method uses sudden temperature changes to facilitate the uptake of plasmid DNA by E. coli. Typically, cells are exposed to a brief heat shock, often around 42 °C, followed by a quick return to a lower temperature. This sudden change increases cell permeability, allowing plasmid DNA to enter. The heat shock method is popular because it is simple and does not require sophisticated equipment.
Key characteristic: The efficiency of this method can significantly depend on the strain of E. coli used and the quality of the plasmid DNA.
Advantages: High transformation rates can be achieved with low-cost materials.
Disadvantages: It may result in lower efficiency in terms of plasmid uptake, particularly with larger plasmids.
Electroporation
Electroporation involves applying an electrical field to create temporary pores in the cell membrane, allowing plasmid DNA to enter. This method is known for its versatility and can be used with various types of cells, including E. coli. The key characteristic of electroporation is its efficiency; it can achieve higher transformation rates compared to traditional methods.
Advantages: Electroporation can handle larger DNA molecules and provides high transformation efficiency.
Disadvantages: This method requires specialized equipment, which may not be accessible for all laboratories.
Microinjection
Microinjection is a technique where a fine needle is used to inject plasmid DNA directly into the cytoplasm of the E. coli cell. This method provides high precision and allows for controlled delivery of the plasmid. However, it is labor-intensive and requires specialized skills.
Advantages: Microinjection allows for precise control of the amount and timing of plasmid introduction.
Disadvantages: It is also time-consuming and not easily scalable for large numbers of cells.
The choice of transformation method is fundamental to the success of plasmid production, and each has its own set of benefits and limitations. Understanding these nuances enables researchers to optimize their methodologies for better plasmid yields.
Factors Affecting Plasmid Yield
The yield of plasmids from Escherichia coli can be influenced by multiple factors. Understanding these elements is necessary for optimizing the production of plasmids for research and practical applications. Whether it’s the selection of the strain or the culture conditions, each aspect plays a critical role in determining the efficiency of plasmid yields.
Selection of Strain
The choice of E. coli strain is paramount. Different strains exhibit varied capabilities in plasmid replication and stability. Some strains are specifically engineered to enhance plasmid yield, such as E. coli BL21, which is favored for its ability to efficiently produce recombinant proteins. Other strains, like E. coli Dα, are preferred for cloning purposes due to their competent transformation capabilities. It is essential to select a strain that aligns with the specific objectives of the plasmid production project.
Culture Conditions
Culture conditions greatly influence the overall health of E. coli and the plasmid yield.
Nutrient Medium
The nutrient medium provides the essential components for E. coli growth. Commonly used media include Luria-Bertani (LB) broth, which is rich in nutrients. The composition supports rapid cell division and allows plasmid replication. The specific aspect of LB medium is its high concentration of yeast extract, which contributes to the overall nutrient profile. However, it’s important that the medium is tailored to the plasmid's needs, as some plasmids may require additional supplements. The balance of nutrients directly impacts the efficiency and stability of plasmid production.
Temperature
Temperature is another critical factor influencing plasmid yield. Generally, culturing E. coli at 37 degrees Celsius is standard, as it promotes optimal growth. An important aspect is the need for temperature control during induction periods of protein expression. If temperatures are too high, it may lead to stress in the bacteria, which can reduce plasmid stability. Moreover, lower temperatures can sometimes favor the quality of protein expression while maintaining plasmid integrity, making temperature management essential for successful outcomes.
pH
The pH level of the culture medium must be carefully monitored. E. coli typically thrives in a neutral pH range of 6.5 to 7.5. Deviation from this range can cause growth inhibition or stress, ultimately affecting plasmid yield. High acidity or alkalinity can lead to denaturation of proteins, including those necessary for plasmid replication. Ensuring a stable pH environment contributes to maintaining cellular processes needed for efficient plasmid production.
Induction of Plasmid Expression
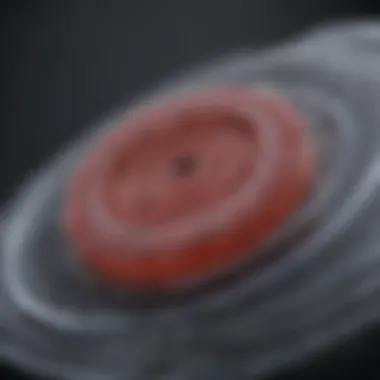
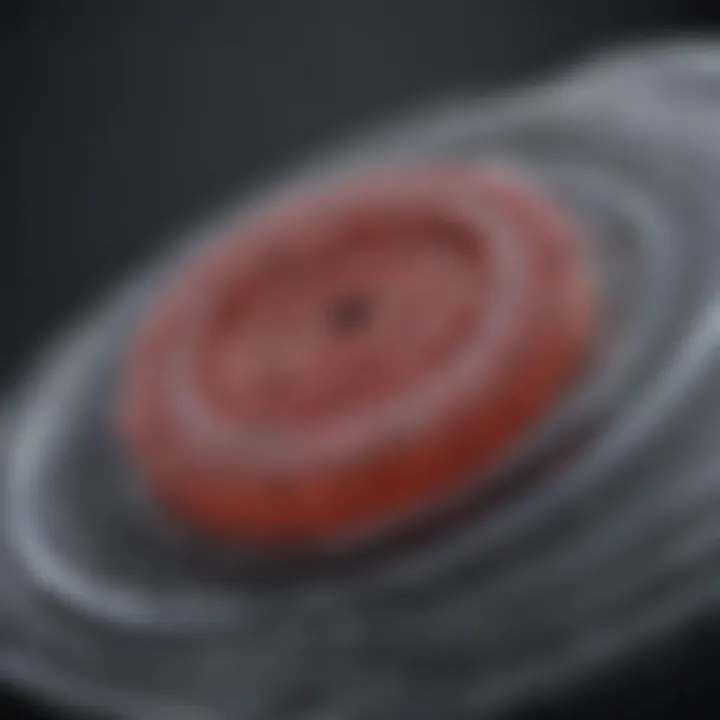
Induction methods are vital for expressing the plasmid once it has been introduced into the E. coli cells. The use of specific inducers, like IPTG (isopropyl β-D-1-thiogalactopyranoside), can activate the expression of plasmid-borne genes. The timing and concentration of the inducer are crucial to optimizing the yield of the desired product. Management of induction conditions can lead to higher protein expression levels, subtly impacting overall plasmid stability and yield.
Plasmid Purification Techniques
Plasmid purification is a critical step in the process of plasmid production. This ensures that the plasmids obtained are of high purity and suitable for various applications such as gene cloning, protein expression, and gene therapy. The methods utilized for purification can significantly influence the yield and quality of the plasmid DNA. Therefore, understanding the various purification techniques is essential for researchers working with plasmids.
Alkaline Lysis
Alkaline lysis is one of the most common and effective methods for isolating plasmid DNA from E. coli cells. The process involves several steps that help lyse the bacterial cells while keeping the plasmid DNA intact. Firstly, bacterial cells are resuspended in a buffer containing glucose and Tris, which protects the cells. Next, a lysis solution containing sodium hydroxide and detergent is added. This high-pH environment breaks down the cell membrane and denatures the chromosomal DNA, but plasmid DNA remains in a circular form.
After the lysis, neutralization occurs by adding an acidic solution. This neutralizes the pH, allowing for the renaturation of plasmid DNA. The resulting mixture is then centrifuged, separating the plasmid DNA from the cellular debris and chromosomal DNA, which precipitate out.
Researchers appreciate this method due to its simplicity and efficiency. However, it requires careful handling of solutions to prevent damage to the plasmid DNA.
Column Chromatography
Column chromatography is another prevalent method employed for the purification of plasmid DNA. This technique relies on the principles of adsorption and desorption. Following cell lysis and debris removal, the plasmid solution is applied to a chromatography column filled with a resin. The plasmid DNA binds to the resin while contaminants such as proteins and salts are washed away.
The bound plasmid can be eluted by adding an elution buffer that disrupts this binding. Column chromatography offers high purity and is often preferred when extreme purity is required.
There are various types of column chromatography, including ion exchange and size exclusion. Each type has specific applications based on the plasmid characteristics and the intended use.
Precipitation Methods
Precipitation methods involve the use of solvents to precipitate plasmid DNA from solution. This method often uses isopropanol or ethanol to selectively precipitate DNA. Before adding the alcohol, a salt such as sodium acetate may be introduced, which helps in the precipitation process by neutralizing the charge on the DNA.
After the alcohol is added, plasmid DNA precipitates out of the solution and can be collected by centrifugation. The pellet is then washed, dried, and dissolved in an appropriate buffer for downstream applications.
While these methods are generally quick and effective, they may sometimes co-precipitate contaminants, requiring additional steps for purification.
Plasmid purification techniques are fundamental for those involved in molecular biology, ensuring that high-quality plasmid DNA is available for further experimentation.
In summary, the choice of purification method depends on the specific requirements of the downstream applications and the nature of the plasmid being studied. Each technique has its advantages and considerations, making it essential for researchers to assess their needs carefully.
Applications of Plasmids
Plasmids serve as essential tools in molecular biology and biotechnology. Their versatile applications have transformed research and industry practices. This section highlights the significance of plasmid utilization, particularly focusing on gene cloning, protein expression, and gene therapy. Understanding these applications encourages innovation and enhances practical outcomes in scientific experiments and therapeutic developments.
Gene Cloning
Gene cloning is one of the primary uses of plasmids. This method allows scientists to create copies of specific genes. By inserting a gene of interest into a plasmid, researchers can replicate that gene within a bacterial host, such as E. coli. The process involves several steps, including selection of an appropriate plasmid, insertion of the target gene, and transformation into a host organism. Utilizing plasmids in gene cloning provides several benefits:
- Amplification: Plasmids enable the quick and efficient cloning of genes, leading to a large number of identical copies for study.
- Versatility: Different plasmids can be engineered for various applications, ranging from sequencing to functional studies.
- Tool for Manipulation: Incorporating foreign DNA into plasmids allows for further genetic manipulation, which is crucial for advanced research.
Overall, gene cloning using plasmids is foundational in fields like genetic research, synthetic biology, and personalized medicine.
Protein Expression
The production of proteins in a controlled manner is another critical application of plasmids. Protein expression systems, leveraging plasmids, enable the translation of genes into functional proteins. Scientists can use plasmid vectors designed to express proteins effectively in bacterial cells. Key aspects include:
- High Yields: E. coli can produce large quantities of proteins when paired with suitable plasmid vectors, allowing for commercial production of enzymes and therapeutic proteins.
- Post-translational Modifications: Although E. coli lacks the ability to perform complex modifications, specific strategies can be employed to achieve desired protein characteristics.
- Enzyme Production: Many industries rely on bacterial protein production, such as pharmaceutical and food sectors, where enzymes play a crucial role in processing and manufacturing.
The power of plasmids in protein expression contributes significantly to research, enabling studies on protein function, interactions, and structures.
Gene Therapy
Gene therapy is an innovative field that utilizes plasmids to deliver therapeutic genes into a patient's cells. The idea is to correct genetic defects or combat diseases by introducing functional genes. Plasmid-based systems offer several advantages in gene therapy applications:
- Safety: Plasmids are non-viral, reducing the risk of adverse effects related to viral vectors.
- Multi-gene Therapy: Advanced plasmids can carry multiple genes, allowing for combination therapies targeting various pathways in diseases.
- Long-lasting Effects: Properly designed plasmids can result in sustained expression of therapeutic genes in target tissues.
With the rise of gene therapy as a viable treatment for genetic disorders, the role of plasmids becomes even more pivotal in therapeutic development.
Challenges in Plasmid Production
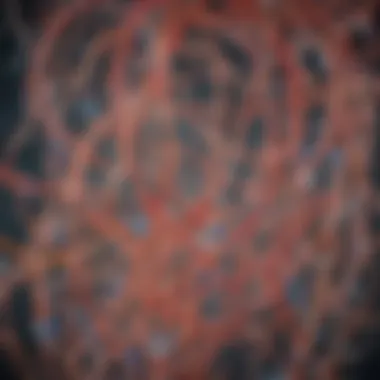
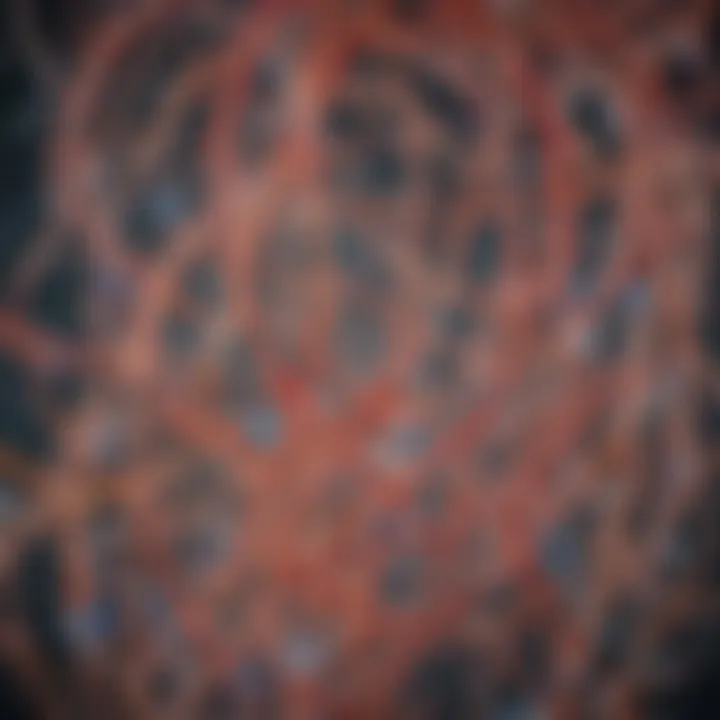
Understanding the challenges in plasmid production is essential for optimizing methodologies and achieving effective results in biotechnology. Plasmid production is not without its hurdles. These challenges can influence both the yield and quality of the plasmids produced. Addressing these issues is crucial for fields such as molecular biology research, gene therapy, and protein production.
Incompatibility Issues
Incompatibility issues refer to the problems that arise when different plasmids compete for limited resources within a host organism, such as E. coli. When multiple plasmids are introduced into the same bacterial strain, they can interfere with each other's replication. This competition can lead to lower yields of the desired plasmid.
To mitigate incompatibility issues, researchers often utilize specific plasmid combinations that are known to coexist without competition. Strategies like using different replication origins or selectable markers can also be effective.
Some key considerations to address incompatibility include:
- Selection of appropriate plasmids: Choose plasmids that are compatible based on their compatibility groups.
- Optimizing transformation protocols: Adjustments during the transformation process can enhance the stability of plasmid retention in bacterial strains.
- Monitoring plasmid ratios: Keep track of the ratios of different plasmids introduced to the E. coli to minimize competition.
Plasmid Stability
Plasmid stability is another significant challenge in plasmid production. Stability refers to the ability of the plasmid to be maintained within the bacterial host over time without loss or degradation.
Several factors can affect plasmid stability:
- Environmental conditions: Fluctuations in temperature, pH, and nutrient availability can impact plasmid maintenance.
- Host strain selection: Some E. coli strains are more prone to plasmid loss than others. Choosing stable strains is essential.
- Plasmid design: Features such as high copy number origins or strong promoters can affect stability positively or negatively.
To enhance plasmid stability, researchers often use:
- Stabilizing agents: Such as antibiotics that ensure continued selection for plasmid-bearing cells.
- Optimization of growth conditions: Maintain optimal culture conditions to promote the health and stability of the host cells.
Maintaining plasmid stability is vital for ensuring that the desired genetic material can be expressed consistently, which is crucial for most applications in research and industry.
Overall, addressing incompatibility issues and enhancing plasmid stability are critical for successful plasmid production. Advances in methods and practices continue to evolve, tailoring solutions to overcome these challenges effectively.
Recent Advancements in Plasmid Technology
Recent developments in plasmid technology have played a pivotal role in the fields of genetics and biotechnology. These advancements enhance the efficiency of plasmid production, improving its applications in gene therapy, vaccines, and synthetic biology. Innovations in synthetic plasmids and CRISPR technology are particularly notable, as they offer new opportunities to manipulate genetic material in precise ways.
Synthetic Plasmids
Synthetic plasmids represent a significant leap forward in plasmid technology. These are man-made plasmids designed for specific functions, tailored to suit various research needs. They can harbor custom-designed genes, making it easier to explore gene functions, protein interactions, and pathways.
Key benefits of synthetic plasmids include:
- Precision: Being engineered allows for accurate adjustments to enhance functionality.
- Versatility: They can be used across different organisms, facilitating comparative studies.
- Efficiency: Optimized for easy transformation into host cells like E. coli, resulting in higher yields of plasmid DNA.
Moreover, synthetic plasmids enable researchers to incorporate novel regulatory elements, improving gene expression control. This is particularly useful in the production of therapeutic proteins and enzymes.
CRISPR Technology
CRISPR technology has revolutionized genetic engineering by providing a precise tool for editing genes. This technology employs a guide RNA to direct the Cas9 enzyme to specific locations in the DNA for modification. The implications for plasmid production are extensive.
In relation to plasmids, CRISPR facilitates:
- Gene Editing: Quickly modifying plasmids to study gene function.
- Improved Targeting: Enhancing plasmid design to ensure that they integrate into the genome at desired locations.
- Rapid Development: Expedites the creation of genetically modified organisms for research or therapeutic use.
Utilizing CRISPR with plasmid technology allows for more streamlined processes in research, reducing time and resources spent on genetic manipulation.
"Recent advancements in synthetic plasmids and CRISPR technology highlight the accelerating pace of genetic research and its applications."
Finale
The conclusion of this article underscores the significance of understanding plasmid production in Escherichia coli. This topic is pivotal in molecular biology and biotechnology, with implications that stretch across various research fields. A thorough comprehension of methodologies, influencing factors, and the applications of plasmids allows researchers to optimize their experiments and enhance the efficacy of their work.
Summary of Key Points
Several key points emerge through the discussion of plasmid production:
- Importance of E. coli: As a model organism, E. coli offers unique advantages such as rapid growth, well-characterized genetics, and ease of manipulation, making it a preferred host for plasmid production.
- Methodologies Discussed: Various genetic engineering techniques, including cloning vectors and transformation methods, are crucial for introducing plasmids into E. coli. Each method presents distinct advantages and challenges.
- Factors Influencing Yields: The selection of E. coli strains and optimization of culture conditions significantly affect plasmid yields. Nutrient media, temperature, and pH are critical factors that require careful control.
- Plasmid Applications: The practical applications of plasmids in gene cloning, protein expression, and even gene therapy highlight their importance in advancing biological research and therapeutic development.
Future Directions in Research
Research in the field of plasmid production in E. coli is ever-evolving. Future directions may include:
- Development of Synthetic Plasmids: Innovations in synthetic biology could lead to the design of more efficient plasmids that optimize expression or stability, tailored for specific applications.
- Integration of CRISPR Technology: The advent of CRISPR technology may revolutionize plasmid production, offering precise editing capabilities to improve plasmids for gene therapy or biotechnology.
- Exploration of Alternative Hosts: While E. coli is the standard, exploring other microbial hosts could uncover new pathways for plasmid production that are even more beneficial for specific applications.
In summary, the ongoing exploration and innovation within plasmid production not only enhance our fundamental understanding of molecular biology but also open a myriad of opportunities for practical applications in medicine, agriculture, and beyond.