RNA Extraction from Muscle Tissue: Techniques and Applications
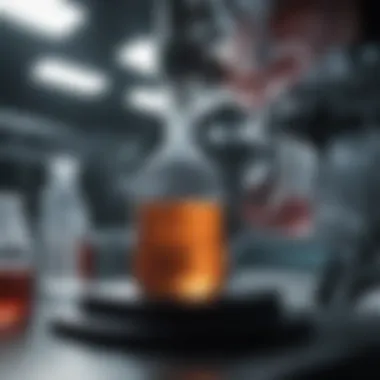
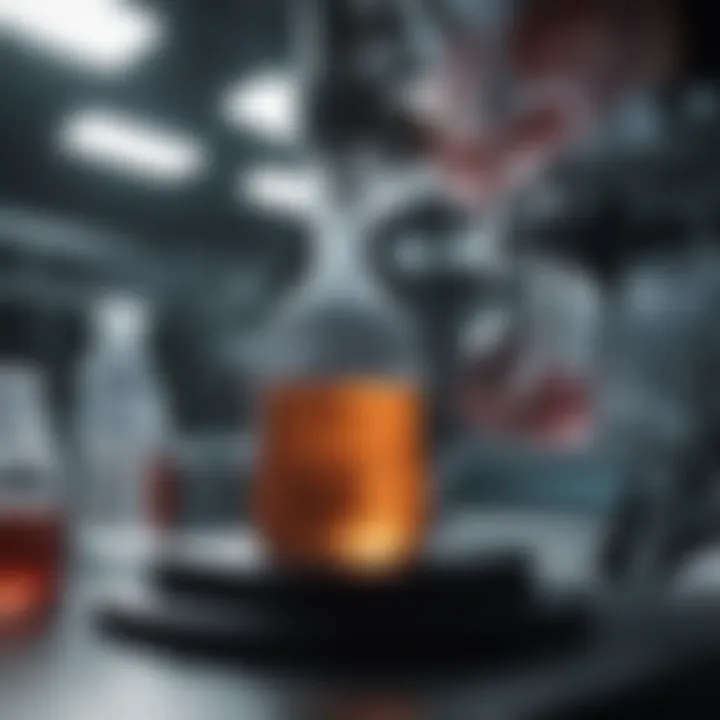
Intro
RNA extraction from muscle tissue is a critical procedure within the field of molecular biology. It plays a significant role in understanding gene expression, muscle physiology, and various diseases associated with muscle dysfunction. The complexities involved in extracting high-quality RNA from muscle tissue require meticulous attention to detail.
Proper extraction techniques can yield RNA that is suitable for various applications in research. These applications range from studying cellular responses to different stimuli to investigating the effects of genetic modifications on muscle development and repair. This article provides a comprehensive overview of the methodologies employed in RNA extraction, the challenges faced, and the implications of the findings.
Key Findings
Summary of the Main Results
Research reveals that optimal RNA extraction from muscle tissue hinges on several factors. These include the selection of appropriate extraction kits and keeping samples at appropriate temperatures to avoid RNA degradation. Recent advancements show that using specialized reagents can enhance yield and purity.
Furthermore, muscle tissue is known for its high content of proteins and secondary metabolites, which complicates the extraction of RNA. Techniques such as homogenization and the use of lysis buffers are essential for breaking down cellular structures. An important finding is that the quality of extracted RNA can directly influence downstream applications such as quantitative PCR or RNA sequencing.
Significance of Findings Within the Scientific Community
The findings from this area of research have broad implications. High-quality RNA extraction techniques facilitate the study of muscle-related diseases, such as muscular dystrophy and age-related atrophy. The community benefits from improved methodologies that allow for reproducible and reliable results, fostering the advancement of molecular biology as a discipline.
Implications of the Research
Applications of Findings in Real-World Scenarios
The applications of effective RNA extraction techniques are far-reaching. In clinical settings, accurate analysis of gene expression informs treatment protocols for muscle wasting conditions. In research laboratories, scientists utilize extracted RNA to investigate the effects of various compounds on muscle tissue, contributing to the development of new therapies.
Potential Impact on Future Research Directions
As RNA extraction methods continue to evolve, future research may focus on further refining these techniques. The integration of automated systems may enhance efficiency and consistency. Additionally, continuous improvement in understanding the characteristics of muscle tissue could lead to innovative strategies for RNA extraction. This could pave the way for breakthroughs in regenerative medicine and biotechnology.
"Understanding the nuances of RNA extraction from muscle tissue propels forward our capacity to understand complex biological systems and their responses to various stimuli."
By examining the methodologies and implications of RNA extraction, we can appreciate its importance in advancing scientific knowledge and addressing pressing health issues. This article aims to provide a thorough understanding of the topic, ensuring the information is valuable for students, educators, and professionals in the field.
Foreword to RNA and Its Role in Muscle Tissue
RNA (ribonucleic acid) is a crucial molecule in the biological processes of all living organisms. Understanding its role in muscle tissue helps elucidate various physiological functions and mechanisms. This section establishes a foundational grasp of RNA, which is essential for the subsequent discussions on RNA extraction methodologies.
Understanding RNA Structure
RNA, like DNA, is made up of nucleotides, but it has several distinct features. Each nucleotide consists of a ribose sugar, a phosphate group, and a nitrogenous base. The nitrogenous bases in RNA are adenine (A), uracil (U), cytosine (C), and guanine (G). The presence of uracil instead of thymine, which is found in DNA, is one of the key differences.
The structure of RNA can vary; it exists primarily as a single strand. However, it can fold back on itself to form various secondary structures. This capability is vital for its function in protein synthesis and gene regulation, especially in muscle cells where precise control over muscle contraction and metabolism is necessary.
Functions of RNA in Muscle Cells
RNA performs several vital roles within muscle cells, particularly in gene expression and translation. Messenger RNA (mRNA) carries genetic information from DNA in the nucleus to the ribosomes, where proteins are synthesized. In muscle cells, the proteins encoded by these mRNAs are critical for muscle contraction, repair, and growth.
Besides mRNA, two other types of RNA are significant. Transfer RNA (tRNA) is responsible for bringing amino acids to the ribosome, effectively translating the mRNA's instructions into functional proteins. Ribosomal RNA (rRNA) forms part of the structure of ribosomes and plays a crucial role in the synthesis process.
The dynamic nature of RNA in muscle cells ensures they can respond rapidly to changes in physiological conditions, such as exercise or injury. Therefore, studying RNA in this context not only provides insights into muscle biology but also lays the groundwork for understanding related diseases and conditions.
Significance of RNA Extraction from Muscle Tissue
RNA extraction from muscle tissue is pivotal in understanding a wide array of biological functions and mechanisms. Muscle tissues provide a complex environment that reflects physiological states, making RNA studies particularly valuable. The process of extracting RNA holds several significant implications, chiefly within gene expression studies and muscle physiology research, which will be explored in detail in the following subsections.
Impact on Gene Expression Studies
Gene expression analysis relies on high-quality RNA to provide insights into cellular functions and regulations. In muscle cells, RNA extraction enables researchers to investigate the transcripts produced, which are crucial for muscle development, repair, and adaptation.
Studies have shown that various factors such as exercise, diet, and disease impact gene expression in muscle tissue. By examining the RNA, researchers can pinpoint specific genes that are upregulated or downregulated under different conditions. This valuable information contributes to our understanding of how muscles respond to external stimuli and internal changes.
Moreover, extracted RNA allows for the application of advanced techniques such as quantitative PCR and RNA sequencing. These methods can lend a quantitative and qualitative perspective on gene expression profiles. Importantly, accurate RNA extraction minimizes variability—ensuring that results reflect true biological changes rather than extraction artifacts.
Another critical aspect is the ability to compare RNA from different muscle types or states. For example, contrasting the RNA profiles from skeletal muscle in athletes versus sedentary individuals can unveil adaptations attributable to training.
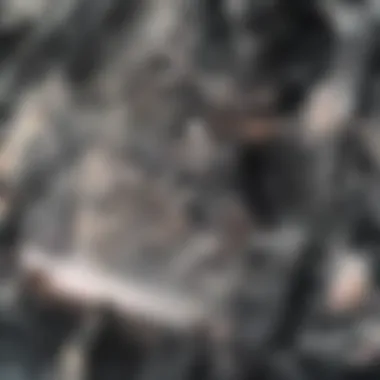
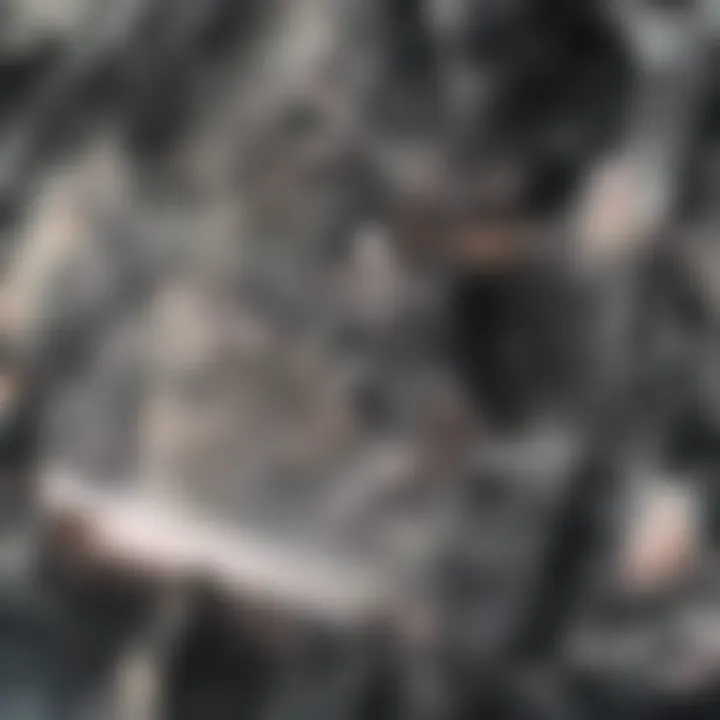
Contribution to Muscle Physiology Research
Understanding muscle physiology is essential for developing strategies to enhance performance, prevent injuries, and treat muscle-associated conditions. RNA extraction from muscle tissue plays an integral role in this domain by providing a detailed view of underlying molecular processes.
The information obtained from RNA studies helps scientists comprehend mechanisms involved in muscle contraction, metabolism, and recovery. For example, alterations in the expression of myogenic regulatory factors are crucial for muscle growth and repair. By analyzing RNA, researchers can elucidate how various signaling pathways influence muscle adaptation.
Furthermore, RNA extraction aids in studying muscle wasting conditions such as cachexia and sarcopenia. Research focused on the RNA profiles in affected muscle tissues can identify potential biomarkers or therapeutic targets. Understanding the transcriptomic changes in diseased states helps in formulating clinical approaches to manage these conditions effectively.
In summary, the significance of RNA extraction from muscle tissue extends beyond a mere laboratory technique. It is a powerful tool that enhances the collective understanding of muscle biology and provides the foundation for innovative research directed toward improving human health.
Overview of RNA Extraction Techniques
The extraction of RNA from muscle tissue is a fundamental process in molecular biology that serves various research needs. Understanding the techniques available is essential for any scientist aiming to study gene expression, investigate muscle physiology, or develop therapeutic strategies. An overview of RNA extraction techniques sheds light on historical and modern methods, offering insights into their advantages, limitations, and specific applications. Not only does this knowledge foster an appreciation for the technology, but it also emphasizes the necessity of selecting appropriate methods based on the study's goals.
Classical Methods of RNA Extraction
Classical methods for RNA extraction include techniques like the phenol-chloroform extraction and guanidine isothiocyanate method. These methods have been widely used for decades and laid the foundation for subsequent extraction protocols. Phenol-chloroform extraction involves using organic solvents to separate RNA from proteins and DNA. The process includes steps of homogenization, centrifugation, and precipitation.
This method benefits from its effectiveness in yielding high-quality RNA. However, it also presents some drawbacks. The use of hazardous chemicals poses safety concerns, necessitating precautions like using fume hoods and personal protective equipment. Furthermore, these methods can be labor-intensive, requiring significant amounts of time and skill.
Key aspects of classical methods include:
- High yield: Classical methods often produce a high concentration of RNA suitable for downstream applications.
- Quality control: The simplicity of the procedure allows for easier troubleshooting and optimization.
- Chemical exposure risk: The use of organic solvents requires caution to avoid potential harm.
- Time-consuming: Protocols can take longer than modern automated methods.
Modern Automated Techniques
With advances in biotechnology, automated RNA extraction techniques have emerged. Companies like Qiagen and Thermo Fisher Scientific offer kits that simplify the extraction process. These automated systems can significantly reduce labor time while improving reproducibility.
Modern techniques often utilize silica membrane-based or magnetic bead-based methods. These extraction kits provide a streamlined process where the sample is lysed, and RNA is selectively captured while contaminants are washed away. Automation in RNA extraction addresses many drawbacks associated with classical methods.
Some advantages of modern techniques include:
- Speed: Automated systems reduce hands-on time and provide quicker results.
- Consistency: Automation decreases variability between dissections, improving the reliability of results.
- Safety: Reduced chemical handling lowers the risk of exposure.
- Scalability: Many systems allow for parallel processing of multiple samples.
In summary, both classical and modern techniques have their places in RNA extraction from muscle tissue. The choice between methods should be based on experimental design, sample types, and specific research needs. By understanding these approaches, researchers can ensure accurate and reproducible results that advance the field of molecular biology.
Pre-Extraction Considerations
The section on pre-extraction considerations is crucial for understanding the foundations of effective RNA extraction from muscle tissue. Proper planning and initial steps can make a significant difference in the subsequent RNA quality and yield. By giving attention to specific elements prior to extraction, researchers can enhance the reliability and reproducibility of their results.
The first step involves the selection of muscle tissue samples. Different types of muscle tissues may yield varying amounts of RNA and can affect the quality of the extracted material. Factors such as age, health status, and physiological conditions of the source organism should also be taken into account. For example, muscle from a healthy specimen may provide superior RNA than that from a diseased one. Moreover, the type of muscle, whether it is skeletal or cardiac, can also present different challenges in extraction.
Additionally, researchers must acquire samples promptly after dissection and employ proper storage techniques to prevent degradation. This urgency is critical because RNA is unstable and sensitive to environmental conditions. Immediate flash freezing in liquid nitrogen is a commonly recommended practice, which preserves the integrity of the RNA until extraction can occur.
Here are some considerations for sample selection:
- Ensuring uniformity in sample size to achieve consistent results.
- Evaluating muscle condition and health status for optimal yield.
- Maintaining a cold chain to minimize RNA degradation.
Selection of Muscle Tissue Samples
The selection process for muscle tissue samples needs careful evaluation because it lays the groundwork for successful RNA extraction. It is advisable to choose samples that are freshly harvested, ideally within a few hours post-mortem. If immediate extraction cannot be performed, appropriate storage conditions must be established.
Various muscle tissues—including skeletal, cardiac, and smooth muscle—have distinct characteristics impacting RNA extraction efficiency. For instance, skeletal muscle often provides higher yields of RNA compared to cardiac. Furthermore, the choice of muscle tissue should align with the specific biological question being researched. Researchers should justify their choice by considering the biological relevance and RNA quality expected from that tissue type.
Importance of Sample Protocols
Sample protocols are essential for ensuring the integrity of RNA during the extraction process. They dictate how samples should be handled, processed, and stored prior to extraction. Following standardized protocols prevents contamination and degradation, which are frequent issues that can adversely affect results.
Researchers may develop a specific protocol based on their laboratory's unique needs, but adherence to established guidelines is recommended. This includes procedures for:
- Collecting and storing samples using sterile techniques.
- Implementing appropriate buffer solutions that stabilize RNA.
- Recording all handling and storage details during the process.
A well-documented sample protocol ensures reproducibility of results and facilitates comparison with other studies. Thus, the importance of protocols cannot be overstated, as they serve as the backbone for successful and repeatable RNA extractions in muscle tissue research.
In summary, the pre-extraction phase sets the stage for effective RNA extraction. Thoughtful choices about tissue selection and adherence to sample protocols significantly influence the quality of RNA obtained, impacting downstream analyses.
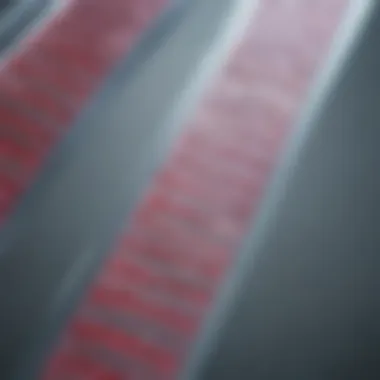
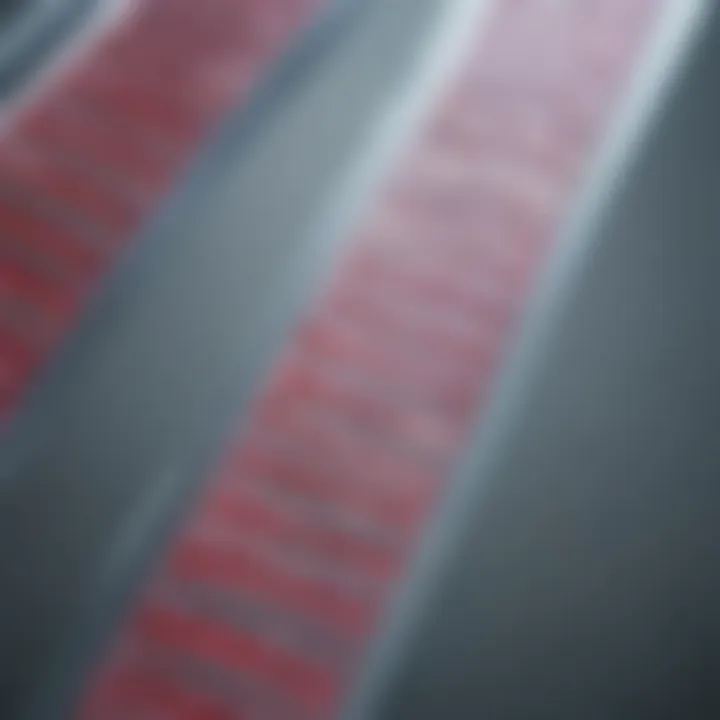
Reagent Selection and Preparation
Reagent selection and preparation are critical steps in the RNA extraction process from muscle tissue. The choice of reagents directly influences the yield and quality of the extracted RNA. This process is not merely mechanical; it requires an understanding of each reagent's role, preparation guidelines, and implications for downstream applications.
The importance of proper reagent selection cannot be overstated. If the wrong reagents are used, it can lead to low RNA yields, compromised integrity, and contamination with genomic DNA or proteins. Thus, choosing effective and reliable reagents is essential for achieving high-quality results in RNA extraction.
Common Reagents Used in RNA Extraction
Several reagents are typically utilized in RNA extraction protocols. These include:
- Lysis Buffer: This is essential for breaking down cell membranes and releasing RNA into solution. It often contains detergents such as SDS or Triton X-100, which facilitate cell lysis.
- Phenol-Chloroform: A classic choice for phase separation. It helps separate RNA from proteins and DNA due to its immiscibility with water, providing a clean extraction.
- Ethanol or Isopropanol: Used for RNA precipitation. These alcohols help in the selective precipitation of RNA after the phase separation step.
- RNase Inhibitors: Added to protect RNA from degradation during extraction. These agents, such as RNasin, prevent the action of ribonucleases.
- Buffer Solutions: Solutions, like Tris or EDTA, are often included to maintain a stable pH and chelate divalent ions, which can be harmful to RNA integrity.
The purity and effectiveness of these reagents have a significant impact on the resulting RNA quality.
Role of Enzymatic and Chemical Agents
In addition to the basal reagents, enzymatic and chemical agents play an important role in enhancing the efficiency of RNA extraction.
- Proteinase K: This enzyme is commonly used to digest proteins in the sample, ensuring that RNA is not co-purified with contaminants. Its inclusion is especially useful in tissues where proteins are abundant.
- Guanidium thiocyanate: This is a strong denaturant that disrupts protein interactions, facilitating RNA isolation. It helps maintain RNA integrity by inactivating ribonucleases that could degrade RNA during extraction.
- Sodium Dodecyl Sulfate (SDS): Utilized primarily as a detergent, SDS aids in lysing cells effectively. It disrupts protein structures and helps solubilize RNA, making it more accessible for extraction.
Each of these agents must be prepared with precision as even slight deviations in concentration can affect the outcome. Adhering to the manufacturer's instructions and optimizing conditions may be necessary, particularly when dealing with different types of muscle tissue.
The correct choice and preparation of reagents are the foundation upon which successful RNA extraction is built. Without adequate understanding and attention to detail, the integrity of extracted RNA may be compromised, affecting further analyses.
Step-by-Step RNA Extraction Procedure
The step-by-step RNA extraction procedure serves as a cornerstone in understanding how to derive high-quality RNA from muscle tissue. This procedure not only addresses the practical execution of RNA extraction but also highlights the importance of precision and care throughout each stage. The integrity of the extracted RNA is crucial for subsequent research applications, particularly in gene expression analysis and physiological studies. Proper execution of each procedural step is essential; small errors can lead to compromised RNA quality or yield, which can, in turn, affect the reliability of future research.
Homogenization of Muscle Tissue
Homogenization is the initial step in the RNA extraction process where muscle tissue is disrupted to release RNA from cells. The effectiveness of this step is critical; complete homogenization ensures that cells are fully lysed, facilitating the release of RNA into the extraction buffer. This process can be performed using various devices such as tissue grinders or bead mills. The choice of method may depend on the specific muscle tissue type being processed.
It is also important to consider the conditions under which homogenization occurs. The use of cold conditions can help mitigate RNA degradation caused by RNases. Additionally, care should be taken to add a suitable lysis buffer immediately after homogenization to shield the RNA from enzymatic digestion. Maintaining an RNase-free environment throughout this step is paramount for achieving optimal RNA yield.
Phase Separation Techniques
After homogenization, phase separation techniques are employed to isolate RNA from other cellular components, such as proteins and DNA. Typically, this step involves using organic solvents like phenol and chloroform. When mixed with the homogenate, these solvents create distinct phases: the upper aqueous phase, where RNA is found, and the lower organic phase containing proteins and lipids.
The key to successful phase separation lies in ensuring sufficient agitation during this mixing process, followed by centrifugation. Centrifugation allows for the separation of the phases based on density, leading to the desired isolation of RNA in the upper phase. Care should be exercised during the transfer of the upper phase to avoid contamination with the lower phase, which would adversely affect RNA purity.
Precipitation and Washing Steps
Following phase separation, the extracted RNA needs to be precipitated and washed. This step typically involves the addition of alcohol, such as isopropanol or ethanol, to the aqueous phase containing RNA. Alcohol precipitates RNA from the solution, allowing it to be collected by centrifugation.
Washing steps follow precipitation to remove any remaining contaminants. The precipitated RNA can be washed with ethanol, which helps eliminate salts and other impurities. It is important to perform this washing step gently to avoid losing RNA. After washing, the RNA pellet is often dried briefly to remove excess alcohol before being resuspended in RNase-free water or buffer. Each of these steps contributes to the overall quality and integrity of the extracted RNA, preparing it for quantification and analysis.
Proper execution of the RNA extraction procedure is critical. Any errors can lead to low yields or degraded RNA, ultimately impacting research outcomes.
In summary, a thorough understanding of these steps, including homogenization, phase separation, and the final precipitation and washing, equips researchers with the necessary skills to reliably extract RNA from muscle tissues. This foundational knowledge is essential for those engaged in molecular biology and related fields.
Post-Extraction RNA Quality Assessment
Evaluating the quality of RNA following extraction is critical in ensuring the reliability and validity of downstream applications. RNA quality assessment helps researchers to confirm that the extracted RNA is suitable for various analyses, including gene expression studies and functional assays. Poor-quality RNA can lead to inconclusive or misleading results, rendering the research efforts ineffective.
Measuring RNA Concentration
Measuring RNA concentration is a crucial first step in RNA quality assessment. It allows researchers to determine how much RNA is available for further experimentation. Common methods for measuring RNA concentration include spectrophotometry and fluorometry. Spectrophotometers can measure absorbance at 260 nm and 280 nm wavelengths, which helps to assess RNA concentration and purity, respectively. An A260/A280 ratio of ~2.0 indicates pure RNA.
Fluorometric methods, on the other hand, employ fluorescent dyes that specifically bind to RNA. This method is often considered more sensitive and accurate, especially for low concentrations of RNA. Knowing the RNA concentration is essential, as it guides subsequent dilution and experimental design, ensuring that RNA is not under or over-utilized in assays.
Assessing RNA Integrity
Assessing the integrity of RNA is equally important as measuring its concentration. RNA integrity indicates whether or not the RNA is intact and functional for further applications. Degraded RNA can produce misleading results in experiments. The most widely used method for assessing RNA integrity is the Agilent Bioanalyzer, which performs an electrophoresis-based analysis, providing a RNA Integrity Number (RIN) score. A RIN score closer to 10 indicates intact RNA, while scores below 5 suggest significant degradation.
Visual inspection of RNA quality via gel electrophoresis is another method for assessing RNA integrity. In this method, RNA samples are loaded onto an agarose gel and subjected to an electric field. The resulting bands reveal the purity and state of the RNA. The presence of sharp, distinct bands corresponding to ribosomal RNA (rRNA) indicates good integrity, while smeared or faint bands suggest degradation.
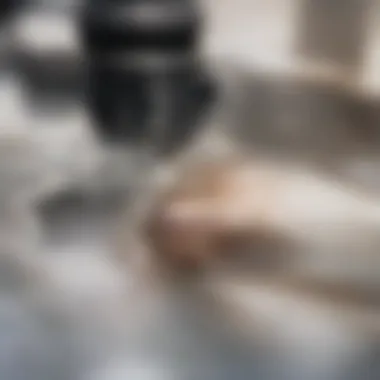
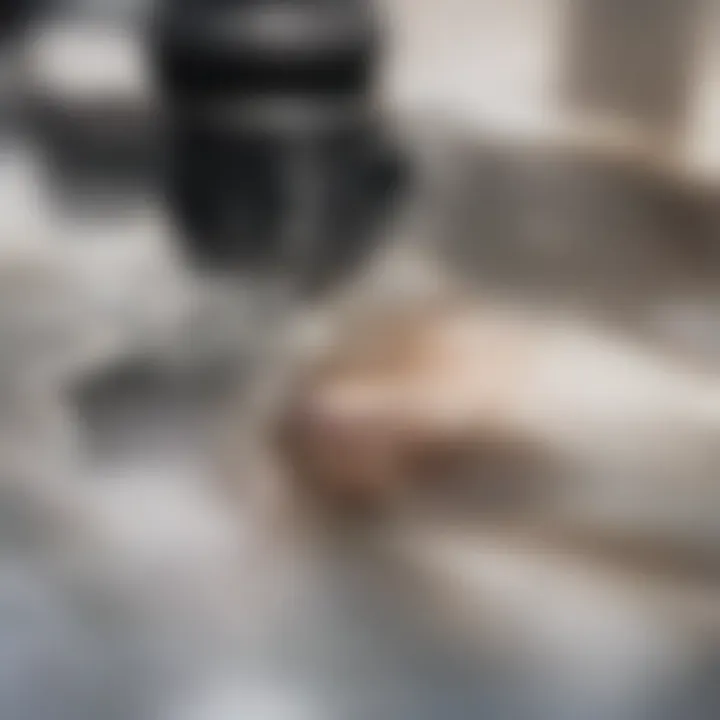
Ensuring high RNA quality is not only about having enough quantity; the integrity of the RNA is pivotal in ensuring reliable results.
Maintaining best practices during extraction and handling can minimize RNA degradation risks. This includes using RNase-free reagents and materials, as well as working quickly to limit exposure to ambient conditions. By adhering to these protocols, researchers can obtain high-quality RNA that is suitable for a range of molecular biology applications.
Troubleshooting Common Issues
Troubleshooting common issues during RNA extraction is crucial for ensuring high-quality results. Problems such as low RNA yield and RNA degradation can significantly affect the integrity of the extracted nucleic acids. Understanding how to identify and rectify these issues not only enhances the efficiency of the extraction process but also ensures that the subsequent analyses yield meaningful data. This section discusses key challenges and potential solutions, making it essential reading for both novice and experienced researchers alike.
Low RNA Yield
Low RNA yield can be a frustrating outcome when extracting RNA from muscle tissue. There are various factors that contribute to this issue. First, the selection and quality of the muscle tissue sample play a significant role in the yield. Samples that are not fresh or that have undergone freeze-thaw cycles are more likely to provide low RNA concentrations. Additionally, improper homogenization may result in poor lysis of the tissue, leading to inadequate RNA extraction.
To mitigate this problem, researchers should ensure that high-quality, fresh samples are used. It is recommended to immediately snap-freeze muscle tissues in liquid nitrogen after dissection to preserve RNA integrity. Furthermore, employing rigorous homogenization techniques can improve the yield by breaking down cell structures more effectively.
Another aspect to consider involves the reagents used during the extraction process. The quality of reagents can also influence RNA yield. Using aged or improperly stored reagents can lead to inconsistent results. Therefore, using fresh reagents is recommended to make sure they perform optimally.
RNA Degradation
RNA degradation is a major concern in any RNA extraction protocol. The stability of RNA is compromised by ribonucleases, which are enzymes that break down RNA molecules. Even small amounts of these enzymes can lead to degradation, which can be disastrous for downstream applications such as qPCR or RNA sequencing.
To prevent RNA degradation, researchers must practice diligence in their extraction workflows. Utilizing RNase-free equipment and reagents is critical. Employing protective measures, such as working on ice and using gloves, can help to minimize the risk of contamination. Additionally, the immediate processing of muscle tissue samples can limit RNA degradation.
Another effective strategy is the use of RNase inhibitors during the extraction process. These inhibitors can help to preserve the integrity of the RNA by inhibiting the activity of ribonucleases. Proper storage conditions post-extraction are also vital; samples should be stored at -80°C to maintain RNA quality for future experiments.
Key Takeaway: Addressing low RNA yield and RNA degradation effectively requires a thorough understanding of the factors at play and the implementation of best practices in sample handling, reagent quality, and extraction techniques. This will ultimately result in high-quality RNA suitable for advanced research applications.
By recognizing and resolving these common issues, researchers can significantly improve the reliability of their results, leading to more conclusive findings in muscle biology and related fields.
Applications of Extracted RNA in Research
Extracted RNA serves a pivotal role in molecular biology, especially in muscle tissue research. The extraction process allows for the study of gene expression and muscle biology, providing insights that are critical for understanding various physiological mechanisms and pathological conditions. The relevance of RNA extraction spans several domains including genetics, clinical research, and therapeutic development.
Gene Expression Analysis
Gene expression analysis is one of the foremost applications of extracted RNA. This process involves measuring the RNA levels of specific genes to determine their activity within muscle tissue. Understanding gene expression is essential for deciphering the functional dynamics of muscle cells under various conditions such as exercise, disease, or aging.
Several techniques are employed in gene expression analysis, such as quantitative PCR (qPCR) and RNA sequencing (RNA-seq). These methods enable researchers to obtain quantitative data about gene expression levels, which can be related back to physiological conditions. For instance, elevated levels of certain mRNAs may indicate muscle hypertrophy or regeneration after injury.
Furthermore, gene expression profiles can reveal underlying mechanisms of muscle diseases, including muscular dystrophies and atrophy. The data obtained can inform therapeutic strategies, driving the development of targeted treatments. RNA from muscle tissue, therefore, contributes significantly to the understanding of both normal physiology and disease.
Functional Studies in Muscle Biology
Functional studies in muscle biology utilize extracted RNA to investigate the role of specific genes and pathways in muscle function and development. By analyzing the products of gene expression, particularly proteins, scientists can elucidate how various factors influence muscle performance, plasticity, and recovery.
In these studies, researchers might employ techniques like Western blotting or mass spectrometry to correlate RNA data with protein levels and activity. This integrated approach helps clarify the functional implications of gene expression changes in muscle tissue. For example, studies might focus on the effects of resistance training on muscle gene expression involved in metabolism or adaptation.
Additionally, understanding gene function through RNA studies often leads to innovative ideas for rehabilitation and performance enhancement. The importance of extracted RNA is compounded when developing interventions that can improve muscle health in both athletes and patients suffering from muscle-wasting conditions.
Extracted RNA is not just a by-product of tissue studies; it is a central component in uncovering the complex interactions that define muscle health and disease.
In summary, the applications of extracted RNA in research are multifaceted. Whether through gene expression analysis or functional studies, RNA extraction is indispensable in providing clarity and insight into muscle biology. This understanding can drive further advancements in treatments, therapies, and our comprehension of muscle function.
Future Directions in RNA Extraction Techniques
The continuous advancement in RNA extraction techniques is essential in enhancing molecular biology research. As methods become more refined, the ability to extract high-quality RNA efficiently will only improve. This section examines how technological improvements impact RNA extraction, emphasizing automation and standardization. It also highlights the significance of these advancements for research applications.
Advancements in Technology
Recent years have seen noteworthy advancements in RNA extraction technologies. Automation stands out as a major trend. The use of automated robotic systems minimizes human error and enhances reproducibility. Such systems can process multiple samples simultaneously, increasing throughput.
Novel extraction kits, such as the Qiagen RNeasy or Thermo Fisher's MAX kit, have introduced simpler protocols and users-friendly guidelines. These kits can yield higher-quality RNA with minimal impurities. Integration with microfluidics technology is also emerging. Microfluidic devices allow for rapid, micro-scale extraction. They conserve samples and reagents, which may be particularly beneficial when working with limited amounts of tissue.
Another key development is the use of alternative extraction methods. Techniques such as magnetic bead-based extraction and enzymatic methods provide improved results. For example, magnetic beads can be used for binding RNA selectively, reducing the risk of contamination.
Implications for Research Applications
The advancements in RNA extraction techniques hold great implications for various areas of research. Enhanced extraction methods lead to better quality and quantity of RNA. This is crucial for gene expression studies and allows for more accurate results. Improved RNA integrity ensures reliable downstream applications, such as qPCR, RNA sequencing, and microarray analysis.
In muscle biology research, these improvements support detailed investigations into muscle development and regeneration. Higher accuracy in RNA analysis may contribute to understanding muscle diseases. Furthermore, efficient extraction ensures that studies can be replicated, a critical criterion in scientific research.
"Advancements in RNA extraction methods are not merely technical improvements; they are essential tools for illuminating molecular mechanisms that underlie complex biological processes, particularly in specific tissues like muscle."