Perovskite Solar Cell Efficiency: Advances and Challenges
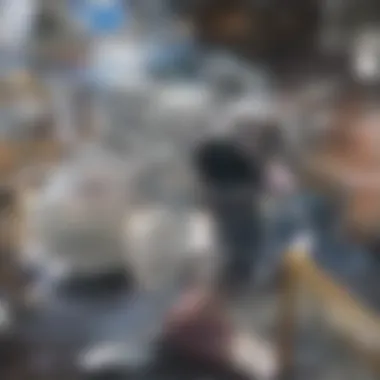
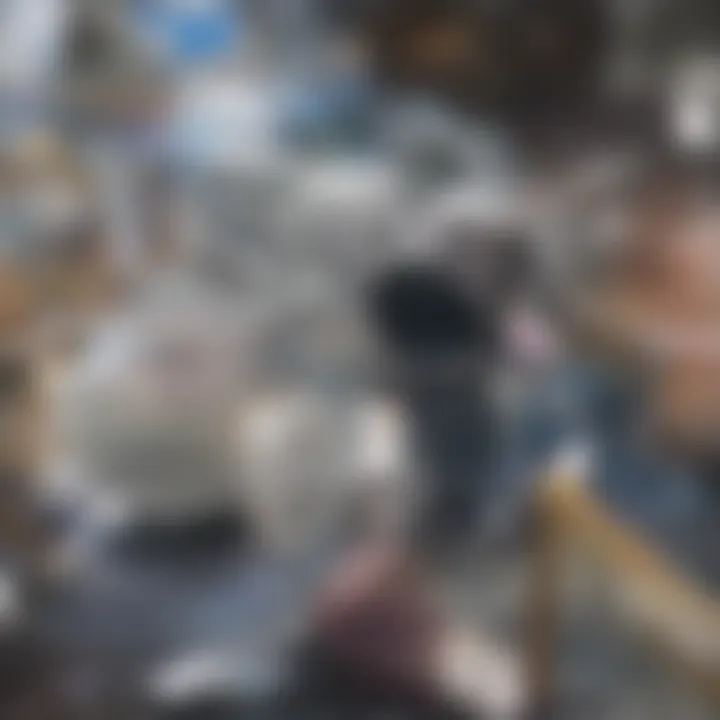
Intro
Perovskite solar cells have emerged as a prominent technology within the renewable energy sector. Their unique structure enables them to achieve high efficiency in converting sunlight into electricity, often outperforming traditional silicon-based solar cells. The composition and properties of perovskite materials allow for exceptional light absorption and charge mobility, making them a focal point of current solar energy research.
This article will navigate the complexities surrounding perovskite solar cells, examining recent innovations and persistent challenges. In particular, it will delve deep into the efficiency of these cells and the factors that can enhance or impede their performance. Attention will also be devoted to the viability of perovskite technology in real-world applications and its potential to transform the energy landscape. Understandably, with advancements come challenges, and this discussion will carefully consider the issues impacting stability and long-term usability within diverse environments.
Through a detailed exposition of fundamental concepts, significant discoveries, and future directions, this narrative aims to equip students, researchers, educators, and professionals with comprehensive insight into the current state and trajectory of perovskite solar cell technology.
Prelude to Perovskite Solar Cells
Perovskite solar cells have emerged as a significant development in renewable energy technology. Their ability to achieve high efficiency at relatively low production costs makes them a compelling option in the quest for more sustainable energy solutions. Understanding these cells requires grasping their composition, historical growth, and current integration into solar technology.
Definition and Composition
Perovskite solar cells derive their name from the mineral perovskite, which has a particular crystal structure. The general formula for perovskite materials is ABX3, where ‘A’ and ‘B’ are cations of different sizes, and ‘X’ is an anion. In solar cells, common materials include organic cations like methylammonium for ‘A’, metal cations such as lead or tin for ‘B’, and halogen elements for ‘X’. This composition allows for excellent light absorption and efficient charge transport. Perovskite structures also enable tunability, meaning that changing the materials can enhance performance by modifying the band gap.
Historical Development
The journey of perovskite solar cells began in 2009 when the first hybrid organic-inorganic perovskite solar cell was reported. These early prototypes showcased surprising efficiency gains, rapidly boosting interest in this technology. Within a few years, researchers had improved the efficiency rates significantly, reaching levels comparable to traditional silicon-based solar cells. Key milestones include the development of stable materials and improved fabrication techniques. The fast-paced growth of this field reflects a broader trend in solar technology, where innovation directly leads to increased performance.
Current Status in Solar Technology
Today, perovskite solar cells are no longer just a theoretical concept; they are recognized contenders in the solar energy market. Currently, lab-scale cells have reached over 25% efficiency in converting sunlight into electricity. The lightweight and flexible nature of perovskite materials opens novel applications, making them attractive for integration into various surfaces, including building materials and consumer electronics. However, despite these successes, commercial viability still faces challenges, particularly regarding stability and long-term performance. The intersection of academic research with industry continues to evolve as both sectors aim for practical deployment of perovskite technologies.
Understanding Efficiency Measurements
Understanding efficiency measurements in perovskite solar cells is crucial for both researchers and practitioners in the solar industry. Efficiency metrics provide insights into how effectively solar cells convert sunlight into electrical energy. This section highlights the importance of investigating efficiency measurements, their implications for research advancements, and their relevance in commercial applications.
Theoretical Maximum Efficiency
The theoretical maximum efficiency of a solar cell refers to the highest possible efficiency that can be theoretically achieved under ideal conditions. For perovskite solar cells, this efficiency is dictated by fundamental physical laws. The Shockley-Queisser limit indicates that for a single-junction solar cell, the maximum efficiency is approximately 33.7% when absorbing sunlight across the entire spectrum.
While perovskite materials have shown promise in approaching these theoretical limits, practical constraints continue to pose challenges. Factors such as light trapping, carrier collection, and optical losses play significant roles. Improvements in material composition and cell structure aim to push efficiencies closer to this theoretical maximum, yet they require extensive experimental validation.
Common Efficiency Metrics
Several metrics are essential for evaluating the efficiency of perovskite solar cells. The most common ones include:
- Power Conversion Efficiency (PCE): This is the primary measure of a solar cell's efficiency, representing the ratio of the electrical power output to the incident solar power. High PCE indicates better performance and is often the most reported parameter in research publications.
- Open-Circuit Voltage (Voc): This relates to the maximum voltage the solar cell can achieve under open-circuit conditions. Higher Voc generally contributes to improved PCE.
- Short-Circuit Current Density (Jsc): This indicates the current produced when the output terminals are shorted. Jsc is affected by the amount of light absorbed and the efficiency of charge carrier collection.
- Fill Factor (FF): This metric evaluates the quality of the solar cell. A higher fill factor suggests less energy loss within the cell, indicating better overall efficiency.
Understanding these metrics helps inform ongoing research and development to enhance the performance of perovskite solar cells. Each metric provides valuable feedback on the strengths and weaknesses of the solar cell design, paving the way for future innovations.
"Efforts to improve the efficiency of perovskite solar cells rely heavily on a strong grasp of these efficiency measurements."
Physical Principles of Perovskite Solar Cells
Understanding the physical principles of perovskite solar cells is crucial for grasping their efficiency potential and technological viability. The interplay of light absorption mechanisms, charge carrier dynamics, and recombination processes shapes how effectively these cells can convert sunlight into usable energy. Each of these elements contributes to the overall performance and longevity of the solar cells, which is especially significant when assessing their competitive standing against established technologies like silicon-based cells.
Light Absorption Mechanisms
Perovskite materials feature unique light absorption capabilities due to their exceptional bandgap properties. The bandgap of a perovskite solar cell can be tuned by modifying its composition, thus optimizing it for various light wavelengths. This tunability leads to enhanced absorption of light across a wider spectrum. A noteworthy aspect is that perovskite films achieve high absorbance with minimal thickness. This efficiency allows for reduced material use and potentially lower production costs.
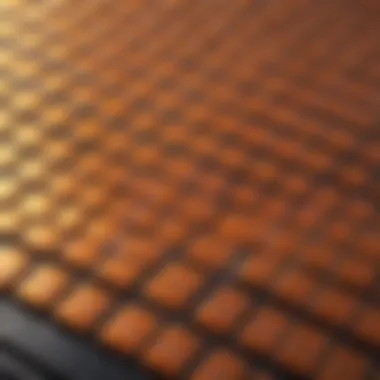
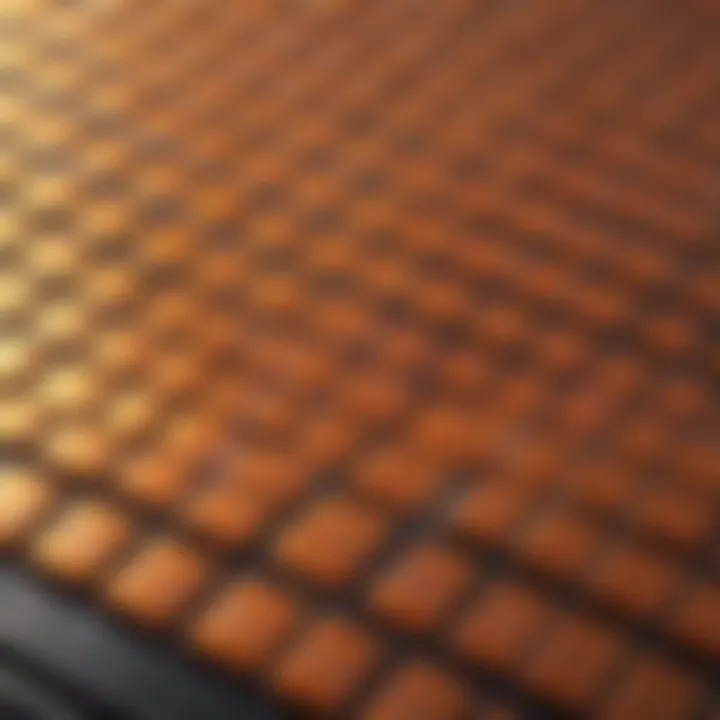
Moreover, high-quality perovskite films can absorb up to 99% of incident light, which is a significant advantage over other solar materials. The absorption process generates excitons—bound pairs of electrons and holes—which are crucial for current generation. The efficiency of this absorption mechanism directly influences the cell's ability to harness solar energy effectively and, thus, underscores the importance of selecting the right perovskite material composition.
Charge Carrier Dynamics
Once excitons are created in the perovskite layer, the next step involves their dissociation into free charge carriers—electrons and holes. This process is vital, as it determines how well a solar cell can generate power. In perovskite materials, the mobility of charge carriers is remarkably high. This means that electrons and holes can travel through the material with ease, leading to better current generation.
However, the dynamics also depend on how quickly these charge carriers can migrate to the respective electrodes. Efficient charge transport is essential; any delay can lead to energy loss and reduce overall efficiency. Additionally, the presence of defects or impurities within the material can interfere with this process, leading to decreased performance. Therefore, understanding and optimizing charge carrier dynamics in perovskite solar cells is fundamental to achieving higher efficiency.
Recombination Processes
While charge carriers need to reach electrodes for power generation, recombination processes can hinder their journey. Recombination occurs when electrons pair up with holes before they can contribute to the energy output. There are two types of recombination to consider: radiative recombination, which can be harnessed for light emission, and non-radiative recombination, which results in energy loss.
The rate of recombination is influenced by numerous factors, including material quality and environmental conditions. In perovskite solar cells, reducing the rate of non-radiative recombination is critical. Strategies to address this include passivating surface defects and optimizing layer configurations to minimize loss. Moreover, innovative material designs, such as using mixed halides, aim to enhance stability and performance by mitigating these losses.
"Understanding the balance between absorption, transport, and recombination processes is key to maximizing the efficiency of perovskite solar cells."
By diving into the physical principles governing these solar cells, researchers can devise methods to enhance performance, which is fundamental to advancing perovskite technology as a viable renewable energy source.
Recent Advances in Efficiency
Recent advances in the efficiency of perovskite solar cells have been marked by significant strides in material science and engineering. This topic is crucial as it addresses both the technological and economic viability of solar energy. Enhancing efficiency is not just about improving energy conversion; it implicates larger discussions about sustainability and the potential for wider adoption of renewable energy sources.
Research in this area is pivotal for several reasons:
- Higher Output: Increasing efficiency means that perovskite cells can produce more energy from the same amount of sunlight, making them more attractive alternatives to conventional silicon-based technologies.
- Cost-Effectiveness: As efficiencies rise, the cost per watt of solar energy decreases, thus improving the economic feasibility of solar installation for consumers and businesses alike.
- Integration Potential: Advanced efficiency opens doors for integration into various applications, including building-integrated photovoltaics and portable energy solutions.
Overall, the advances in efficiency are not merely technical achievements; they represent a pathway toward scaling up solar technology for global adoption. Now, we will delve into specific advancements that have contributed to boosting the efficiency of perovskite solar cells.
Hybrid Structures
Hybrid structures involve the combination of perovskite materials with other photovoltaic technologies. This approach leverages the benefits of multiple material systems to enhance overall performance. One prominent example is the integration of perovskite layers with silicon solar cells.
Such hybridization can yield efficiencies exceeding 30%, which is a significant improvement over traditional cells. The combination allows better light absorption and improved charge carrier dynamics. Furthermore, hybrid structures can exhibit better stability compared to standalone perovskite cells. This construction method also lends itself to addressing some of the common criticisms of perovskite technology, such as environmental concerns tied to certain materials.
Advanced Layering Techniques
Advanced layering techniques are key to optimizing perovskite cell performance. The precise engineering of layer thickness and material composition can greatly influence the light absorption and energy conversion processes. Techniques such as vapor deposition and solution processing are commonly used.
These methods allow for:
- Controlled thickness of the active layer affecting the light absorption efficiency.
- Tailored interface properties to minimize charge recombination, which is critical for maintaining high efficiency.
By experimenting with different layer configurations, researchers have achieved notable increases in power conversion efficiencies. This innovation not only enhances performance but also paves the way for more versatile applications in the energy sector.
Optimal Material Selection
Selecting the right materials is fundamental to enhancing the efficiency of perovskite solar cells. Perovskite materials, primarily comprising organic and inorganic components such as methylammonium lead iodide, have been the focus. However, research is diversifying to find suitable alternatives that improve stability and performance without compromising efficiency.
Key considerations in material selection include:
- Stability: Materials must endure environmental conditions without significant degradation.
- Bandgap Engineering: Choosing materials that enable effective light harvesting across a wider range of the solar spectrum.
- Toxicity Concerns: Investigating less toxic compounds can improve public acceptance and regulatory approval.
By focusing on optimal material selections, researchers aim to create a new generation of perovskite solar cells that combine high efficiency with minimal environmental impacts.
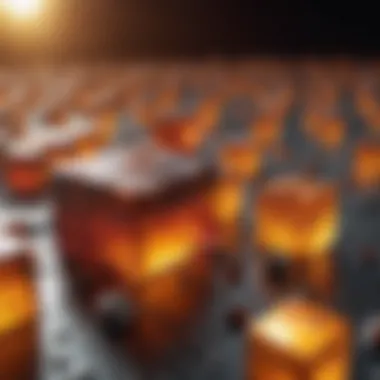
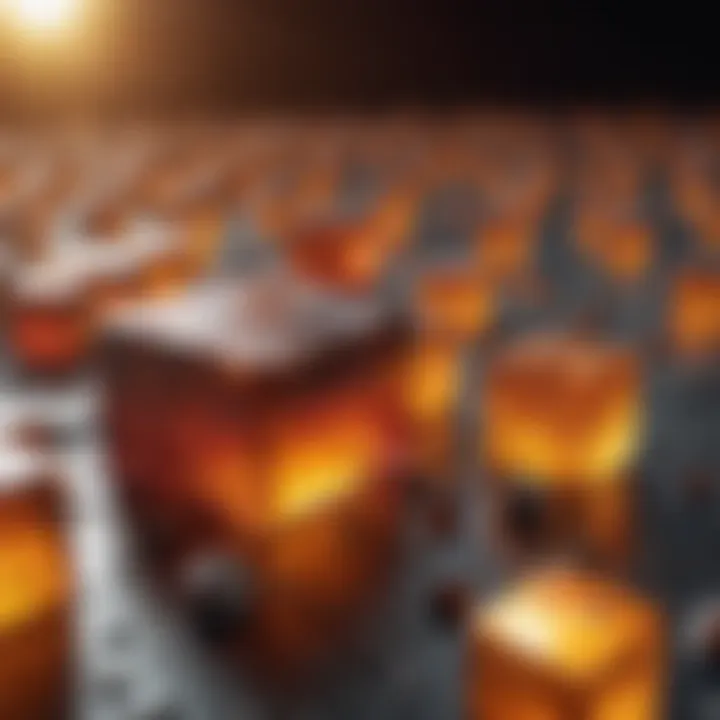
In summary, advances in hybrid structures, advanced layering techniques, and strategic material selection are essential components driving the efficiency of perovskite solar cells forward. These innovations not only address the immediate challenges facing solar technology today but also redefine what is possible for future applications in renewable energy.
Challenges in Achieving Higher Efficiency
Perovskite solar cells present remarkable potential in the field of renewable energy. However, there are significant challenges in achieving their maximum efficiency. These challenges stem from various factors that influence both material properties and the manufacturing processes. Understanding these obstacles is crucial for researchers and industry professionals aiming to commercialize perovskite technology. The primary areas of concern include material stability, degradation mechanisms, and issues related to manufacturing scale-up.
Material Stability
Material stability is one of the primary challenges facing perovskite solar cells. These materials, though easy to fabricate and adaptable, are inherently less stable compared to traditional silicon solar cells. Their performance can degrade rapidly under environmental stressors such as humidity, temperature fluctuations, and UV radiation.
Efforts to improve stability have led to the exploration of various composite materials and encapsulation techniques. Some researchers have focused on creating hybrid materials that blend organic and inorganic components. By improving the stability of perovskites, we can enhance their longevity and reliability in real-world applications. Material stability is pivotal not only for efficiency but also for the commercial viability of perovskite solar technologies.
Degradation Mechanisms
The degradation mechanisms in perovskite solar cells are complex and multifaceted. Factors such as ion migration, thermal stresses, and phase segregation contribute to the decline in performance. Ion migration, in particular, is a significant issue as it can lead to charge trap formation and reduced photocarrier collection.
To tackle these degradation mechanisms, researchers are investigating various additives and stabilizers that can mitigate the adverse effects. Understanding the root causes of these degradation processes enables the development of strategies to enhance cell lifetime. Addressing these issues not only aids in optimizing efficiency but is also essential for ensuring the sustainability of perovskite technologies in competitive solar markets.
Manufacturing Scale-up
Scaling up the manufacturing of perovskite solar cells presents its own set of challenges. The transition from laboratory to large-scale production involves numerous technical and economic considerations. The methods used for synthesis and deposition at a small scale often encounter issues at a larger scale due to uniformity, reproducibility, and material availability.
One important aspect is the need for standardized processes. By establishing clear protocols for scaling, manufacturers can enhance production efficiency and reduce costs. Collaborative efforts between academia and industry are significant for addressing manufacturing challenges. This cooperation can lead to innovations in techniques such as roll-to-roll processing and other methods aimed at improving scalability and overall production efficiency.
Comparative Efficiency Analysis
The comparative efficiency analysis is crucial in evaluating the performance of perovskite solar cells. This section highlights the fundamental aspects of comparing perovskite cells with traditional photovoltaic technologies and emerging alternatives. Understanding these comparisons helps in assessing the advantages and limitations of perovskite technology. Through rigorous analysis, researchers and industry professionals can determine the potential market viability of perovskite solar cells.
Comparison with Silicon-Based Cells
Silicon-based solar cells have dominated the market for decades due to their proven reliability and efficiency. However, perovskite solar cells offer exciting possibilities. The efficiency of silicon cells typically hovers around 20% to 25%, while perovskite cells can achieve efficiencies exceeding 25% in laboratory settings. This notable performance edge prompts the question of what makes perovskite materials so effective.
Key factors in this comparison include:
- Absorption Spectrum: Perovskite materials can capture a broader range of the solar spectrum. This characteristic allows for increased energy conversion compared to traditional silicon cells.
- Cost Efficiency: The production process for perovskite cells can be less expensive. This includes cheaper raw materials and simpler manufacturing techniques. This cost factor makes them appealing for widespread adoption.
- Mobility of Charge Carriers: Perovskite cells exhibit superior charge carrier mobility, which leads to enhanced photocurrent generation.
However, the comparison must also consider challenges associated with perovskites. They typically exhibit more significant stability concerns than their silicon counterparts. The long-term degradation rates of perovskite materials can affect overall lifespan and efficiency in real-world applications. Therefore, while the performance metrics are promising, the longevity of perovskite solar cells remains a key consideration.
Emerging Alternative Technologies
Beyond silicon and perovskite technologies, several emerging alternatives are entering the solar market. Each brings unique benefits and contributes to the competitive landscape of solar power solutions. Some notable technologies include:
- Cadmium Telluride (CdTe): Known for lower manufacturing costs, CdTe cells are efficient, but they face criticism due to the toxic nature of cadmium.
- Copper Indium Gallium Selenide (CIGS): CIGS cells can achieve high efficiencies but require more complex manufacturing processes than both silicon and perovskite technologies.
- Organic Photovoltaics: These cells utilize organic compounds for light absorption and exhibit flexibility. They are lightweight and can be produced at lower costs but often lack the efficiency and stability of inorganic options.
With various technologies vying for market share, comparative efficiency analysis provides valuable insights into their viability. Research and development continue to focus on optimizing these technologies, particularly the integration of perovskites with other materials to enhance overall performance. As the renewable energy market evolves, understanding these comparative dynamics will be essential for future innovations in solar technology.
Global Research Initiatives
The landscape of perovskite solar cells is heavily influenced by ongoing global research initiatives. As the demand for sustainable energy solutions grows, these initiatives play an important role in advancing the technology and addressing its challenges. Increased collaboration between academia, industry, and government entities is reshaping the research environment around perovskite solar cells.
To achieve higher efficiency and stability in perovskite solar cells, global research initiatives provide several key benefits. First, they foster knowledge sharing among leading experts from various fields. This collaboration leads to innovative solutions and novel approaches to persistent problems. Second, global initiatives often lead to the pooling of resources, enabling researchers to conduct larger and more impactful experiments. Third, such initiatives attract funding from multiple sources, which can substantially accelerate research progress.
Additionally, global research projects can align with national and international sustainability goals. This alignment not only amplifies the visibility of research outcomes but also ensures that developments are environmentally conscious and market-relevant. Researchers involved in these initiatives continuously explore the potential applications and implications of their findings, considering both technological advancement and environmental responsibility.
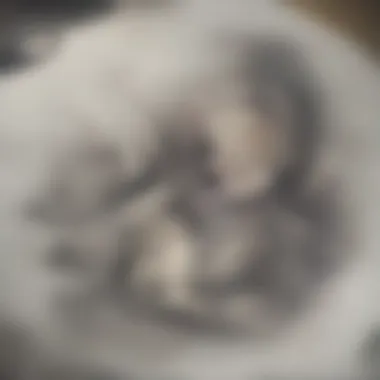
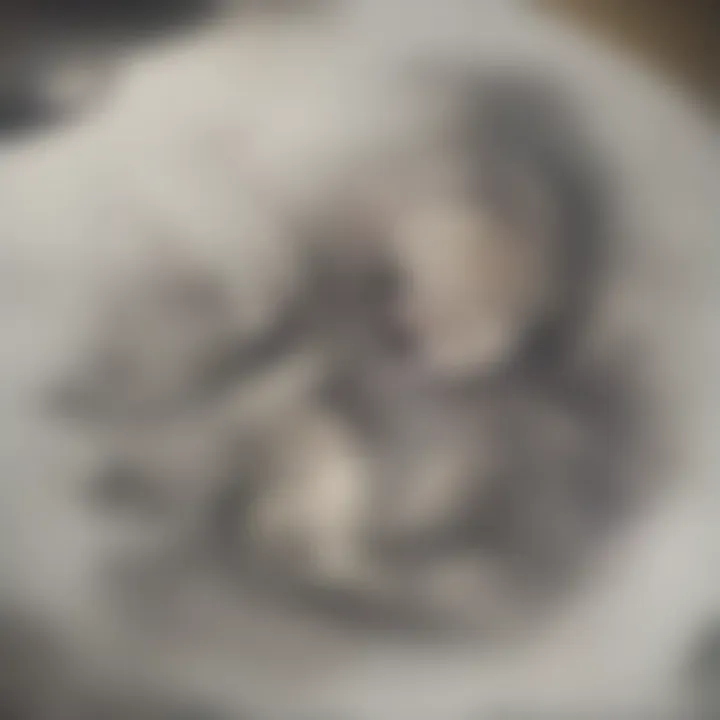
Leading Research Institutions
Leading institutions contribute significantly to progress in perovskite solar cell technology. Universities and research centers around the world are at the forefront of novel material development and efficiency measurement techniques. Notable institutions include the Massachusetts Institute of Technology (MIT), the California Institute of Technology (Caltech), and the University of Cambridge. These institutions have established groups that focus on perovskite material science, device engineering, and long-term stability testing.
These institutions attract some of the best minds in the field. Their collaborations often result in high-profile publications and significant breakthroughs. For instance, researchers at MIT developed a method to improve the stability of perovskite layers, which can potentially extend the lifecycle of solar cells.
Furthermore, investment in infrastructure allows these institutions to test large-scale prototypes. This testing informs future manufacturing processes and scales-up developments.
Collaborative Projects
Collaborative projects are critical in the global effort to enhance perovskite solar cell technology. Often, these projects involve multiple stakeholders, including universities, government research agencies, and private sector companies. By working together, distinct teams can address different aspects of the challenges associated with perovskite solar cells.
These projects often aim to improve various parameters, such as energy conversion efficiency and material durability. For example, the European Commission has funded numerous projects dedicated to integrating perovskite cells within existing photovoltaic systems. These projects seek to leverage the strong light absorption capabilities of perovskites while mitigating their weaknesses.
Collaborative efforts also emphasize education and training for new researchers. Understanding the complexities of perovskite technologies requires specialized knowledge. Projects often include training components to develop future expertise in the field.
In addition, collaborations extend beyond just technical advantages. They can also create a unified voice advocating for policy changes that favor renewable energy research and development. This aspect can significantly influence future investment and regulatory frameworks in favor of perovskite technologies.
Future Prospects for Perovskite Solar Cells
The future of perovskite solar cells holds significant promise in shaping the landscape of renewable energy. This section explores the potential applications and market predictions for these cells. It is essential to understand that perovskite solar technology is not only about increasing efficiency but also about making renewable energy more accessible and sustainable for diverse applications.
Potential Applications
Perovskite solar cells have a range of potential applications that could revolutionize energy generation. They are lightweight and flexible, allowing integration into various surfaces and structures. For instance:
- Building-Integrated Photovoltaics (BIPV): Perovskite cells can be incorporated into windows or building facades, efficiently converting sunlight into electricity without requiring additional land.
- Portable Electronics: Their light weight and flexibility make them suitable for charging devices such as smartphones and laptops.
- Agrivoltaics: These cells can be placed over agricultural areas, generating energy while providing shade for crops, potentially boosting yield.
- Space Applications: Their high efficiency and low weight are valuable in space missions where power efficiency and resource savings are critical.
The innovation in perovskite materials continues to expand, leading to even more versatile applications in energy generation, thereby contributing significantly to sustainable development.
Predictions for Market Adoption
As the technology matures, experts predict a gradual yet significant market adoption of perovskite solar cells. This could be driven by several factors:
- Cost Reduction: The production costs are decreasing, making it more financially viable compared to traditional technologies.
- Scalability: The manufacturing processes can be scaled up without compromising efficiency, which could lead to larger market deployments.
- Regulatory Support: Governments worldwide are implementing policies favoring sustainable energy solutions, which could enhance the adoption of such technologies.
- Increased Research Investment: Growth in research funding will foster innovations that improve durability and efficiency.
"The transition towards cleaner energy sources will largely depend on technologies that enhance efficiency and reduce costs, making perovskite a key player in the solar market soon."
Finale
The conclusion of this article carries significant weight in the overall understanding of perovskite solar cells. It synthesizes the discussion on efficiency, advances, and challenges, bringing together key insights that underline the potential of these emerging technologies.
Summary of Key Insights
In this article, we explored various aspects of perovskite solar cells.
- Material Composition: The unique structure of perovskite materials underpins their ability to achieve high efficiencies.
- Efficiency Metrics: We examined the theoretical maximum efficiency and critical metrics that quantify performance.
- Recent Advances: Innovations such as hybrid structures and advanced layering techniques have propelled progress.
- Challenges: Stability and degradation remained recurring themes, impacting practicality and scalability.
- Research Initiatives: Collaboration among leading institutions has been vital in driving advances in this area.
- Future Prospects: The potential applications and market adoption of these cells remain promising yet uncertain.
These insights suggest a landscape ripe for exploration, balancing hope with caution. The journey of optimizing perovskite solar cells is complex but crucial to sustainable energy transitions.
Need for Continued Research
The necessity for ongoing research cannot be overstated. As perovskite technology continues to mature, several areas require further investigation:
- Material Stability: Enhancing the resilience of perovskite compounds can mitigate degradation issues, extending the usable life of solar panels.
- Scalability: Developing efficient manufacturing processes to scale up production is essential for market entry.
- Efficiency Enhancements: Continuous exploration of novel materials and architecture will push efficiency boundaries.
Research also benefits from interdisciplinary approaches, merging insights from materials science, engineering, and environmental science. Maintaining a focus on both theoretical exploration and practical applications will be critical as we work toward integrating perovskite solar cells into existing energy systems.
Continued innovation is crucial to unlock the full potential of perovskite solar cells, shaping their future in sustainable energy.