Ferric Oxide Nanoparticles: Properties and Applications
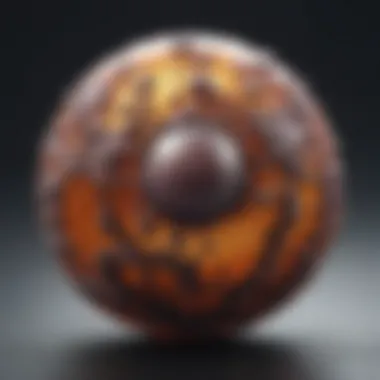
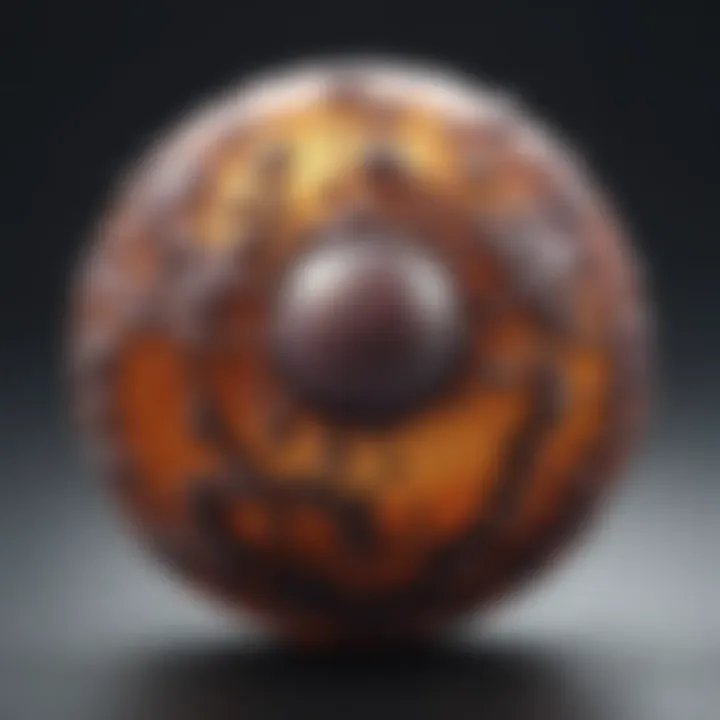
Intro
Ferric oxide nanoparticles have gained considerable attention in recent years due to their unique properties and versatile applications across diverse scientific disciplines. These nanoparticles, often represented by the formula Fe(_2)O(_3), are known for their magnetic characteristics and chemical stability, making them particularly valuable in fields such as biomedicine, environmental remediation, and materials science.
As researchers continue to explore the multifaceted potential of these nanoparticles, it becomes increasingly important to understand not only their intrinsic properties but also how they can be synthesized and applied effectively in real-world situations.
This narrative will unfold the significance of ferric oxide nanoparticles, diving into their distinctive features, various synthesis methods, and the challenges and implications they present within the scientific landscape.
Key Findings
Ferric oxide nanoparticles are remarkable for several key characteristics, which include:
- Superparamagnetism: Unlike larger particles, these nanoparticles display a unique ability to become magnetized in the presence of a magnetic field but lose their magnetism when the field is removed. This property is particularly useful in medical applications such as targeted drug delivery and magnetic resonance imaging (MRI).
- Biocompatibility and Non-toxicity: The small size and inherent stability of ferric oxide nanoparticles make them less harmful compared to some other nanoparticles, thus supporting their use in biomedical applications.
- High Surface Area: The increased surface area provided by their minuscule size allows for enhanced reactivity, making them excellent candidates for catalytic applications and environmental remediation.
Significance of Findings within the Scientific Community
The exploration of ferric oxide nanoparticles has significant implications. Their unique properties enhance not only existing applications but also pave the way for innovative uses that were previously thought to be out of reach. For instance, their utilization in drug delivery systems can improve the efficacy of treatments while minimizing side effects, thus revolutionizing how we approach patient care in medicine.
In addition, understanding their environmental implications can inform strategies for pollution control and remediation efforts. Researchers are eager to investigate not only how these nanoparticles can assist in cleaning up contaminants but also the effects they might pose to ecosystems, spurring debate and further investigation into their overall safety.
Implications of the Research
The discoveries surrounding ferric oxide nanoparticles extend far beyond the laboratory setting, carrying tangible applications in various fields:
- Biomedicine: They have shown promise in enhancing the effectiveness of chemotherapy drugs, as well as improving MRI imaging clarity.
- Environmental Applications: Their ability to absorb heavy metals makes them suitable for water purification efforts, presenting a potential solution to water scarcity and pollution issues.
- Data Storage and Electronics: Their magnetic properties may play a role in advancing technologies in data storage systems, impacting how digital information is stored and retrieved.
Potential Impact on Future Research Directions
Future research on ferric oxide nanoparticles is a moving target. As our understanding of their capabilities deepens, innovative applications are bound to emerge. Potential areas of research include:
- Nanotoxicology: Investigating the safety implications of exposure, especially in biomedical contexts.
- Customization of Properties: Developing methods to tailor the synthesis of ferric oxide nanoparticles to achieve desired properties for specific applications.
- Long-Term Environmental Impact Studies: Examining how these nanoparticles interact with biological systems and the environment over time is critical for their responsible deployment.
"Ferric oxide nanoparticles might just be the key to unlocking advancements across a myriad of fields, provided we tread carefully in understanding their full impact."
Prologue to Ferric Oxide Nanoparticles
In the field of nanotechnology, ferric oxide nanoparticles have emerged as significant players, catching the attention of researchers and professionals alike. These nanoscale materials, primarily composed of iron oxides like Fe2O3, exhibit unique properties that set them apart from their bulk counterparts. Understanding these properties is vital as they hold the key to numerous applications in areas ranging from biomedical science to environmental remediation.
Ferric oxide nanoparticles are prized for their magnetic characteristics, biocompatibility, and versatility. The capability to manipulate them at the nanoscale opens doors for innovative solutions that weren't possible before. For instance, in medical applications, they can increase the efficiency of drug delivery systems and improve imaging contrast in Magnetic Resonance Imaging (MRI). Moreover, their potential in sensing applications and in environmental clean-up makes them even more relevant.
As we further explore the topic, it’s essential to consider not only the advantages ferric oxide nanoparticles provide but also the challenges that come with their usage. Questions surrounding toxicity, environmental impact, and regulatory guidelines must be at the forefront of any discussion concerning their application.
Defining Ferric Oxide Nanoparticles
Ferric oxide nanoparticles are defined as tiny particles made predominantly of iron oxide that typically measure less than 100 nanometers in diameter. These diminutive particles can take on various forms, including magnetite (Fe3O4), hematite (Fe2O3), and maghemite (γ-Fe2O3). Their size gives them distinct physical and chemical properties, which facilitate many of their applications.
The unique semi-conducting properties, combined with their magnetic susceptibility, make ferric oxide nanoparticles an attractive option for various high-tech applications. The surface area-to-volume ratio increases dramatically as materials are reduced to the nanoscale, enhancing their reactivity and functionality.
Historical Context
Ferric oxide, or rust, as many might colloquially know it, has been around since ancient times, primarily recognized for its use in pigments and iron production. However, the scientific community's interest in its nanoscale form picked up significantly in the late 20th century. The development of nanotechnology opened new avenues for understanding and manipulating materials at the atomic and molecular levels.
In the last two decades, research saw a remarkable boom. This period has witnessed exponential growth in potential applications for ferric oxide nanoparticles, especially notable in the health and environment sectors. For example, researchers have explored their use as targeted drug delivery agents, where they can be steered to specific sites in the body using external magnetic fields.
While we reflect on the historical advancements, it's essential to remain aware of the evolving landscape. Each discovery adds a layer to our understanding of ferric oxide nanoparticles and propels further inquiry into their capabilities and implications.
Chemical Composition and Structure
Understanding the chemical composition and structure of ferric oxide nanoparticles is crucial. This knowledge lays a solid foundation for examining their properties and applications. Ferric oxide, primarily recognized by its chemical formula Fe2O3, comes in various polymorphic forms. Each variation holds distinct characteristics that can significantly influence how these nanoparticles behave in different environments and applications. This foundation ultimately aids researchers and professionals when selecting ferric oxide nanoparticles for specific tasks.
Chemical Formula and Types
Ferric oxide nanoparticles are commonly represented by the formula Fe2O3, and they can be categorized mainly into two types: maghemite and hematite. Maghemite (γ-Fe2O3) tends to have a cubic structure and showcases superparamagnetic properties, making it attractive for biomedical applications, such as targeted drug delivery and magnetic resonance imaging. Hematite ( α-Fe2O3), on the other hand, exhibits a more stable and less reactive structure, which is relevant in many environmental applications, especially in photocatalysis and heavy metal remediation.
- Maghemite (γ-Fe2O3):
- Hematite (α-Fe2O3):
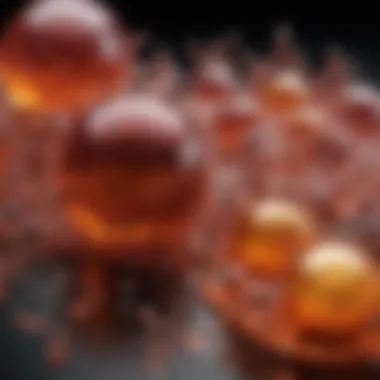
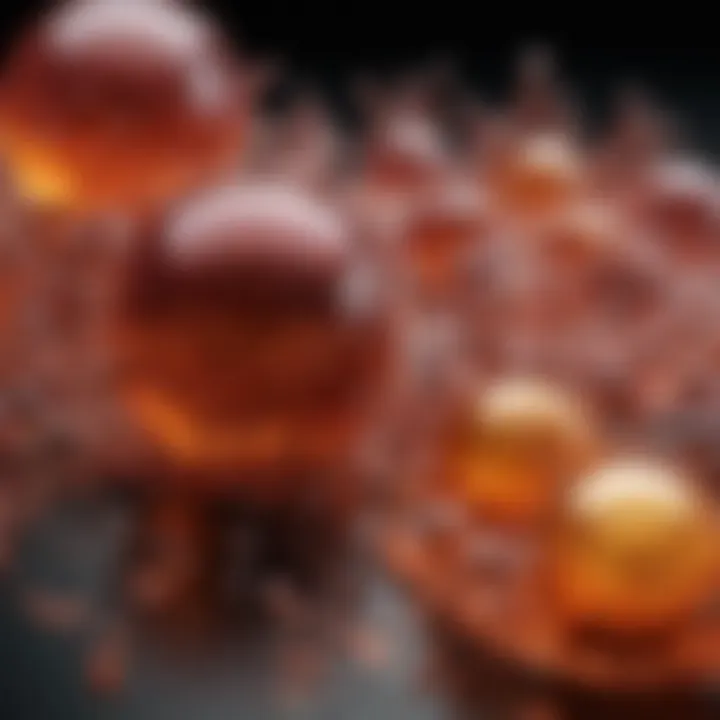
- Superparamagnetic properties.
- Suitable for biomedical applications.
- Stable structure.
- Used in environmental remediation.
The distinct properties arising from their composition and structure necessitate careful consideration when applying ferric oxide nanoparticles to various fields. The choice between these types depends on the intended application, thus influencing the performance and efficiency of nanoparticle use.
Nanostructure Morphology
The morphology of ferric oxide nanoparticles is another pivotal aspect that influences their functionality. Structurally, these nanoparticles can exist in several forms, including spherical, rod-like, or irregular shapes. The three-dimensional arrangement can significantly affect their physical and chemical properties, including surface area, magnetism, and reactivity. A higher surface area-to-volume ratio often improves catalytic performance and enhances interactions with biological systems.
Some key aspects of nanostructure morphology include:
- Size: Generally, nanoparticles range from 1 to 100 nanometers. This small size increases their mobility and potential reactivity.
- Shape: The shape can dictate how nanoparticles aggregate and interact with other materials.
- Uniformity: Consistent nanoparticles in size and shape can optimize performance in applications, particularly in drug delivery systems.
These morphological variations not only dictate the behavior of these nanoparticles in different media but also empower them to serve distinct purposes across various fields, ranging from medicine to environmental science.
Surface Properties
Surface properties of ferric oxide nanoparticles are vital in determining their interaction with surrounding environments. These properties include surface charge, hydrophobicity, and functional groups present on their surface. For example, the surface charge can impact stability, while the hydrophobic or hydrophilic nature can influence dispersibility in various solvents.
Key considerations regarding surface properties include:
- Surface Charge: Can dictate how nanoparticles stabilize in suspension. A favorable surface charge may reduce agglomeration, enhancing performance across applications.
- Functionalization: Tailoring surface chemistry to include specific functional groups can improve performance characteristics. For example, attaching biocompatible molecules can enhance drug delivery efficiency.
- Reactivity: Altering surface properties can modify the reactivity of ferric oxide nanoparticles, making them more suitable for catalyzing specific reactions or interactions with biological targets.
"The surface characteristics of ferric oxide nanoparticles are not just decorative; they play a crucial role in dictating functionality and efficiency in practical applications."
In essence, a comprehensive understanding of chemical composition, nanostructure morphology, and surface properties is expedient for grasping the full potential of ferric oxide nanoparticles. Each characteristic interplays to determine how these nanoparticles might shape future innovations in fields such as medicine, environmental science, and energy applications.
Synthesis Techniques
The area of synthesis techniques holds a significant position in the exploration of ferric oxide nanoparticles, laying the groundwork for their practical applications and enhanced functionalities. Understanding how these nanoparticles are synthesized allows researchers to manipulate their properties, tailoring them for specific purposes, whether that's improving their efficacy in drug delivery systems or their ability to remove contaminants from water. The choice of synthesis technique influences not only the structural properties of the nanoparticles but also their biological interactions and environmental impact. Thus, having a solid grasp of the various synthesis methods is critical for any endeavor involving these materials.
Top-Down Approaches
Top-down approaches involve breaking down larger bulk materials into nanoparticles. This can be accomplished using various methods like mechanical milling or lithography. Each method comes with its own pros and cons. For example, mechanical milling is straightforward and cost-effective, often relying on simple machinery. However, it does pose challenges in controlling the uniformity and size of the resulting particles. On the other hand, lithography can produce highly uniform nanoparticles but at a significant cost and complexity.
In practical terms, the mechanical milling process entails grinding down ferric oxide bulk material into fine particles, with sizes often reaching the nanoscale. This technique allows for a high yield of particles, although one must watch for potential contamination from the milling tools. One popular method here is planetary ball milling, where balls collide with the material to create fine particles.
Bottom-Up Methods
Bottom-up methods, in contrast, create nanoparticles from atomic or molecular precursors through chemical reactions. These techniques include sol-gel processes, co-precipitation, and chemical vapor deposition. Each method provides unique benefits that can considerably affect the final product's characteristics.
The sol-gel process is particularly notable for its ability to form nanoparticles at lower temperatures, thereby preventing possible oxidation or other unwanted chemical reactions. In this method, solutions are transformed into solid gels, which are then dried and heat-treated to yield ferric oxide nanoparticles. Conversely, the co-precipitation method brings together different iron salts in a solution, causing them to precipitate out as nanoparticles. This approach is especially valued for producing particles with controlled morphology and size, making it quite popular in various applications.
Comparative Efficiency of Methods
When we weigh the effectiveness of these methods, it's essential to consider factors like cost, scalability, and the properties of the resulting nanoparticles. Here’s a quick overview:
- Top-Down Approaches:
- Bottom-Up Methods:
- Advantages: Simplicity, high yields.
- Disadvantages: Less control over particle size, potential contamination.
- Advantages: Greater control over uniformity and size, high purity.
- Disadvantages: Often more complex and costly.
Characterization Techniques
Characterization techniques play a vital role in understanding the properties and behaviors of ferric oxide nanoparticles. These methods provide essential insights into their physical and chemical characteristics, which dictate their functionality and applications across various fields. Without a clear grasp of the nanoparticles' structure and properties, it’s challenging to harness their full potential or assess their environmental impacts.
The selection of the appropriate characterization technique directly affects the accuracy and relevance of the data gathered. Various methods, each with its strengths and limitations, contribute to a more comprehensive understanding of ferric oxide nanoparticles. This collective information is crucial for researchers and professionals alike, ensuring that ferric oxide is used efficiently and safely.
Microscopy Techniques
Microscopy techniques are indispensable for visualizing nanoparticles, revealing their size, shape, and distribution. Techniques like Transmission Electron Microscopy (TEM) and Scanning Electron Microscopy (SEM) provide high-resolution images and detailed morphology of ferric oxide nanoparticles. They allow researchers to observe minute details that are otherwise missed by conventional methods.
- Transmission Electron Microscopy (TEM) provides sharp images at the atomic scale. It enables investigators to discern internal structures and crystallinity, valuable for understanding synthesis processes.
- Scanning Electron Microscopy (SEM), while not as high in resolution as TEM, is quicker and reveals surface structures and textures, making it easier to analyze particle dispersion and aggregation states.
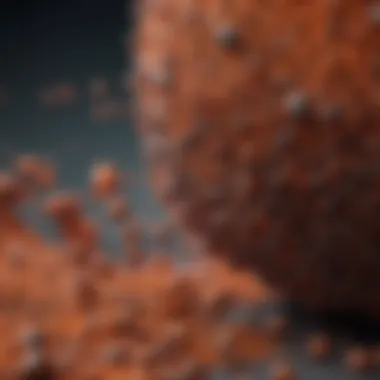
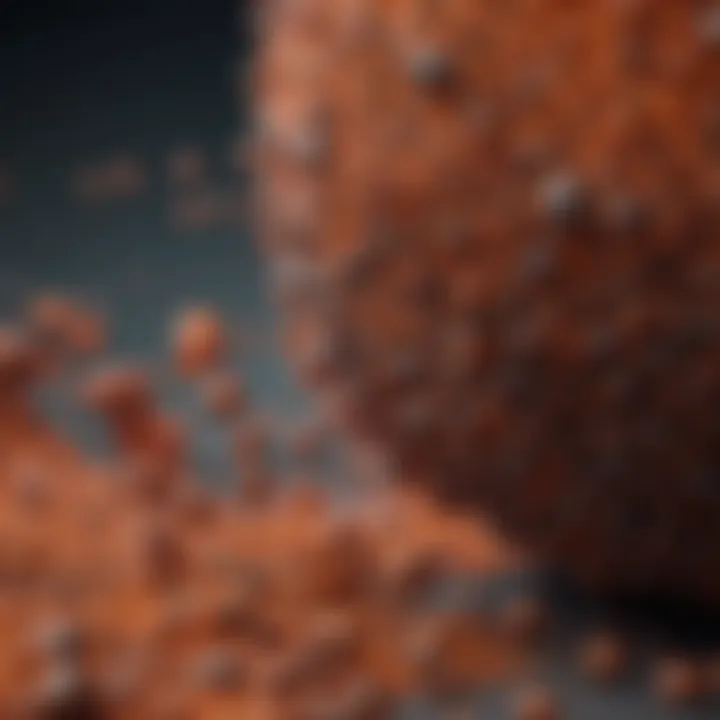
These techniques, combined, deliver a well-rounded perspective on how ferric oxide nanoparticles behave under different conditions, essential for tailoring them for specific applications.
Spectroscopic Methods
Spectroscopic methods extend beyond mere visual observation; they unveil the chemical properties of ferric oxide nanoparticles. Techniques such as Fourier-Transform Infrared Spectroscopy (FTIR) and Raman Spectroscopy offer insights into the molecular vibrations, chemical bonds, and functional groups present in the nanoparticles.
- Fourier-Transform Infrared Spectroscopy (FTIR) helps identify the chemical functionalities. It detects molecular vibrations, allowing for the assessment of surface modifications and interactions with other materials.
- Raman Spectroscopy complements FTIR findings by providing fingerprints of molecular species using inelastic scattering of light, giving further credence to the understanding of chemical compositions.
By employing these spectroscopic techniques, researchers can gather substantial data on the electronic and vibrational structures, establishing a solid foundation for predicting behaviors in various applications.
Thermogravimetric Analysis
Thermogravimetric Analysis (TGA) focuses on the thermal properties of ferric oxide nanoparticles, examining their stability and composition as the sample is heated. This method tracks changes in weight as a function of temperature or time, shedding light on crucial aspects of thermal stability and residue composition.
Through TGA, researchers can evaluate several factors:
- Decomposition temperatures that inform about stability in varying environments.
- Composition analysis, as different materials decompose at unique temperature profiles, thereby indicating the presence of certain elements.
Understanding these thermal characteristics is paramount when considering applications where temperature variations are prevalent, like energy storage or catalysis.
"The selection of the right characterization technique ultimately shapes the trajectory of research and application development in ferric oxide nanoparticles."
Applications of Ferric Oxide Nanoparticles
Ferric oxide nanoparticles are making quite the ripples across various fields, thanks to their unique properties and versatile applications. Their nano-scaled form grants them increased surface area and reactivity, making them invaluable in numerous advanced technologies. The discussion around their applications is not merely academic; it reflects the potential real-world improvements in medicine, environmental remediation, and energy storage. Understanding these applications also entails a recognition of the socio-economic implications, where effective utilization of ferric oxide nanoparticles may ease pressing global challenges.
In Medicine
Drug Delivery Systems
One notable application of ferric oxide nanoparticles is in drug delivery systems. These nanoparticles can be engineered to encapsulate medication, improving its therapeutic efficacy. The key characteristic here is their ability to be controlled magnetically, allowing for targeted delivery of drugs precisely where they're needed. This precision minimizes side effects typically associated with conventional drug delivery methods, making ferric oxide a popular choice in modern medicine.
The unique feature of these systems is their biocompatibility alongside magnetic properties. This dual advantage can significantly enhance delivery efficiency. However, it does raise questions about the long-term effects on human health, requiring thorough investigation.
Magnetic Resonance Imaging
Magnetic resonance imaging (MRI) is another area where ferric oxide nanoparticles show promise. They serve as contrast agents, improving the visibility of internal organs during imaging. One key characteristic of this application is its capacity for enhancing image quality. This enhancement ensures that even minute abnormalities can be detected, which is a major advantage in diagnostics.
The unique feature of this application lies in the ability of ferric oxide particles to provide clearer images at lower concentrations than traditional agents. While this is advantageous, complications may arise concerning the safety of these nanoparticles in long-term exposure, which warrants further scrutiny.
In Environmental Remediation
Heavy Metal Ion Removal
In environmental science, ferric oxide nanoparticles play a critical role in heavy metal ion removal from contaminated water sources. They can bind to metal ions, effectively pulling them out of solutions. The important aspect of this application is its efficiency; ferric oxide nanoparticles can operate in a variety of conditions, making them adaptable to different contamination scenarios. This adaptability makes them a favored option for addressing pollution effectively.
A unique feature of this method is the speed at which these nanoparticles can precipitate contaminants. However, challenges do exist, particularly regarding their disposal and potential re-release of absorbed metals back into environments, which may pose risks.
Water Purification
Water purification also benefits from the application of ferric oxide nanoparticles. They can optimize the removal of pollutants, ensuring cleaner water for drinking or industrial use. The key characteristic is their high reactivity, enabling rapid interaction with various contaminants. This reactivity illustrates why they are a promising choice in filtration systems.
However, the unique feature in this case is their specificity; ferric oxide nanoparticles can sometimes selectively capture certain harmful substances, which allows for tailored purification processes. Still, this specialization raises questions around cost-effectiveness and scalability when implemented at larger levels.
In Energy Storage
Battery Technology
Ferric oxide nanoparticles are increasingly being harnessed in battery technology as electrode materials. Their high electrical conductivity and stability over cycles are critical advantages, leading to improved battery performance. This characteristic makes them an attractive option, especially in the pursuit of more efficient energy storage solutions.
The unique feature of these nanoparticles lies in their ability to enhance the energy density of batteries. With the rising demand for portable energy sources, the integration of ferric oxide signifies a step toward longer-lasting batteries. Yet, there are disadvantages regarding the manufacturing costs and the need for further research into optimizing performance.
Photovoltaic Cells
Lastly, photovoltaic cells stand to gain from ferric oxide nanoparticles as well. By incorporating them into solar cells, one can potentially boost light absorption and energy conversion rates. The important element here is their ability to enhance efficiency, which is paramount as the world shifts toward renewable energy solutions.
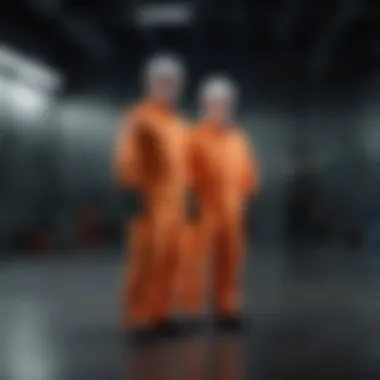
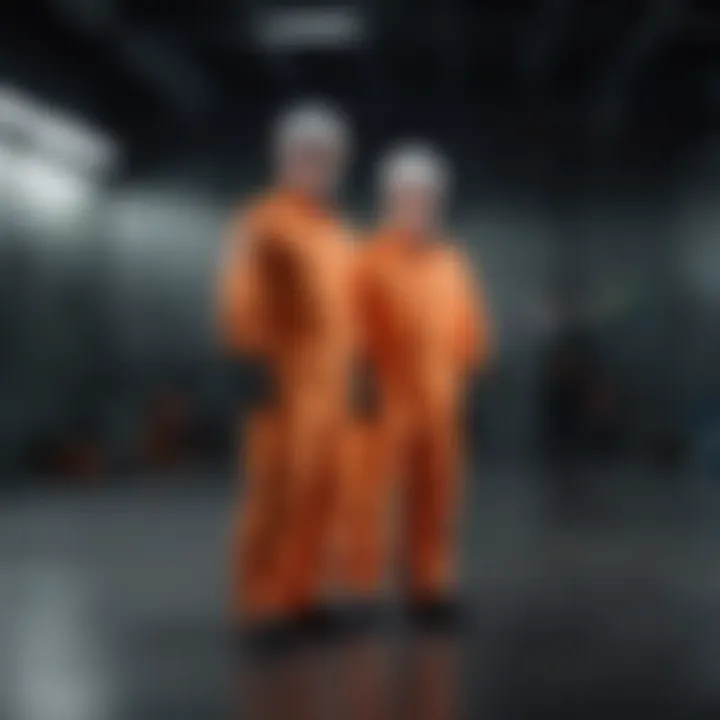
A defining feature of ferric oxide in this context is its durability under various environmental conditions, offering long-term reliability in energy harvesting. However, one should consider economic feasibility and production scalability, which can be barriers to widespread adoption.
Ferric oxide nanoparticles illustrate a pivotal bridge between scientific innovation and practical applications, touching on health, the environment, and sustainable energy.
Toxicity and Environmental Impact
Understanding the toxicity and environmental impact of ferric oxide nanoparticles is critical for comprehending their safety and efficacy in various applications. As these materials continue to gain ground in fields like medicine and environmental remediation, recognizing potential hazards linked with their use becomes vital. The analysis goes beyond just their beneficial properties; it digs into how they interact with biological systems and the ecosystem.
Potential Risks
Ferric oxide nanoparticles, like any chemical agents, can present several risks. These risks may vary depending on factors such as size, surface charge, and chemical composition. One of the primary concerns relates to their potential for bioaccumulation. When introduced into biological systems, nanoparticles can persist in living organisms. This raises the question of whether these particles might accumulate over time and cause toxic effects.
Moreover, their interaction with human cells can vary dramatically. While some studies show that ferric oxide nanoparticles can enhance drug delivery by penetrating cells more effectively, others have revealed cytotoxic effects at high concentrations. This duality highlights the importance of conducting thorough risk assessments before deploying these materials.
"Not all that glimmers is gold; caution must prevail where innovation is at stake."
Besides individual health risks, there are ecosystem-related concerns. The release of these nanoparticles into the environment can disrupt local fauna and flora. The particles may enter waterways, possibly affecting aquatic life and posing risks to the overall food chain. The factors influencing these risks include the particle's stability in different environmental conditions and its ability to degrade.
Regulatory Considerations
As the use of ferric oxide nanoparticles expands, regulatory frameworks are beginning to catch up. Multiple agencies are working to establish guidelines that ensure the safe utilization of these nanoparticles. Organizations like the Environmental Protection Agency (EPA) in the United States have initiated programs that focus on assessing the safety of nanomaterials across various industries.
A significant part of regulatory considerations revolves around the need for transparency and proper labeling. For instance, manufacturers are encouraged to disclose detailed information about the size, shape, and surface properties of the nanoparticles they use, as these attributes influence toxicity. The idea here is to create an informed public and incentivize safe practices in manufacturing and application.
Furthermore, there is an ongoing dialogue about standardized testing methods to assess the environmental impact of these materials. Unlike conventional substances, nanoparticles behave differently in biological systems, necessitating unique protocols for toxicity testing. Education and collaboration between scientists, regulatory bodies, and industry professionals are indispensable to create comprehensive standards that ensure both safety and effectiveness.
As researchers aim to balance benefits with potential downsides, a dense tapestry of regulations will likely evolve to encircle ferric oxide nanoparticles, ensuring their responsible usage in future applications.
Future Directions in Research
The study of ferric oxide nanoparticles continues to evolve, demanding attention to future directions in research. As we harness their potential across various fields, it’s essential to think ahead, ensuring that the methods we develop, and the applications we pursue are both innovative and responsible.
Efforts are underway to refine synthesis techniques and enhance the effectiveness of ferric oxide nanoparticles in existing applications. This not only increases their utility in areas such as biomedicine, environmental remediation, and energy storage but also plays a critical role in minimizing potential risks associated with their use.
Continuing research into the properties of these nanoparticles is crucial for broader implications. Scientists are tasked with addressing questions about their behavior in different environments and their interactions with biological systems, which can help mitigate any adverse effects on health and the ecosystem. Furthermore, research is geared towards developing regulatory frameworks that can keep pace with advancements in technology, ensuring safe and ethical use of these materials.
Emerging Trends
In recent years, several trends have emerged that signal a shift in how ferric oxide nanoparticles are understood and utilized. Some of these are:
- Smart drug delivery: Researchers are exploring the use of ferric oxide nanoparticles as vehicles for targeted drug delivery systems, aiming to enhance the precision and efficacy of treatments.
- Wastewater treatment innovations: Monitored studies indicate that ferric oxide nanoparticles can effectively remove contaminants from water, leading to more sustainable practices in environmental cleanup efforts.
- Nano-biosensors: The application of ferric oxide nanoparticles in sensors is gaining traction. Their magnetic properties enable the development of sensitive and selective detection methods for various compounds.
These emerging trends not only underline the versatility of ferric oxide nanoparticles but also emphasize the importance of continuous exploration across multiple scientific domains.
Interdisciplinary Approaches
Future research must embrace interdisciplinary approaches to unlock the full potential of ferric oxide nanoparticles. Collaboration among disciplines such as material science, biology, chemistry, and engineering can lead to breakthroughs that single-field studies could miss. This cross-pollination of ideas fosters creativity and innovation, allowing researchers to:
- Develop new methodologies that utilize combined expertise for enhanced synthesis techniques.
- Create comprehensive models that better predict the behavior of ferric oxide nanoparticles in real-world applications.
- Address ethical and environmental concerns through a multifaceted lens, pushing for regulatory measures based on solid scientific understanding.
By actively fostering these interdisciplinary connections, the scientific community can strive for a coordinated effort, ensuring the responsible and efficient advancement of ferric oxide nanoparticle technologies.
"Interdisciplinary collaboration offers a wealth of perspectives, enhancing our capacity to tackle complex challenges in nanoparticle research."
The exploration of ferric oxide nanoparticles will continue to thrive only through constant inquiry and a willingness to embrace new ideas, proving that the future of science relies on flexibility and cooperation.
The End
Understanding ferric oxide nanoparticles (FONPs) is not just an intellectual exercise; it's crucial for navigating modern scientific landscapes. The applications they bring to the table cut across various fields, from medicine to energy. Their unique properties make them versatile, but this versatility requires a thorough grasp of their implications.
Summary of Findings
Throughout the article, we've explored the myriad dimensions of ferric oxide nanoparticles. Here’s a concise distillation of the key findings:
- Defining Characteristics: Ferric oxide nanoparticles showcase distinct properties, such as magnetic traits and biocompatibility, making them suitable for various applications.
- Synthesis Techniques: Different methods of synthesis create nanoparticles with unique properties, affecting their efficacy in real-world applications. Both top-down and bottom-up approaches have their merits and challenges.
- Applications: From drug delivery systems in medicine to heavy metal ion removal in environmental remediation, the applications are vast and paramount. In energy storage, these nanoparticles contribute significantly to improving battery technology.
- Toxicity and Environmental Concerns: The potential risks associated with FONPs must not be overlooked. Regulatory considerations continue to shape how these materials are used, ensuring they benefit society without adverse implications.
- Future Directions: Emerging trends dictate a shift towards interdisciplinary collaboration, potentially unveiling novel applications and overcoming current limitations.
This comprehensive summary highlights how ferric oxide nanoparticles could influence diverse sectors if leveraged responsibly and innovatively.
Implications for Future Research
As we look ahead, several implications emerge for future research concerning ferric oxide nanoparticles.
- Enhanced Characterization Techniques: Continuous advancements in characterization methods will aid in understanding the precise behaviors of FONPs under various conditions. This will allow researchers to tailor their applications more effectively.
- Toxicology Studies: There is an urgent need for rigorous toxicity assessments. Understanding how these nanoparticles interact with biological systems will be crucial for safe applications, particularly in medical contexts.
- Sustainable Synthesis: Developing environmentally friendly synthesis methods could mitigate the potential negative impacts of these materials. Exploring greener alternatives ensures that advancements do not come at a steep environmental cost.
- Cross-Disciplinary Approaches: Bridging gaps between different scientific fields will likely yield innovative applications of ferric oxide nanoparticles ranging from innovative materials science to advanced medical therapies.