The Collision of Black Holes: A Cosmic Exploration
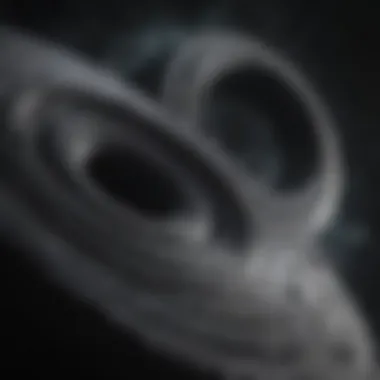
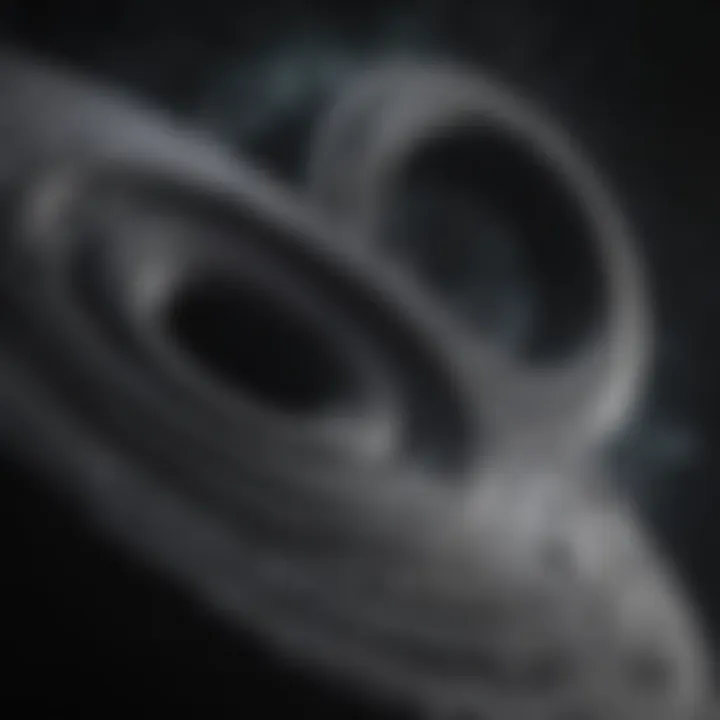
Intro
Black holes have long captured the imagination of scientists and the public alike. As enigmatic cosmic entities, they challenge our understanding of physics and the universe. The significance of black hole collisions extends far beyond mere curiosity. These events have profound implications for our understanding of gravity, spacetime, and even the very fabric of reality itself. The collisions of black holes provide insights into some of the most fundamental aspects of astrophysics.
This article will explore various facets of black hole collisions, including their mechanics, how they produce gravitational waves, and their influence on our understanding of the universe. It aims to elucidate the details surrounding these cosmic phenomena, catering to students, researchers, educators, and other professionals in the field.
Understanding black hole collisions is not just an academic exercise. These findings impact our understanding of the cosmos in tangible ways. By studying these events, scientists gain insights that can alter our view of mathematics, time, and even the origins of the universe.
The following sections will provide an in-depth analysis of the mechanics involved in black hole mergers and the implications that arise from these catastrophic occurrences. Furthermore, this exploration will highlight key findings in the realm of gravitational waves, which have resonated throughout the scientific community.
Key Findings
Summary of the main results
The recent advancements in our observational capabilities have yielded significant findings regarding black hole collisions. Observatories like LIGO and Virgo detected gravitational waves from these events, allowing scientists to gain unprecedented insights. Key results include:
- The confirmation of the existence of binary black hole systems.
- Evidence for the merger of black holes, providing data on their masses and spins.
- The detection of gravitational wave signals consistent with Einstein's general theory of relativity.
These findings support theoretical models and confirm predictions about the behavior of matter under extreme conditions.
Significance of findings within the scientific community
The discoveries surrounding black hole collisions have far-reaching implications. They validate theories of gravity and energy, reinforcing the foundations of modern astrophysics. Moreover, these mergers serve as laboratories for testing fundamental physics.
As scientists continue to analyze data from gravitational wave observatories, they are opening new frontiers in research. Notably, the study of binary black hole mergers has ushered in a new era in astrophysics, where observations can reveal the dynamics of powerful celestial events.
The detection of gravitational waves is a groundbreaking step in our understanding of the universe, representing the marriage of astronomy and fundamental physics.
Implications of the Research
Applications of findings in real-world scenarios
The implications of understanding black hole collisions extend beyond theoretical physics. These findings can inform various real-world applications, such as:
- Advancements in technology: The methods developed to detect gravitational waves may lead to innovations in measurement technology.
- Interdisciplinary research: Insights from black hole studies contribute to fields such as materials science, data analysis, and even quantum computing.
Potential impact on future research directions
The continuous study of black hole collisions promises to reshape current astrophysical paradigms. Future directions may encompass:
- Investigations into the nature of dark matter and dark energy through the lens of merged black hole data.
- An exploration of how these cosmic events affect galaxy formation and evolution.
- Enhanced understanding of gravitational wave physics, leading to further technological advancements in detection methods.
Preamble to Black Holes
Understanding black holes is crucial when studying the broader realm of astrophysics. They are phenomena that push the limits of our understanding of gravity and spacetime. By delving into the nature and types of black holes, we can better grasp the mechanics behind their collisions, which are among the most energetic events in the universe. Moreover, the significance of this topic extends beyond black holes themselves. It includes the study of gravitational waves and their implications for cosmology and our understanding of the universe.
Defining Black Holes
Black holes are regions in space where gravitational pull is so strong that nothing, not even light, can escape from them. Formally speaking, they are defined by their event horizon, a boundary beyond which information cannot escape. This unique property makes black holes some of the most fascinating objects in space. Their existence challenges classical physics and raises questions about the nature of reality itself. This crucial initial definition sets the stage for further exploration into their types, formation, and the mechanics of their collisions.
Types of Black Holes
Black holes are categorized primarily into three types: Stellar, Supermassive, and Intermediate black holes. Each type manifests distinct characteristics and plays unique roles in the cosmic landscape.
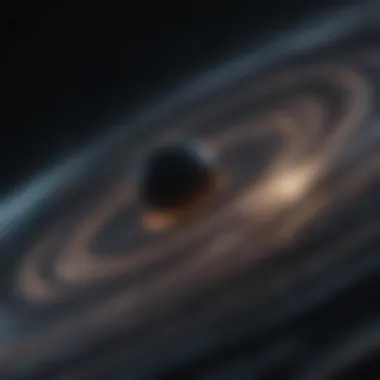
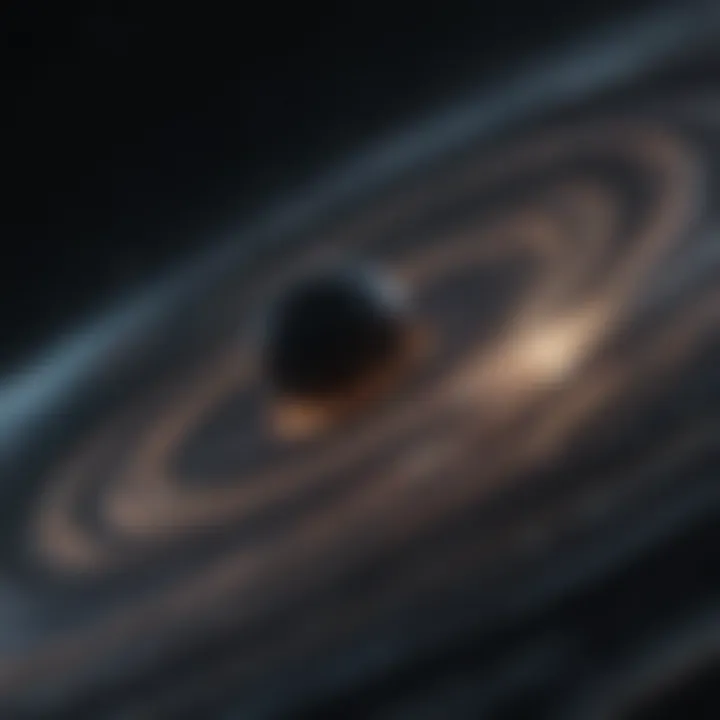
Stellar Black Holes
Stellar black holes form from the gravitational collapse of massive stars after they exhaust their nuclear fuel. The key characteristic of stellar black holes is their relatively small mass compared to other types, typically ranging from a few to a few tens of solar masses. This makes them a beneficial choice for the article, as they represent the most common type of black hole observed. A unique feature of stellar black holes is their potential to influence surrounding matter, leading to the formation of accretion disks. These disks can emit substantial amounts of radiation as material spirals inward, providing an observable signature of their presence. The simplicity of studying stellar black holes makes them an accessible entry point for those exploring the topic.
Supermassive Black Holes
Supermassive black holes are found at the centers of most galaxies, including our Milky Way. They are exceptionally massive, often containing millions to billions of solar masses. This immense size is a key characteristic that highlights their gravitational strength and influence on galaxy formation and behavior. The existence of supermassive black holes is a crucial aspect for this article because their gravitational fields significantly shape the galaxies they reside in. A unique feature of these black holes is their connection to quasars, highly luminous objects powered by accreting material. While challenging to study directly, supermassive black holes allow insights into early galaxy formation and evolution, making them a fundamental topic in cosmic studies.
Intermediate Black Holes
Intermediate black holes, which range from hundreds to thousands of solar masses, bridge the gap between stellar and supermassive black holes. Their existence is still somewhat of a mystery, and they pose unique challenges in terms of detection and study. The key aspect of intermediate black holes is their potential to provide insights into how black holes grow and evolve in different environments. They are considered a beneficial topic because they may shed light on the formation processes of supermassive black holes. Unique features of intermediate black holes include their potential formation in dense star clusters, setting them apart from their smaller and larger counterparts. However, their elusive nature and the difficulty in locating them represent a disadvantage in research.
Understanding these different types of black holes is essential to grasping the broader implications of black hole collisions and their consequences on the universe.
The Nature of Black Holes
Understanding the nature of black holes is crucial in grasping their role in the universe and the mechanics behind their collision. Black holes are not just cosmic curiosities; they significantly influence the dynamics of galaxies and the surrounding space. They challenge our existing theories of physics, especially in areas related to gravity and quantum mechanics. The nature of black holes includes their formation, characteristics such as the event horizon, and singularity. By exploring these aspects, we uncover why studying black holes matters not only for theoretical physics but also for practical astrophysics.
Formation of Black Holes
Gravitational Collapse
Gravitational collapse is one of the primary mechanisms leading to the formation of black holes. In simple terms, it occurs when a massive star exhausts its nuclear fuel. Without the outward pressure that nuclear fusion provides, gravity takes over, causing the star to collapse under its own weight. This collapse can lead to the formation of a black hole if the residual core mass exceeds the Tolman-Oppenheimer-Volkoff limit.
The key characteristic of gravitational collapse is its inevitability in stars with sufficient mass. It serves as a prominent example of how natural processes contribute to the existence of these enigmatic entities. Its significance in this article lies in the explanation of how ordinary stars transform into black holes, making it a foundational concept of astrophysical studies.
One unique feature of gravitational collapse is that it perfectly encapsulates the balance between gravity and the internal forces of a star. The advantage of focusing on this process is that it illustrates the eventual fate of massive stars, directly linking stellar life cycles to black hole existence. However, one must also acknowledge its limitations. Gravitational collapse alone does not account for other forms of black hole formation, such as those resulting from mergers of smaller black holes or the direct collapse of massive gas clouds.
Supernova Explosions
Supernova explosions, particularly of type II, also play a vital role in the formation of black holes. When a massive star reaches the end of its lifecycle, it can undergo a dramatic supernova event. During this event, the outer layers of the star are expelled into space in an immense explosion while the core collapses. If the core mass is high enough, it results in a black hole.
The key characteristic of supernova explosions is their capability to distribute heavy elements across the universe while also leading to the formation of black holes. This dual aspect makes supernovae a particularly enriching topic for this article, as they illustrate the interconnectedness of stellar evolution and cosmic recycling.
The unique feature of supernova explosions is their violence and aftermath, influencing surrounding stellar populations by enriching the interstellar medium and potentially triggering the formation of new stars. The advantage of discussing this process is that it highlights a spectacular end of a star's life while contributing to the cosmic ecosystem. However, supernovae also have their disadvantages, like the challenges in observing them and tying their outcomes directly to black hole formation, keeping the process somewhat elusive.
Event Horizon and Singularity
The event horizon and singularity represent two fundamental concepts related to black holes. The event horizon is the boundary beyond which nothing can escape the gravitational pull of a black hole. It marks the point of no return, where the escape velocity exceeds the speed of light. Understanding the event horizon is essential in studying the mechanics of black holes as it encapsulates the concept of boundaries in spacetime and serves as a trigger for numerous theories in physics.
Singularity, on the other hand, refers to a point at the center of a black hole where density becomes infinite and the laws of physics break down. It poses profound questions regarding our understanding of the universe, combining both gravitational and quantum theories. Together, the event horizon and singularity compel us to rethink established theories, interconnecting general relativity and quantum mechanics.
Mechanics of Black Hole Collisions
The study of black hole collisions reveals significant insights into the fabric of our universe. Understanding the mechanics behind these cosmic events allows researchers to grasp the complex interactions between massive objects influenced by gravitational forces. The collisions of black holes lead to the production of gravitational waves, which carry vital information regarding the collision dynamics and the characteristics of the involved black holes. This understanding also paves the way for advancements in astrophysics, particularly in the fields of cosmology and fundamental physics.
Gravitational Wave Emission
The Basics of Gravitational Waves
Gravitational waves are ripples in spacetime caused by accelerating masses, particularly during dramatic events like black hole mergers. They were first predicted by Albert Einstein in 1916 as a consequence of his General Theory of Relativity. The unique characteristic of gravitational waves is their ability to travel at the speed of light, providing a means to observe phenomena that are otherwise invisible. This is especially beneficial in understanding black hole collisions.
Detecting gravitational waves can reveal crucial information about the merging black holes, such as their masses, spins, and distance from Earth. The detection of these waves represents a significant advancement in observational astrophysics, offering a new way to study the universe. However, the technology required to detect them is highly sophisticated and must contend with noise from various sources, making the process challenging.
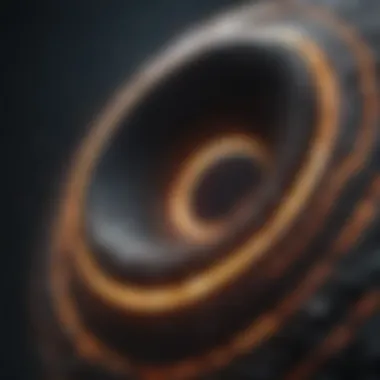
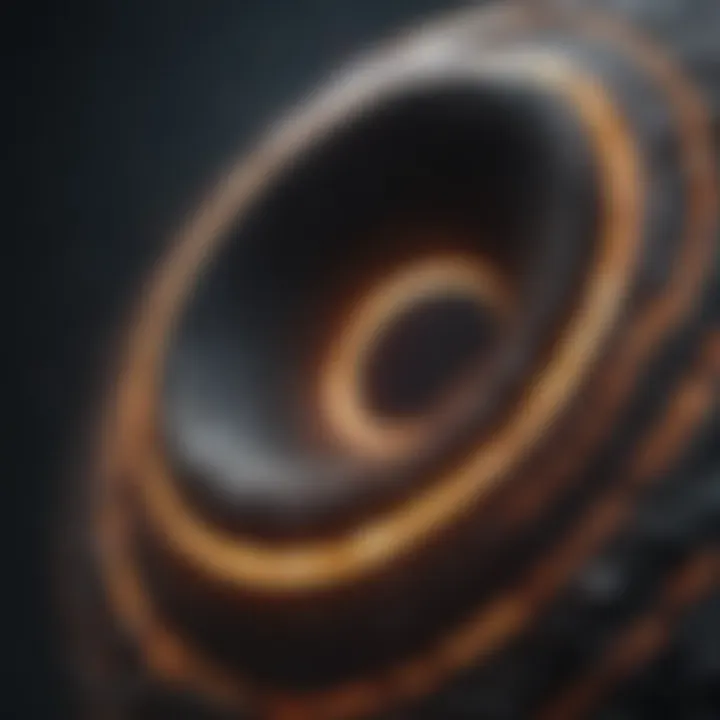
Detection Methods
Detection methods for gravitational waves have evolved significantly over recent years. The Laser Interferometer Gravitational-Wave Observatory (LIGO) is the most prominent example of detection technology. This innovative tool uses laser beams and interferometry to measure the minute changes in distances caused by passing gravitational waves. This method is a popular choice in this article because it provides powerful evidence of black hole mergers, having successfully detected several events since its first observation in 2015.
While LIGO's success has transformed the field, it does have limitations. The sensitivity of its measurements can be affected by environmental noise, which can mask weaker signals from more distant events. Olher detectors, such as Virgo and KAGRA, complement LIGO by providing additional data and perspectives on gravitational waves.
Phases of Black Hole Merger
Inspiral Phase
The inspiral phase of a black hole merger refers to the process where two black holes orbit each other, gradually spiraling inward due to gravitational wave emission. This phase is crucial for understanding the dynamics leading up to a merger, as it can last for billions of years. During this time, the orbital energy is radiated away as gravitational waves, causing the black holes to approach closer together.
What makes the inspiral phase significant is the predictable nature of gravitational wave signals emitted during this time. Researchers can simulate and model these waves to better understand the characteristics of the black holes involved. However, the challenge lies in the complexity of accurately predicting the signal forms and their behaviors as the black holes spiral inwards.
Merger Phase
The merger phase occurs when the two black holes collide and ultimately merge into a larger black hole. This process results in an intense release of energy, producing a strong gravitational wave signal that is detectable by observatories like LIGO. This phase is particularly essential for confirming theories about black hole formation and mass distribution in the universe.
The merger phase stands out due to the dramatic shift in dynamics as the black holes coalesce. This unique feature allows astrophysicists to study phenomena like the final masses and spins of resultant black holes. The transient nature of this phase makes it both rewarding and challenging to study, as capturing data requires precise timing in observation to align with event occurrences.
Ringdown Phase
After the merger, the newly formed black hole transitions into a stable state during the ringdown phase. In this phase, the black hole emits gravitational waves as it settles into equilibrium, characterized by oscillations or 'ringdowns' that decrease over time. This phase is critical for understanding the properties of the final black hole, including its mass and spin.
The significance of the ringdown phase lies in its informative gravitational wave signals, which carry information about the qualities of the black hole formed. While it is less intense than the merger phase, the data help improve our understanding of black hole characteristics and the fundamental nature of gravity. However, its relatively short duration poses challenges in capturing its details compared to the inspiral and merger phases.
Observational Evidence
Observational evidence plays a crucial role in the study of black hole collisions. This evidence serves as the foundation for understanding not only the mechanics of these cosmic events but also their implications in astrophysics. Through various methods of observation, scientists gain insights into the characteristics and behaviors of black holes as they collide, offering a more nuanced view of their nature and the universe at large.
Key Observations in Astrophysics
LIGO Discoveries
The Laser Interferometer Gravitational-Wave Observatory, commonly known as LIGO, marks a significant advancement in astrophysical observations. One of its primary contributions is the detection of gravitational waves produced by black hole collisions. This technology allows scientists to observe events that are invisible through traditional electromagnetic means. The key characteristic of LIGO is its sensitivity to the minute distortions in spacetime.
LIGO's distinctiveness lies in its ability to capture these fluctuations, which emerge from energy released during black hole mergers. An important advantage of LIGO is that it enables scientists to verify and examine the predictions made by Einstein’s general theory of relativity. However, its effective operation requires precise conditions and sophisticated technology, potentially limiting observational opportunities.
Observations from Space Telescopes
Space telescopes provide another layer of observational capability when it comes to studying black hole collisions. Instruments like the Hubble Space Telescope allow direct observation of the events leading to black hole mergers. Their ability to capture high-resolution images across various wavelengths offers unique insights into the environment surrounding black holes.
The key characteristic of space telescopes is their advantage of being above Earth's atmosphere, which affects ground-based observations by creating disturbances. This feature makes space telescopes highly beneficial for gathering data about distant galaxies where black holes are located. One unique aspect of these observations is the potential to study the formation and evolution of black holes. Nonetheless, high costs and complex operations may limit the frequency and extent of observations.
Significance of Observations
The observations obtained through both LIGO and space telescopes have immense significance in expanding our knowledge of black holes. They not only confirm theoretical models but also challenge current paradigms in astrophysics. With accurate observational data, researchers can refine their understanding of gravitational waves, black hole formation, and the universe's overall dynamics. The insights derived from these observations contribute to the ongoing narrative in astrophysics, emphasizing the complexity and wonder of cosmic phenomena.
Impact on Cosmology
The investigation of black hole collisions offers vital insights into the evolving understanding of cosmology. Each merger serves as a cosmic laboratory, revealing intricate processes that shape the universe. Gravitational waves, produced during these events, present novel information about the fabric of space-time. The study of such collisions illuminates both the mechanics of black hole mergers and their cultural impact on galaxy formation.
Role in Galaxy Formation
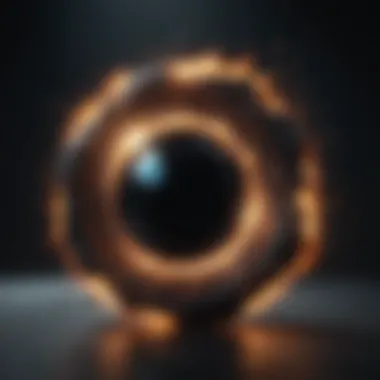
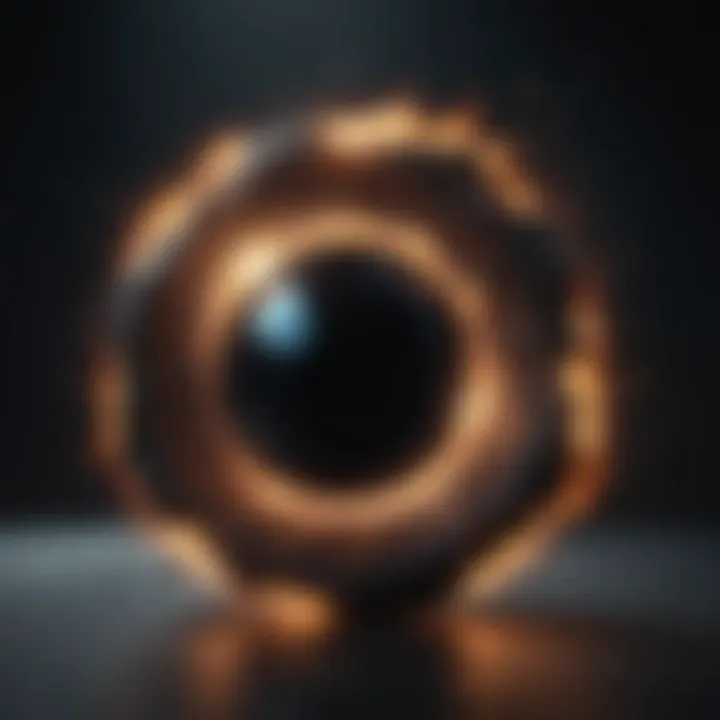
The role of black holes in galaxy formation cannot be overstated. Supermassive black holes are often found at the centers of galaxies, and their formation is closely tied to the development of the galaxies themselves. The mass and gravitational influence exerted by these black holes contribute to the dynamic nature of their galactic surroundings. As galaxies form and evolve, the interaction between stars and their central black holes becomes evident.
- Regulating Star Formation: Black holes can influence the rate at which stars form in their host galaxy. Feedback mechanisms, such as energy released during black hole accretion, may inhibit or enhance star formation within nearby gas clouds.
- Shaping Galactic Structure: The matter accretion for supermassive black holes impacts the structure of galaxies. This process leads to the formation of galaxy bulges and balances the surrounding stellar densities.
- Mergers Leading to Growth: When galaxies collide, their respective black holes may also merge. This merger facilitates size growth, contributing to the population of supermassive black holes over cosmic time.
Understanding the connection between black holes and galaxy formation is crucial for developing comprehensive models of the universe's evolution.
Understanding Dark Matter and Dark Energy
Black holes may also provide significant clues about dark matter and dark energy, two of the most enigmatic components of our universe. While their exact natures remain unclear, black hole collisions can potentially offer insights into their influences on cosmic phenomena.
- Dark Matter Interaction: Black holes may be involved in gravitational interactions with dark matter. Observations related to black holes can yield indirect information about the properties and distribution of dark matter in galaxies. This affects the galactic rotation curves, which continue to perplex astronomers.
- Dark Energy Implications: The expansion of the universe is believed to be driven by dark energy. Black holes contribute to our understanding of how this expansion affects cosmic structures over time. Observational data from black hole collisions can illuminate the relationships between dark energy and the large-scale structure of the universe.
- Testing Cosmological Models: Research on black hole collisions also serves as a testing ground for cosmological models, including those that attempt to unify various fundamental forces. These models help scientists understand the complexities of the universe and refine theories pertaining to black holes, dark matter, and dark energy.
In summary, the impact of black hole collisions extends beyond immediate astrophysical phenomena. It encompasses profound implications for understanding galaxy formation and addressing the mysteries surrounding dark matter and dark energy.
Future Research Directions
The exploration of black hole collisions opens a doorway to deeper cosmic mysteries. The urgency of advancing research in this field is paramount as it has the potential to unlock fundamental knowledge about the universe. By understanding the aftereffects of cosmic events such as black hole mergers, scientists can address larger questions about the formation and evolution of galaxies. Moreover, the implications extend to concepts involving dark matter and dark energy, which remain elusive in modern physics.
Advancements in Technology
Technological progress is crucial for enhancing our capability to study black hole collisions. Instrumentation improvements in gravitational wave observatories like LIGO and Virgo have revolutionized our approach to detecting these cosmic phenomena. The detection of gravitational waves allows researchers to gather data on the characteristics of merging black holes, such as their masses and spins.
Future advancements may include the development of space-based observatories, improving sensitivity even further. Projects like the Laser Interferometer Space Antenna (LISA) promise to measure waves from lower-frequency events that traditional observatories cannot detect. This technology enables a broader frequency range in gravitational wave detection, leading to a more comprehensive understanding of black hole dynamics.
Expanding Theoretical Frameworks
Theoretical research accompanying technological advancements is equally vital. The expansion of theoretical frameworks is necessary to interpret the data collected from black hole collisions. Concepts like the information paradox and Hawking radiation require rigorous mathematical models to explore their implications on quantum gravity.
Furthermore, new theories could integrate insights from different areas of physics, bridging gaps between general relativity and quantum mechanics. Such integrations can lead to a unified theory that explains black holes not only as physical entities but also in the context of the universe's life cycle.
Pursuing these research directions allows astrophysicists to refine existing theories or even develop radically new paradigms in understanding cosmic phenomena.
The future of black hole research must embrace multi-disciplinary approaches, drawing from fields such as computational astrophysics and data science. This collaboration will ensure that our understanding of these complex phenomena continues to evolve, and as a result, the broader implications for the structure and fate of the universe can be systematically addressed.
The End
The discussion surrounding black hole collisions proves to be of paramount importance in astrophysics. Understanding these cosmic phenomena not only unravels the complexities of black holes themselves but also contributes significantly to our comprehension of the universe. The article presents several key elements that outline this significance.
Firstly, black hole mergers serve as vital tools for testing theories of general relativity. As gravitational waves radiate from these events, they provide empirical data that scientists can analyze, affirming or challenging existing frameworks. The implications of this research extend beyond theoretical physics; they offer insights into the fundamental laws governing our universe.
Secondly, the observational evidence gathered from detectors like LIGO has transformed how we perceive the cosmos. These observations have allowed us to witness phenomena that were previously mere conjectures. With each new collision detected, a clearer picture of our universe’s architecture emerges, reshaping our understanding of galactic evolution and structure.
Furthermore, the collisions of black holes also hint at deeper mysteries, such as the roles of dark matter and dark energy. As researchers explore the gravitational dynamics of these events, they may uncover vital information regarding the unseen forces that shape the cosmos.
The ongoing journey in astrophysics, fueled by technology and innovation, showcases that the study of black holes remains a burgeoning field. As our methods of detection and theoretical models continuously evolve, so shall our understanding of the universe and its intricate phenomena.
Summary of Key Points
- Black hole mergers are essential for testing general relativity.
- Observatories like LIGO play a crucial role in expanding our knowledge.
- These events may provide insights into dark matter and dark energy.
- Technological advancements will continue to enhance our research capabilities.
The Ongoing Journey in Astrophysics
The exploration of black hole collisions is not static; it is a dynamic and ongoing journey. Researchers and astronomers constantly strive to develop new technologies and methodologies to probe deeper into these cosmic wonders.
As we move forward, we are poised to uncover new theories that could transform our comprehension of space-time and the fabric of the universe itself. Innovative projects, such as the Laser Interferometer Space Antenna (LISA), are on the horizon, which promise to revolutionize our ability to observe gravitational waves.
Moreover, collaborations across the globe foster an environment where shared knowledge accelerates discoveries. Conferences, academic papers, and communal discussions enrich the astrophysics community, driving prospective research directions.
In summary, the journey into understanding black holes and their collisions is essential. Each advancement not only enhances our knowledge but also opens up myriad avenues for inquiry that could redefine our place in the cosmos.