Bacterial DNA Extraction Protocols Explained
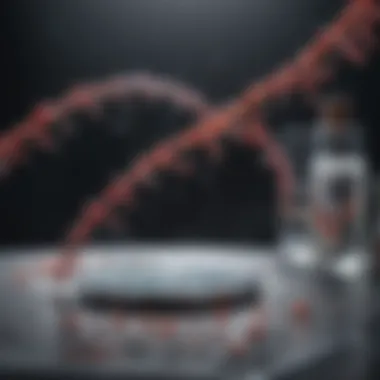
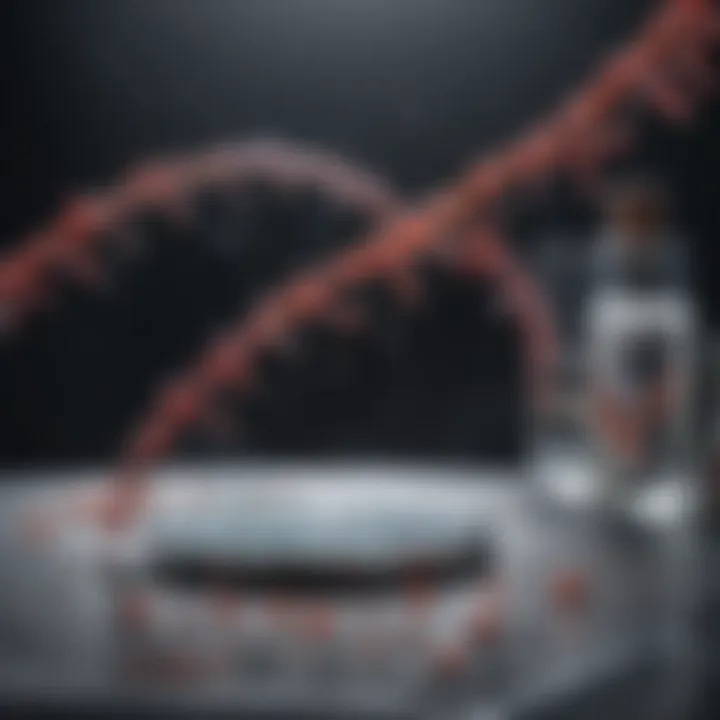
Intro
The extraction of bacterial DNA stands as a cornerstone in the field of molecular biology. Understanding how to effectively obtain this genetic material is crucial, not only for research but also for clinical applications. Bacteria, with their vast diversity, provide insights into various biological processes, and the ability to isolate their DNA opens avenues for numerous scientific endeavors. This process, while seemingly straightforward, involves a multitude of steps, each requiring precision and care.
In this comprehensive exploration, we dissect the protocols for bacterial DNA extraction, shedding light on the methodologies employed, the challenges faced, and the implications of these techniques. By delving into the nuts and bolts of extraction protocols, we aim to equip researchers, educators, and enthusiasts with a detailed understanding, making it accessible even for those not steeped in molecular biology.
Key findings within this area not only enrich academic discussions but also lay the groundwork for practical applications. From environmental microbial studies to clinical diagnostics, the role of extracted bacterial DNA cannot be overstated. Throughout this article, we will touch upon the significance of these findings and how they shape future research directions.
Prelims to Bacterial DNA Extraction
Understanding bacterial DNA extraction is fundamental to microbiology and molecular biology at large. This process unlocks a treasure trove of genetic information from microorganisms, serving not just research interests but also practical applications across various industries. The extraction of DNA from bacteria paves the way for advancements in medicine, agriculture, and environmental science, among other fields.
Bacterial DNA extraction is significant for several reasons. First, it facilitates studies related to genetic diversity, evolution, and the role of bacteria in ecosystems. Moreover, the insights gleaned from bacterial genomes can direct research towards combating antibiotic resistance and understanding pathogenic mechanisms. This knowledge is a stepping stone toward developing targeted therapeutics and diagnostic tools.
Significance of DNA Extraction
DNA extraction serves as the cornerstone of molecular biology. Its significance cannot be overstated. By isolating genetic material, researchers can analyze genes, study mutations, and explore gene expression in bacteria. This is not merely a matter of academic curiosity; it has real-world implications. For instance, in clinical settings, extracting bacterial DNA is crucial for identifying pathogens in infections, guiding treatment plans, and monitoring the spread of antibiotic resistance.
Furthermore, examining bacterial DNA enhances our understanding of microbial ecology. It sheds light on how microorganisms adapt to changing environments and interact with their surroundings. This level of insight is key to biotechnological advancements. For example, specific bacterial strains are harnessed in bioremediation to detoxify polluted environments, thanks to their unique genetic traits.
Common Applications in Research and Industry
The applications of bacterial DNA extraction span across various domains:
- Molecular Diagnostics: Testing for bacterial infections and guiding treatment decisions relies heavily on accurately extracting bacterial DNA.
- Biotechnology: Genetic engineering utilizes extracted DNA to manipulate bacterial cells, producing insulin or other therapeutics.
- Environmental Monitoring: Bacterial DNA analysis can assess ecosystems' health through biodiversity evaluations.
- Synthetic Biology: Engineered bacteria are designed for specific functions, such as producing biofuels or pharmaceuticals, based on the extracted genetic material.
- Food Safety Tests: Detecting foodborne pathogens via DNA analysis safeguards public health by preventing outbreaks.
"Without the ability to extract and study DNA, countless scientific breakthroughs would simply not exist."
Overview of DNA Structure
Understanding the structure of DNA is foundational to grasping the nuances of bacterial DNA extraction protocols. DNA essentially serves as the blueprint for life, dictating the processes that occur within every organism. This section aims to elucidate the specific structural characteristics of bacterial DNA, paving the way for practical extraction methods and enhanced comprehension of genetic manipulation in microbial systems.
Basic Components of Bacterial DNA
Bacterial DNA differs significantly from the DNA found in higher organisms, mainly in its structure and organization. The fundamental components of bacterial DNA include:
- Nucleotides: The building blocks of DNA, each comprised of a sugar, phosphate group, and nitrogenous base. In bacteria, these nucleotides form long chains that create the double helix structure.
- Double Helix Formation: Bacterial DNA is predominantly circular and exists as a double-stranded helix. When it comes to size, bacterial genomes can vary widely, but they are generally far smaller than those of eukaryotic counterparts.
- Plasmids: These are additional small, circular pieces of DNA that can replicate independently of chromosomal DNA. Plasmids often carry genes that confer beneficial traits, such as antibiotic resistance, making them crucial for survival and adaptation.
The unique circular structure is key to the extraction processes—understanding this allows researchers to tailor their methods effectively. It is essential while designing protocols to optimize yield and purity, considering that the circular form requires more specific lysis and separation techniques than the linear configurations seen in eukaryotes.
Comparison with Eukaryotic DNA
When drawing a parallel between bacterial and eukaryotic DNA, a few critical differences stand out:
- Structure Variations: As mentioned before, bacterial DNA is typically circular, while eukaryotic DNA is linear and encapsulated within a nucleus. This major distinction has implications during extraction since the methods must be adapted to account for these physical forms.
- Gene Density: Bacterial genomes exhibit higher gene density with minimal non-coding regions compared to eukaryotes. This has significant ramifications when optimizing for yield, as the extraction of bacterial DNA often yields informative, functionally relevant sequences more directly conducive to research applications.
- Introns and Exons: In eukaryotes, genes often contain introns that are spliced out during mRNA processing. Conversely, bacterial genes typically lack introns, leading to more straightforward extraction and analysis of coding sequences. This feature simplifies downstream applications in molecular biology, as the sequences extracted are more likely to encode directly for functional proteins
The differences in DNA structure not only facilitate a better understanding of extraction protocols but also impact the downstream applications in genetic research and biotechnology.
In summary, the examination of the structure of bacterial DNA serves as an essential cornerstone for DNA extraction methodologies. Recognizing these differences equips researchers and students alike with the knowledge to navigate the complexities of extracting and utilizing bacterial DNA efficiently. This understanding will enable practitioners to tap into the myriad opportunities presented by bacterial genetic material in fields ranging from genetic engineering to clinical diagnostics.
Key Steps in Bacterial DNA Extraction Protocol
Understanding the key steps in bacterial DNA extraction is crucial for anyone involved in molecular biology. This process forms the backbone of modern research and applications in areas like diagnostics and genetic engineering. Each step is meticulously designed to ensure the integrity and yield of the DNA extracted. Everyone who deals with bacterial samples must recognize the importance of these steps, as they can often become the bottleneck in research workflows. Thus, a firm grasp on the operational intricacies can significantly enhance the quality and reliability of results obtained in subsequent analyses.
Sample Collection and Preparation
The journey of DNA extraction begins long before the actual lysis of cells. Sample collection and preparation are pivotal. The quality of the bacterial sample directly affects the downstream processes. Therefore, it is essential to ensure that samples are collected with aseptic techniques to minimize contamination.
Key points to consider include:
- Selection of Appropriate Culture Conditions: The growth media and conditions must be tailored to promote the growth of the target bacteria while inhibiting contaminants. Different strains may have specific nutritional needs, so understanding the characteristics of the bacteria is essential.
- Timing of Collection: Harvesting samples at the right growth phase, typically the log phase, can yield better DNA quality. Cells during this phase are actively dividing, which usually allows for a higher yield of intact DNA.
- Storage Requirements: Once collected, samples should be stored under conditions that maintain their viability. Often, this involves freezing, but depending on the species, stored samples may require specific temperatures.
In summary, optimal sample collection and preparation can dramatically impact the outcomes of bacterial DNA extraction methods.
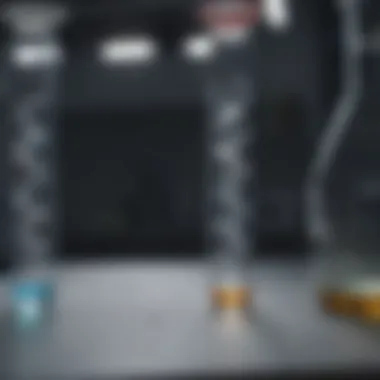
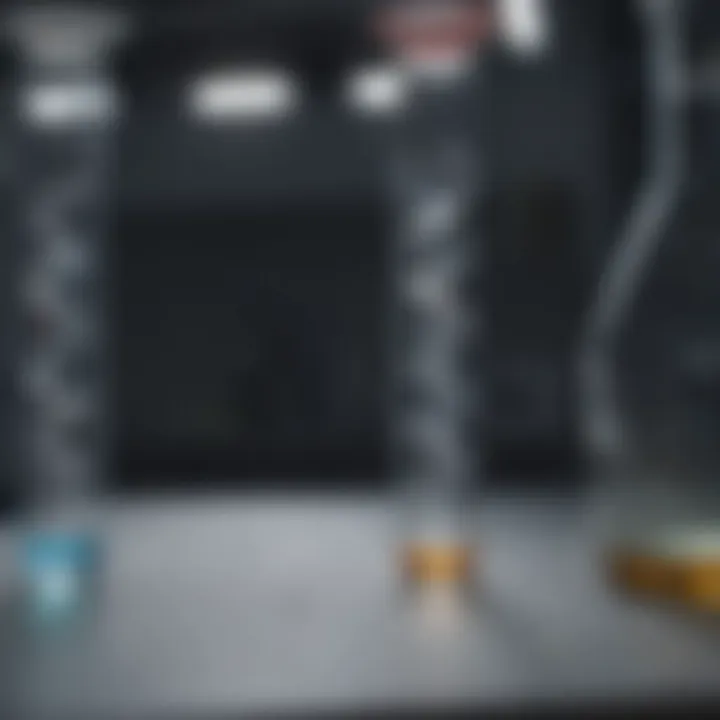
Cell Lysis Techniques
After successful sample preparation, the next crucial step involves breaking down the bacterial cell walls to release DNA. This is known as cell lysis, and it’s essential for accessing the genetic material locked inside.
When it comes to cell lysis techniques, there are several commonly used methods, including:
- Chemical Lysis: This method often utilizes detergents such as SDS that disrupt the lipid bilayer of the bacterial cell membrane. By solubilizing the membrane, the cellular contents, including DNA, are released into solution. Though effective, one must judge the right concentration carefully to avoid damaging the DNA.
- Mechanical Lysis: This involves physically breaking the cell walls using tools such as bead mills or homogenizers. Beads, usually made of glass or ceramic, are mixed with the sample and agitated, which causes the cells to rupture. It’s crucial that the duration and speed of shaking are optimized to prevent shearing of the DNA.
- Enzymatic Lysis: Utilizing enzymes like lysozyme to digest peptidoglycan in the cell wall is another effective approach. Combining this method with others can often yield superior results.
Choosing the appropriate cell lysis technique depends not only on the type of bacteria but also on the downstream applications intended for the extracted DNA.
DNA Purification Methods
Once the cell membrane has been disrupted and DNA released, the next step is purification. Impurities, including proteins, lipids, and other cellular debris, must be removed to ensure high-quality DNA suitable for future applications like PCR or sequencing.
There are various purification methods, each with its advantages:
- Phenol-Chloroform Extraction: A traditional method that separates DNA from proteins and other contaminants using organic solvents. Although effective, this method requires caution due to the hazardous nature of the solvents used.
- Silica-Based Columns: Often utilized in commercial extraction kits, where DNA binds to silica membranes under high salt conditions. Impurities wash away, followed by eluting pure DNA in a low salt buffer. This method is user-friendly with minimal handling.
- Magnetic Bead-Based Purification: An innovative approach where magnetic beads coated with silica or other materials are used for binding DNA. After a simple wash step, the DNA can be eluted. This method is increasingly popular due to its simplicity and efficiency, suitable for automation.
Effectively purifying DNA is paramount. The ultimate aim is to obtain a product free from contaminants that could inhibit downstream processes, thus ensuring accurate results in your research.
Methods of Bacterial DNA Extraction
Understanding the methods of bacterial DNA extraction is vital for researchers and practitioners involved in molecular biology. This phase of study shines a spotlight on how to obtain high-quality DNA from bacterial cells, which is the bedrock of numerous applications. Various techniques can significantly affect the yield and purity of DNA, influencing downstream processes such as cloning, sequencing, or genetic analysis. Each method encompasses unique benefits and considerations, with some tailored to specific types of bacterial strains or research goals. Hence, choosing the right approach can be a game changer in experimental results.
Phenol-Chloroform Method
The Phenol-Chloroform method stands out as a classical technique, frequently highlighted in textbooks for its effectiveness in isolating nucleic acids. This method utilizes a mixture of phenol and chloroform, which separates proteins and other impurities from the DNA. One of the notable advantages of this approach is its ability to produce highly purified DNA, suitable for sensitive applications like PCR amplification.
However, it’s important to handle the reagents with care as they are toxic and can pose health risks if mishandled. The general sequence of steps in this technique includes:
- Sample lysis: Cells are broken open using a lysis buffer.
- Phenol-chloroform extraction: The lysate is mixed with phenol-chloroform, leading to phase separation.
- DNA precipitation: Alcohol is added to precipitate the DNA, which can then be collected by centrifugation.
While effective, the method can sometimes yield lower amounts of DNA from specific bacterial strains, which can be a downside.
Silica-Based Methods
Silica-Based methods have gained popularity in the current landscape of DNA extraction due to their efficiency and ease-of-use. They rely on the property that DNA binds to silica in the presence of chaotropic salts. This allows for the separation of DNA from cellular debris without the need for toxic organic solvents. The typical workflow involves:
- Lysis of the sample, similar to the previous method.
- Addition of a binding buffer containing chaotropic salts to facilitate DNA adherence to silica.
- Washing of the silica to remove contaminants, followed by elution of the DNA with a low-salt buffer or water.
Adopting silica-based methods can minimize risks associated with hazardous chemicals, making it an attractive option for labs prioritizing safety. Though it is widely applicable, some strains may still pose challenges during the process due to cell wall structure, requiring further optimization strategies.
Magnetic Bead Methods
Magnetic Bead methods represent the forefront of DNA extraction technology, offering a rapid and reliable approach. By using magnetic beads coated with specific substances that bind DNA, this method simplifies the separation process and reduces contamination risks. Researchers often favor this method for its flexibility and adaptability to automation, which can lead to high-throughput capabilities.
The typical workflow consists of:
- Sample lysis: As with other methods, the bacterial cells are lysed to release DNA.
- Binding: Magnetic beads are added to the lysate, where DNA attaches to the beads under specific conditions.
- Separation: Magnets are employed to pull the beads out, leaving impurities behind.
- Elution: Finally, the DNA is retrieved by washing the beads with an appropriate elution buffer.
The ability to easily scale the process while ensuring high specificity makes magnetic bead methods a valuable tool in both research and clinical applications.
In summary, when it comes to extracting DNA from bacterial cells, each method carries its own strengths and weaknesses. The choice often hinges on the specific requirements of the project, including the type of bacteria, the desired yield, and purity of DNA, and available laboratory resources.
Considerations for Effective DNA Extraction
When it comes to extracting DNA from bacterial samples, it’s critical to pay attention to the finer details that can make or break the process. Effective DNA extraction is not merely about following a protocol; it’s about understanding the nuances that influence the quality and quantity of the yield. The right strategies can mean the difference between a successful methodology and a failed experiment.
Contamination Prevention Strategies
Contamination is one of the biggest headaches in DNA extraction. Even the smallest of contaminants, be they from the environment or through improperly handled samples, can skew results and jeopardize the integrity of genetic analysis. Thus, having a robust set of contamination prevention strategies is not just advisable; it’s essential.
To minimize contamination risks, consider the following:
- Use of sterile equipment: Always use consumables like pipette tips, tubes, and plates that are certified as sterile.
- Personal hygiene: Lab coats, gloves, and masks can prevent the introduction of extraneous DNA from human sources.
- Clean work environment: Regularly decontaminating surfaces with appropriate disinfectants can significantly reduce the presence of unwanted nucleic acids.
- Dedicated equipment: Where possible, dedicate certain instruments to DNA work only, preventing cross-contamination with non-DNA related activities.
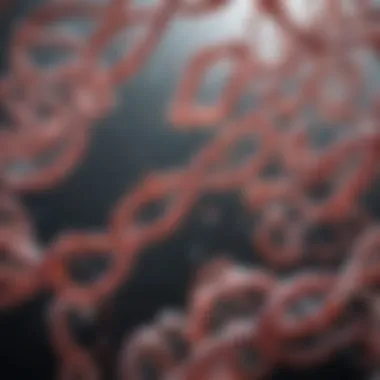
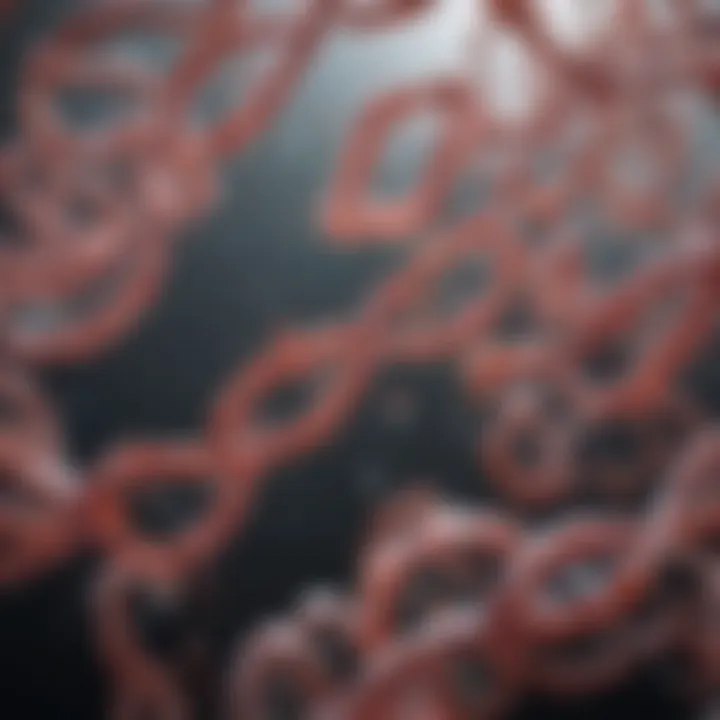
“An ounce of prevention is worth a pound of cure.”
By implementing these strategies, you can create a cleaner extraction environment, thereby enhancing the reliability of your data.
Yield Optimization Techniques
Once contaminant risks are under control, the next step is to focus on maximizing yield. Optimizing DNA yield isn’t just about getting a larger quantity; it’s equally about ensuring that the quality of the DNA extracted is suitable for downstream applications.
Here are a few techniques to consider for yield optimization:
- Adjusting lysis conditions: Different bacterial strains have varying cell wall compositions, thus tailoring the lysis solution can help enhance DNA release.
- Utilizing enzymatic treatments: Employing enzymes such as lysozyme or proteinase K can assist in breaking down cell walls more effectively, leading to improved yield.
- Manipulating incubation times: Sometimes simple tweaks like extending the incubation period during lysis can make a notable difference in the final yield.
- Using binding solutions specific to bacterial DNA: Different methods and kits come with binding solutions designed to maximize yield from bacterial cells, improving purification efficiencies.
With these optimization techniques in mind, any researcher can extract more valuable genetic material from their samples. Effective management of extraction procedures not only increases yield, but also sets the stage for successful analyses and applications that rely on high-quality bacterial DNA.
Quality Assessment of Extracted DNA
A cornerstone in the realm of molecular biology is the quality assessment of extracted DNA. This pivotal step determines not just the usability of the DNA samples but also the reliability of downstream applications. Poor quality DNA can lead to skewed results in experiments ranging from sequencing to cloning, hence assessing the integrity and purity of the extracted material cannot be stressed enough. Researchers must dig deep into the nuances of DNA quality to ensure that their findings are both accurate and impactful.
Agarose Gel Electrophoresis
Agarose gel electrophoresis stands tall as a go-to method for assessing DNA quality. This process involves the separation of DNA fragments based on size through a porous gel matrix. When samples are loaded into the gel, an electric current is applied. DNA, being negatively charged due to its phosphate backbone, migrates towards the positive electrode.
What makes agarose gel electrophoresis particularly useful is its ability to not just show how much DNA is present but also to visualize the size distribution of the fragments. After running the gel, each DNA sample produces bands at various levels depending on size, which can be sensitive indicators of quality.
To interpret the results effectively, one must pay close attention to:
- Band definition: Clear, distinct bands suggest high-quality DNA, while smeared or faint bands could indicate degradation or contamination.
- Size markers: Including a molecular weight ladder alongside samples helps in estimating the size of DNA fragments, enabling better analysis of results.
- Yield assessment: The thickness of bands can provide a rough measure of the DNA yield, further guiding any necessary adjustments in the extraction process.
In this way, agarose gel electrophoresis serves not only as a quality control tool but also a learning platform for researchers about the intricacies of their samples.
Spectrophotometric Analysis
Another widely used method for DNA quality assessment is spectrophotometric analysis. This technique employs a spectrophotometer to measure the absorbance of DNA solutions at specific wavelengths, primarily 260 nm and 280 nm. The readings at these wavelengths give insight into the purity and concentration of the DNA extracted.
The beauty of this method lies in its simplicity and speed. By calculating the ratio of absorbance at 260 nm to that at 280 nm, researchers can make quick assessments:
- A260/A280 Ratio: A typical value for pure DNA should hover around 1.8. Values much lower hint at contamination by proteins or phenol, while higher ratios could suggest contamination by RNA.
- Concentration Calculation: The absorbance at 260 nm can be translated directly into concentration, allowing researchers to work out precise amounts for further experiments.
What to keep in mind is the sensitivity of spectrophotometry to contaminants that may not necessarily interfere with electrophoresis results, making it a complementary method rather than a standalone solution.
“The combination of agarose gel electrophoresis and spectrophotometric analysis can provide a robust framework for quality assurance in bacterial DNA extraction protocols.”
By intertwining these two methods, researchers can achieve a comprehensive overview of their DNA's health state, ensuring that every subsequent step in their projects stands on solid ground.
Challenges in Bacterial DNA Extraction
Bacterial DNA extraction is not always a walk in the park. Various factors can impede the smooth sailing through the extraction process, leading to inconsistent results and inefficiencies. Highlighting these challenges is crucial as it equips researchers with the awareness and strategies to handle potential issues effectively. By subscribing to this comprehensive overview, individuals can enhance their approaches when faced with hurdles, promoting a more streamlined and successful extraction experience.
Inhibitory Substances in Sample
When dealing with bacterial samples, one of the most significant headaches comes from inhibitory substances that can wreak havoc during the DNA extraction process. As these samples often contain proteins, lipids, and polysaccharides, their presence can dramatically hinder enzymatic reactions needed for breaking down cellular components.
Some common inhibitors include:
- Proteins: These can interfere with enzyme activity, affecting the yield and quality of extracted DNA.
- Humic acids: Soil-sourced bacteria often contain these dark brown substances. They have been known to inhibit PCR reactions, thereby complicating downstream applications.
- Ethanol and other solvents: Residual solvents can affect DNA purity, leading to complications in analysis.
The presence of such inhibitors can slow down procedures or even render the extracted DNA unusable. As a workaround, researchers often employ methods like sample dilution, additional purification steps, or the use of specialized inhibitor removal kits designed to cleanse samples of these troublesome substances. Effective treatment ensures that the extracted DNA is both pure and viable for further research.
Variability Among Bacterial Strains
Another consideration that can turn extraction into a bit of a puzzle is the inherent variability among different bacterial strains. Not all bacteria are created equal—some possess stubborn cell wall structures or unique extracellular components that can complicate extraction efforts.
Key factors leading to variability include:
- Cell wall structure: Gram-negative bacteria have outer membranes that can resist lysis unless specific lysis methods are utilized, contrasting with the simpler walls of Gram-positive species.
- Growth conditions: Bacteria grown under different environmental or nutrient conditions can express distinct properties, which may lead to variations in DNA yield and quality.
- Strain characteristics: Even within the same species, different strains can exhibit unique genetic traits or metabolic capabilities that influence their response to extraction techniques.
Addressing this variability often requires tailoring extraction protocols to align with the specific characteristics of the bacterial strain being studied. Opting for strain-specific techniques not only enhances the efficiency of the extraction process but also ensures that the quality of the extracted DNA meets necessary standards for research or diagnostic applications.
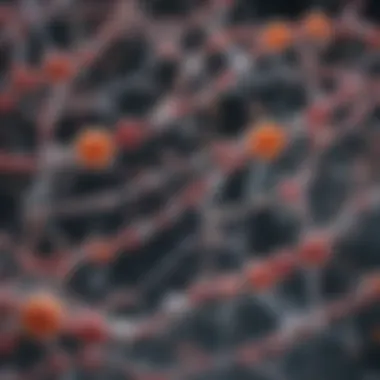
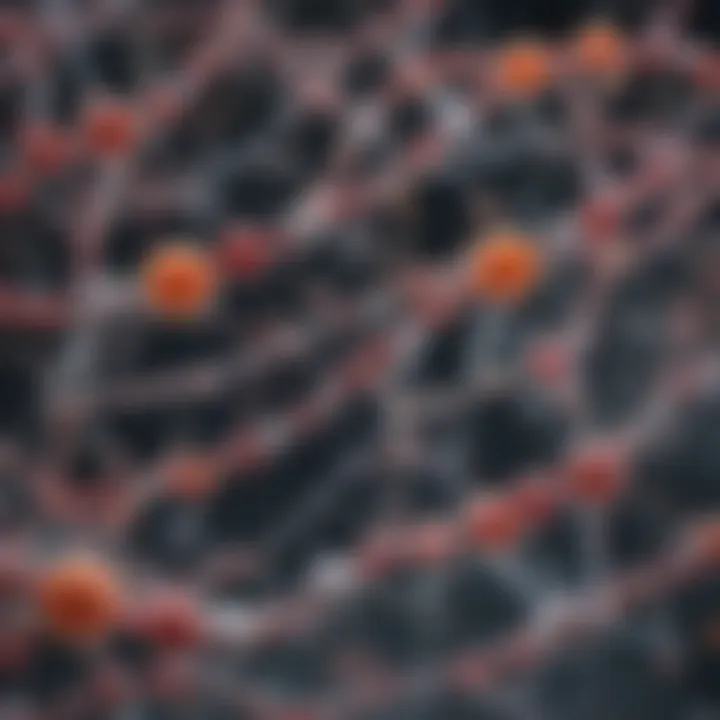
"Understanding these challenges is half the battle in successful bacterial DNA extraction; it empowers researchers to adapt and innovate.”
In summary, by being acutely aware of inhibitory substances in samples and the variability among bacterial strains, researchers can devise more effective strategies in their DNA extraction endeavors. By tackling these challenges head-on, the path to reliable outcomes becomes clearer and more attainable.
Applications of Extracted Bacterial DNA
Understanding the applications of extracted bacterial DNA is crucial, not only for molecular biology but also for various fields including medicine, agriculture, and environmental science. The ability to extract and analyze bacterial DNA has unlocked many doors, providing insights that were previously out of reach. This section will delve into the primary applications, focusing on the specific roles that bacterial DNA plays in molecular diagnostics and genetic engineering.
Molecular Diagnostics
Molecular diagnostics leverages the power of extracted bacterial DNA to identify pathogens swiftly and accurately. Traditional methods of diagnosing infectious diseases can be slow and cumbersome, often leading to misdiagnosis or delayed treatment. Molecular diagnostics, on the other hand, offers a solution by detecting the genetic material of bacteria directly.
Benefits of Molecular Diagnostics:
- Rapid Identification: Extracted bacterial DNA enables quick identification of pathogens. Techniques like polymerase chain reaction (PCR) use this DNA to amplify specific sequences, allowing researchers to ascertain the presence of bacteria in a matter of hours.
- Higher Sensitivity: Compared to culture-based methods, DNA extraction allows for the detection of very low levels of bacterial DNA, making it possible to diagnose infections even in cases where bacteria are not easily cultivated in a lab.
- Specificity: The genetic information in extracted DNA can be tailored to identify specific strains of bacteria, thereby facilitating more precise treatments and management of diseases.
Due to these advantages, molecular diagnostics is a cornerstone in fields such as clinical microbiology. For example, in cases of suspected tuberculosis, extracting and analyzing the DNA from a sample can lead to quicker confirmation of the disease, allowing for timely intervention.
Genetic Engineering and Biotechnology
Bacterial DNA also plays a pivotal role in genetic engineering and biotechnology, where it serves as a vital building block for various applications. By extracting DNA from bacteria, scientists can explore its genetic material, enabling the alteration or enhancement of biological functions.
Key Applications in Genetic Engineering:
- Recombinant DNA Technology: Extracted DNA can be cut and recombined with other DNA strands, leading to the development of genetically modified organisms (GMOs). This has significant implications for agriculture, where traits like drought resistance or pest tolerance can be introduced into crops, enhancing food security.
- Production of Biopharmaceuticals: Bacterial DNA is frequently used to produce proteins, including insulin and growth hormones. By inserting human genes into bacterial plasmids, scientists can cultivate bacteria that produce these essential hormones in bulk, paving the way for therapeutic applications.
- Gene Therapy: Research in gene therapy often involves using bacterial DNA to deliver therapeutic genes to patients, offering potential cures for genetic disorders.
In essence, the extraction of bacterial DNA is a foundational practice that fuels innovation in molecular diagnostics and genetic engineering. As technologies evolve and new techniques emerge, the potential applications will only continue to expand, unlocking further possibilities for research and practical use in various industries.
"The extraction and understanding of bacterial DNA is not just an academic pursuit; it's a gateway to revolutionary advancements that can change the course of healthcare and environmental management."
In summary, the applications of extracted bacterial DNA extend well beyond traditional boundaries, offering robust tools for diagnostics and paving the way for genetic advancements that might one day transform industries and enhance our understanding of life itself.
Future Directions in Bacterial DNA Extraction Techniques
The landscape of bacterial DNA extraction is poised for transformative shifts, with innovations emerging that may redefine existing protocols and methodologies. This section aims to illuminate the avenues that researchers might pursue to enhance and refine the extraction of bacterial DNA, elucidating the importance of these developments in advancing scientific inquiry.
Emerging Technologies
Recent years have ushered in a wave of new technologies that promise to simplify and enhance the efficiency of bacterial DNA extraction. One area of notable advancement is the automation of the extraction process. Automated platforms can manage repetitive tasks, markedly increasing throughput and reducing the risk of human error. This not only streamlines workflows in high-volume laboratories but also ensures improved consistency in the quality of extracted DNA.
Another promising technology involves the integration of microfluidics. By manipulating small volumes of liquids on chips, microfluidics allows for faster reactions and more efficient resource usage. Imagine performing several extraction protocols simultaneously — this capability can lead to significant time savings and reduced reagent costs.
Moreover, advancements in nano-materials are paving the way for highly effective and selective extraction methods. For instance, the use of magnetic nanoparticles can enable targeted binding of bacterial DNA, facilitating easier separation and purification from the cellular milieu. This innovative approach may offer researchers not just speed but also improved yields and cleaner DNA extracts, critical for downstream applications like sequencing.
Potential Improvements in Protocols
While existing protocols for bacterial DNA extraction have laid a solid foundation, there's room for evolution to enhance their effectiveness. One approach is optimizing lysis buffers. The exact composition of buffers can vary widely among bacterial strains due to differences in cell wall structures. Tailoring these buffers to match specific bacterial species can lead to more effective cell lysis, improving the yield of DNA.
Additionally, researchers might explore the synergistic effects of combining various extraction methods. For example, a two-step approach that first utilizes enzymatic lysis followed by a silica-based extraction could capitalize on the strengths of both methods, yielding high-quality DNA. This would reflect a growing trend of hybrid methodologies, particularly relevant in the wake of the increasing diversity found in bacterial communities.
"Future advancements in bacterial DNA extraction are likely to spur significant progress in genomics and microbiome research."
Moreover, developing user-friendly kits that can accommodate less experienced users is another crucial improvement. Simplifying protocols without sacrificing quality can democratize access to bacterial DNA extraction, making it feasible not only in research labs but also in educational settings and smaller institutions.
Culmination
In an ever-evolving field like molecular biology, understanding the intricacies of bacterial DNA extraction isn't just a niche interest—it's a vital cornerstone for numerous scientific advancements. This article has provided a thorough exploration of the protocols, emphasizing their significance across various applications. The steps and techniques discussed are not mere laboratory practices; they embody the foundational processes that enable researchers to delve into the genetic blueprints of bacteria, unlocking potential innovations in medicine, biotechnology, and environmental science.
Summary of Key Points
- Importance of Protocols: The extraction protocols serve as bedrock for downstream applications, including cloning, sequencing, and analysis.
- Diverse Methods: We examined several extraction methods—from classic techniques like the phenol-chloroform method to more modern approaches such as silica-based and magnetic bead methods.
- Quality Assessments: Discussed various quality assessment techniques, including spectrophotometric analysis and agarose gel electrophoresis, which are essential in ensuring the integrity of the extracted DNA.
- Challenges and Solutions: Addressed common challenges faced during DNA extraction, such as inhibitory substances and strain variability, and provided innovative strategies to enhance efficiency.
- Future Directions: The exploration of emerging technologies presents exciting possibilities that could revolutionize current protocols and practices.
Implications for Future Research
The implications of enhanced bacterial DNA extraction protocols are profound. As scientists continue pushing the boundaries of genetic research, optimized extraction methods will play a crucial role in expanding our understanding of microbial functions and interactions. Enhanced protocols can lead to better yields and purities, which are paramount for high-accuracy studies, including metagenomics, pathogen detection, and synthetic biology applications.
Moreover, the insights gained from continued refinement in these techniques may stimulate interdisciplinary collaborations, blending microbiology with fields such as bioinformatics, environmental science, and pharmacology. As researchers grapple with complex biological systems, advancements in bacterial DNA extraction will be pivotal in enabling innovations that drive research forward, fostering a deeper understanding of the microbial world.
"Bacterial DNA extraction isn't just about getting DNA; it's about opening doors to new scientific realms."
The future indeed looks bright as we expand our capabilities and methodologies in the realm of bacterial DNA extraction, promising a richer and more nuanced understanding of life at the microscopic level.