Astrocyte Cell Lines in Neuroscience Research
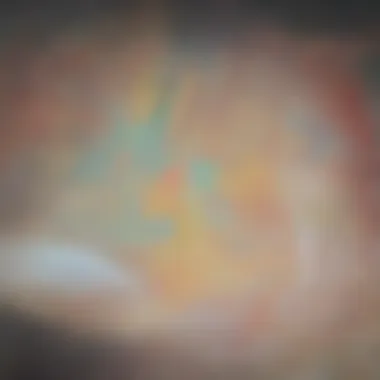
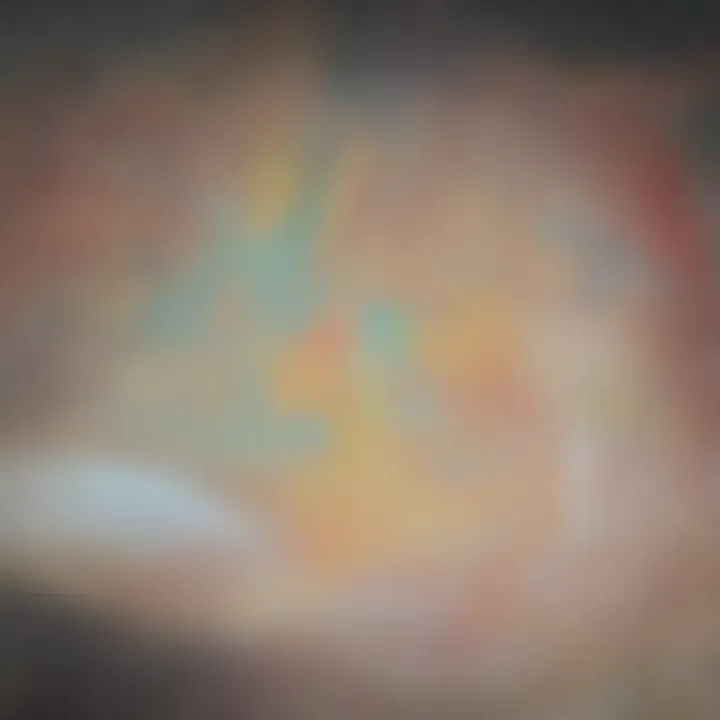
Intro
Astrocyte cell lines are emerging as critical tools in the field of neuroscience. They serve not just as model systems but also as platforms for understanding the intricate relationships between glial cells and neuronal activity. These cell lines are pivotal in the study of various neural conditions, allowing researchers to investigate the underlying mechanisms of brain health and disease. The robust nature of these cell lines, combined with their utility in experimental frameworks, highlights their growing significance in contemporary research.
As we delve into the world of astrocyte cell lines, it becomes clear that their characteristics and development offer rich insights into glial cell function. This section will outline the key findings regarding astrocyte cell lines, their implications, and the potential applications for further research, ultimately fostering a deeper understanding of their role in both basic and applied neuroscience.
Key Findings
Summary of the main results
Astrocyte cell lines have been found to exhibit several key characteristics important for neuroscience research:
- Supportive Role: Astrocytes provide metabolic support to neurons, which is critical for neuronal survival and function.
- Signaling Function: These cells participate in neurotransmitter uptake and release, contributing to synaptic function and plasticity.
- Pathological Insights: They offer windows into neurodegenerative diseases, helping understand conditions such as Alzheimer’s and multiple sclerosis.
Recent studies reveal that astrocyte cell lines can replicate certain in vivo conditions when cultured under specific environments. This adaptability enhances their value in experimental setups aimed at studying neuroinflammation and apoptotic mechanisms.
Significance of findings within the scientific community
The insights gained from astrocyte cell lines underscore their significance in several domains of neuroscience. They facilitate:
- Drug Testing: By modeling disease conditions, researchers can test the efficacy of new therapeutic approaches, which is crucial for developing effective treatments for various neurological disorders.
- Biomarker Discovery: The ability to culture astrocyte lines consistently allows for the identification of potential biomarkers for diseases, improving disease diagnostics.
- Understanding Cellular Interactions: Research into astrocytes clarifies their interactions with other cell types in the brain, illuminating the complexities of neurobiology.
"Astrocyte cell lines are more than just models; they represent a cornerstone in unveiling the mysteries of brain functions and disorders."
Implications of the Research
Applications of findings in real-world scenarios
Astrocyte cell lines have various practical applications. For instance, they can:
- Aid in the development of neuroprotective strategies against brain injuries.
- Facilitate the synthesis of neuroinflammatory mediators, thereby enhancing the understanding of related disorders.
- Serve as platforms for exploring the pharmacological properties of potential drugs.
Potential impact on future research directions
The potential impact of astrocyte cell lines is broad and profound. Future research may involve:
- Increased focus on genetic modifications to study specific functions of astrocytes in neurodegeneration.
- Development of three-dimensional models that better represent the in vivo environment.
- Exploration of astrocyte involvement in psychiatric disorders, providing a more comprehensive picture of brain pathology.
As we continue to investigate the genetic and molecular basis of astrocyte function, we can expect a surge in knowledge that will illuminate the pathophysiology of various neurological conditions. The future of neuroscience relies on the insights provided by astrocyte cell lines, promoting a deeper understanding of both brain health and dysfunction.
Preface to Astrocytes
Astrocytes are a critical component of the central nervous system, with a significant role in supporting neuronal functions and maintaining homeostasis. The importance of understanding astrocytes in neuroscience cannot be understated. In this article, we will explore their roles, functions, and how they relate to the broader scope of neurobiology. With advances in research technologies, astrocytes have garnered attention due to their involvement in various neurological conditions, including neurodegenerative diseases. Their complex interactions with neurons and other glial cells highlight the need for deeper exploration.
Definition and Role
Astrocytes are star-shaped glial cells found in the brain and spinal cord. They are the most abundant cell type in the human brain. The primary function of astrocytes is to provide structural support to neurons. They play a role in several processes essential for neuronal health, such as regulating blood flow, providing nutrients, and maintaining the blood-brain barrier.
In addition to these supportive roles, astrocytes are active participants in neurotransmission. They can take up neurotransmitters from the synaptic cleft, recycling these chemical messengers and thus influencing synaptic activity. Astrocytes also release gliotransmitters, which modulate synaptic transmission and can impact neuronal signaling. The dynamic nature of astrocytic functions indicates their broader implications in neural communication and brain function.
Astrocytic Functions in the Central Nervous System
The functions of astrocytes extend well beyond mere support roles. They are involved in various physiological and pathological processes, making them integral to understanding brain function. Some critical functions include:
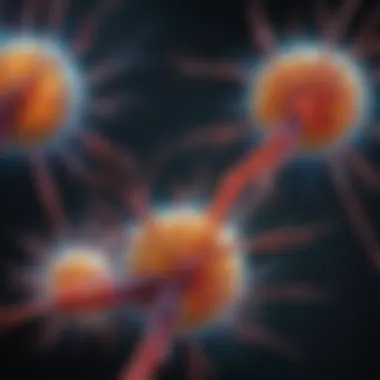
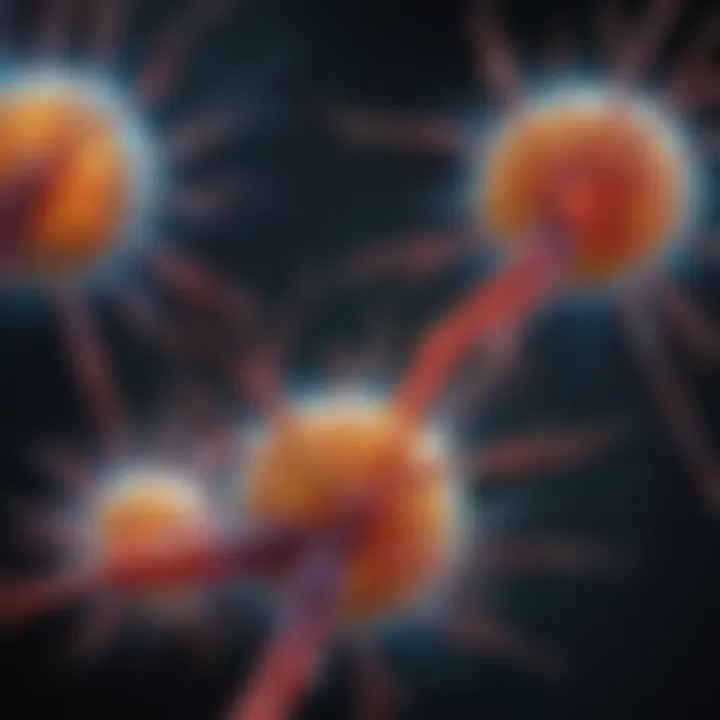
- Neurotransmitter Regulation: Astrocytes modulate the levels of neurotransmitters in the synaptic cleft, ensuring balance in neuronal signaling.
- Ion Homeostasis: They help maintain ion balance, particularly potassium ions, critical for maintaining the resting potential of neurons.
- Support of Neurogenesis: In specific contexts, astrocytes can promote the generation of new neurons in the brain.
- Response to Injury: Astrocytes are part of the brain's response to injury. They can proliferate and form a glial scar, which can both help and hinder recovery.
"Astrocytes are fundamentally entwined with neuronal health and disease; their diverse roles make them an area of interest for researchers aiming to understand complex brain functions."
The intricacies of astrocytic activity delineate their importance in both preserving and disrupting brain function. Understanding these dynamics provides a pathway to unraveling their implications in various neurological and psychiatric disorders.
Overview of Astrocyte Cell Lines
Astrocyte cell lines are crucial components in neuroscience research. They allow scientists to explore the functions and effects of astrocytes in a controlled environment. With the growing understanding that astrocytes are not merely supportive cells but play active roles in neurotransmission, homeostasis, and neuroprotection, the significance of studying these cell lines has surged. The ability to manipulate these cells, either genetically or chemically, provides powerful insights into their contributions to both healthy and diseased states of the central nervous system.
Research utilizing astrocyte cell lines offers several benefits. These cells can be cultured and maintained under laboratory conditions, facilitating reproducibility and consistency across experiments. They provide researchers with tools to examine the cellular and molecular mechanisms that underlie various neurological conditions. Additionally, astrocyte cell lines can be utilized for high-throughput screening of drugs, significantly accelerating the process of drug discovery and toxicity testing.
However, there are important considerations when working with astrocyte cell lines. Their characteristics can differ from primary astrocytes found in living organisms, which can lead to variations in behavior and function. Understanding these differences is essential for interpreting results accurately. The evolution of astrocyte cell lines, stemming from various sources and isolated conditions, also adds an inherent complexity to their application.
Characteristics of Astrocyte Cell Lines
Astrocyte cell lines exhibit specific traits that set them apart from other cell types. These characteristics can influence how researchers utilize these cells for experimental purposes. Some important features include:
- Morphological Properties: Astrocyte cell lines typically display a stellate shape, which is indicative of their in vivo counterparts. This shape is essential for their functionality, allowing them to interact with neurons and other glial cells.
- Gene Expression Profiles: The expression of astrocyte-specific markers, such as glial fibrillary acidic protein (GFAP), helps confirm the identity of these cells. Variability in expression levels can occur based on the cell line used, thus impacting experimental outcomes.
- Functional Capabilities: Astrocyte cell lines retain some preferential functionalities of primary astrocytes, including the uptake of neurotransmitters and release of gliotransmitters. The extent of these capabilities may vary across different cell lines, which researchers need to account for in their analyses.
Types of Astrocyte Cell Lines
There are various types of astrocyte cell lines derived from different species and regions of the brain, each serving distinct research needs. Some notable examples include:
- C8-B4: This cell line, derived from rat spinal cord astrocytes, has been instrumental in studying spinal cord injuries and neurodegenerative diseases.
- SVG N: Originating from human fetal tissue, SVG N cells are often used for studies on human astrocytic functions and their interactions with neurons in a human-specific context.
- U251: A human glioblastoma cell line, U251 is often utilized in cancer research to understand tumor biology and potential therapeutic targets relating to astrocytic cells.
Methods of Astrocyte Cell Line Isolation
The process of isolating astrocyte cell lines is very important in neuroscience research. It allows researchers to study astrocytes in a controlled environment, helping to provide insights into their functions and roles in various neurological conditions. Understanding these methods is vital as they directly affect the reliability and applicability of the results in experimental settings.
The techniques used to isolate astrocyte cell lines can significantly impact their characteristics and behaviors. Therefore, choosing the right isolation method is crucial for researchers aiming to develop relevant models for in vitro studies. This section discusses two principal methods: primary culture techniques and cell line development from those primary cultures.
Primary Culture Techniques
Primary culture techniques refer to the initial methods used to extract and grow astrocytes from brain tissue. This is usually done through mechanical or enzymatic dissociation. Here are the most common methods of isolation:
- Mechanical Dissociation: Tissue samples are perfused and then physically minced to separate the astrocytes. This can be followed by filtering the suspension to remove large debris.
- Enzymatic Dissociation: Enzymes such as trypsin or collagenase are used to break down extracellular matrix proteins. It often results in a higher yield of viable cells compared to mechanical dissociation alone.
These techniques typically yield mixed cell populations that can include not only astrocytes but also other glial cells and neurons. Careful handling is crucial to maintain cell viability. The primary cultures often exhibit diverse characteristics that reflect the complex environment of the brain.
Cell Line Development from Primary Cultures
After establishing primary cultures, the next step is to develop these into stable astrocyte cell lines. This process usually involves the following:
- Passaging: The primary cultures are periodically split and transferred to new culture vessels to expand the cell population. This allows the growth conditions to be optimized for astrocytes.
- Immortalization: To create a cell line, researchers may introduce specific genes or viral elements that extend the lifespan of the astrocytes. This is often done using retroviral transduction or the introduction of oncogenes. Successful immortalization leads to cell lines that can be passaged indefinitely while retaining critical functions.
- Characterization: The cell lines must undergo thorough testing to ensure they maintain astrocytic properties and do not lose their identities over multiple passages.
While developing astrocyte cell lines provides a renewable source of cells for research, it is crucial to remember that these cell lines may not entirely reflect the in vivo environment. Researchers must take this limitation into account when designing studies and interpreting results.
Cell line development has revolutionized neuroscience research, enabling extensive studies that were not feasible with primary cultures alone.
Applications of Astrocyte Cell Lines
Astrocyte cell lines are crucial in neuroscience research. Their applications provide valuable insights into various aspects of neurobiology. By studying these cells, researchers can dissect complex neural environments. Understanding astrocytic roles helps illuminate mechanisms behind brain function and disease.
In vitro Studies of Neurobiology
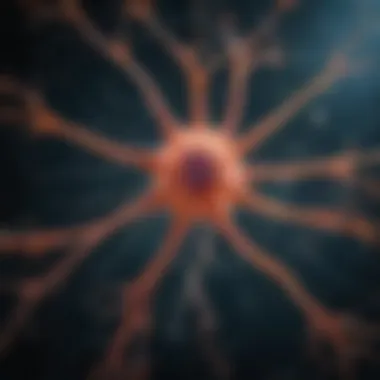
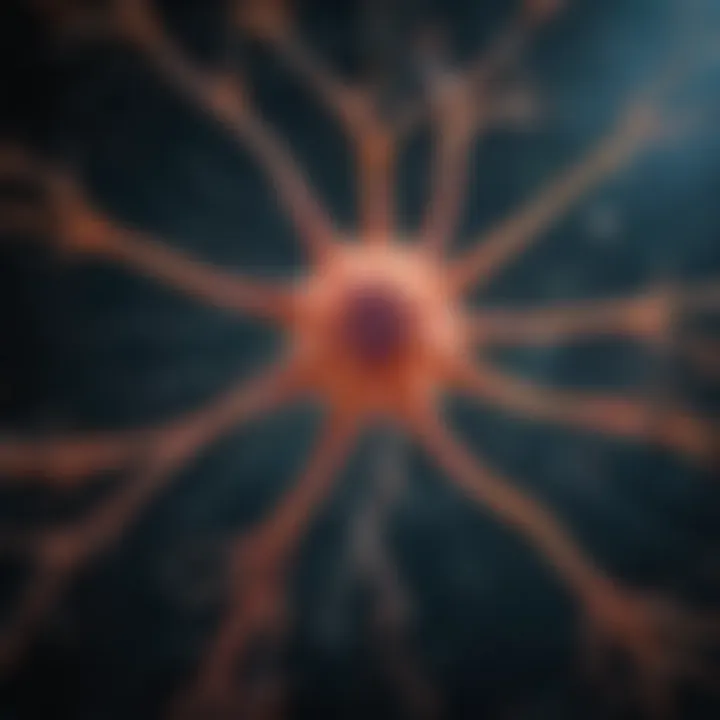
Astrocyte cell lines facilitate in vitro studies, offering a controlled environment to observe cellular behavior. Researchers utilize these lines to understand fundamental processes such as cell signaling and metabolic functions. The ability to manipulate astrocytes in a laboratory setting allows for detailed investigations of their interactions with neurons and other glial cells.
These studies can reveal how astrocytes respond to different stimuli, including neuroinflammatory signals and excitotoxic agents. Furthermore, insights gained through in vitro examinations support the exploration of synaptic modulation, blood-brain barrier integrity, and the overall homeostasis of the central nervous system.
Drug Development and Toxicity Testing
Astrocyte cell lines are instrumental in drug development, particularly when assessing new therapeutic compounds. These cell lines serve as models to evaluate the efficacy and safety of potential drugs affecting glial cells. Understanding drug interactions with astrocytes can predict potential neurotoxic effects and possible therapeutic benefits.
Moreover, astrocytes have a unique role in metabolic support and detoxification. Consequently, toxicity testing using such cell lines provides essential data regarding the implications of drug exposure. This information helps refine drug formulations before clinical trials, promoting better patient safety and treatment outcomes.
Modeling Neurodegenerative Diseases
Astrocyte cell lines play a significant role in modeling neurodegenerative diseases like Alzheimer’s and Parkinson’s. By replicating these diseases in vitro, researchers can study pathophysiological mechanisms relevant to astrocytic dysfunction. This research avenue can highlight how astrocytes influence neuroinflammation, synaptic loss, and amyloid-beta plaque accumulation, which are pivotal in Alzheimer's disease progression.
Notably, these models can help in screening for drugs that may halt or reverse the effects of neurodegeneration. By understanding astrocyte behavior in disease contexts, investigators can identify novel biomarkers and therapeutic targets. This contribution is vital for advancing our comprehension of neurological disorders and developing effective treatments.
Astrocytes in Disease Models
Astrocytes have gained considerable attention in the context of disease models, particularly due to their multifaceted roles in the central nervous system (CNS). The significance of employing astrocytic cell lines in these models lies in their ability to mimic the complex interactions occurring in vivo. By studying astrocytes within various disease contexts, researchers can uncover insights into pathology, therapeutic targets, and potential treatment options. Such a focus allows for deeper exploration of neurodegenerative conditions and their mechanisms of action.
Role in Alzheimer’s Disease Models
Alzheimer's disease is characterized by the accumulation of amyloid-beta plaques and tau protein tangles. Astrocytes contribute to both the maintenance and disruption of homeostasis in this disease context. In vitro models utilizing astrocyte cell lines provide an excellent platform to study the cellular responses to these pathology markers. These studies have revealed that astrocytes can influence amyloid-beta metabolism. They do this through the uptake and clearance mechanisms. Furthermore, activated astrocytes can release pro-inflammatory cytokines, playing a dual role in exacerbating or mitigating neurodegeneration.
Research identifying astrocyte dysfunction in Alzheimer's models underscores the necessity of these cell lines in comprehending disease dynamics. Specifically, astrocytes may contribute to synaptic dysfunction and neuronal loss, highlighting their potential as therapeutic targets. This understanding encourages the development of treatments aimed at restoring normal astrocyte functions or preventing their detrimental effects.
Implications in Multiple Sclerosis Research
Multiple sclerosis (MS) is an autoimmune disorder marked by the degradation of myelin sheaths surrounding neurons. The participation of astrocytes is crucial in MS research, as they become reactive in response to demyelination and inflammatory processes. Utilizing astrocytic cell lines for modeling this disease enables an examination of their role in the inflammatory milieu and the establishment of gliosis.
Astrocytes can secrete both neuroprotective and neurotoxic factors during disease progression. By investigating the behavior of these cells in vitro, researchers can delineate pathways that may lead to either beneficial or harmful outcomes. The dual nature of astrocytic responses makes them a focal point for understanding the progression of MS. Therapies targeting reactive astrocytes could potentially alter the disease course, making the exploration of these cell lines a significant step forward.
Key Insight: By modeling diseases such as Alzheimer's and multiple sclerosis with astrocyte cell lines, researchers unveil the critical roles these cells play in brain health and pathology.
The implications of astrocytes in disease models present valuable opportunities for advancing therapeutic strategies that may eventually lead to improved patient outcomes.
Cell Line Limitations and Challenges
The exploration of astrocyte cell lines in neuroscience research is crucial not only for their applications but also for recognizing their limitations and challenges. Understanding these aspects ensures researchers can interpret findings accurately and optimize the utility of these cell lines in experimental setups. Despite their advantages, astrocyte cell lines can present notable issues that can significantly affect research outcomes. This section delves into two primary challenges: maintaining relevance to in vivo conditions and addressing genetic and phenotypic variability.
Maintaining Relevance to In Vivo Conditions
Astrocyte cell lines often diverge from the natural brain environment in which they originated. This divergence impacts their physiological characteristics and functional relevance. While cell lines provide controlled environments for studies, the complexity of in vivo conditions is not fully replicated. For instance, astrocytes in the brain interact with neurons, blood vessels, and other cell types, creating a dynamic microenvironment. In cell cultures, these interactions are often simplified or entirely absent.
Researchers need to be aware that results obtained from astrocyte cell lines may not always translate effectively to in vivo situations. Factors such as altered signaling pathways, changes in metabolic processes, and even differences in responsiveness to pharmacological agents can arise. It is essential to consider these factors when designing experiments and interpreting results.
Such limitations highlight the importance of complementary approaches. Utilizing primary astrocyte cultures or organoid models alongside established cell lines can provide a more comprehensive understanding of astrocytic behavior.
Genetic and Phenotypic Variability
Another significant challenge in using astrocyte cell lines lies within genetic and phenotypic variability. Genetic mutations, epigenetic changes, and environmental influences can lead to substantial differences among cell lines, even those derived from the same origin. This can affect experimental reproducibility and the reliability of findings.
Variability may manifest in different ways:
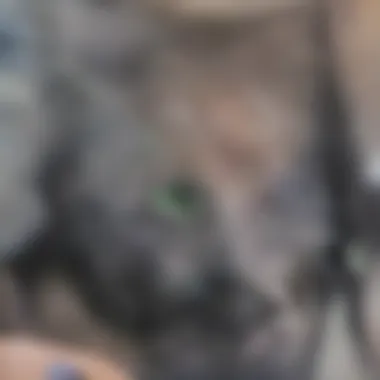
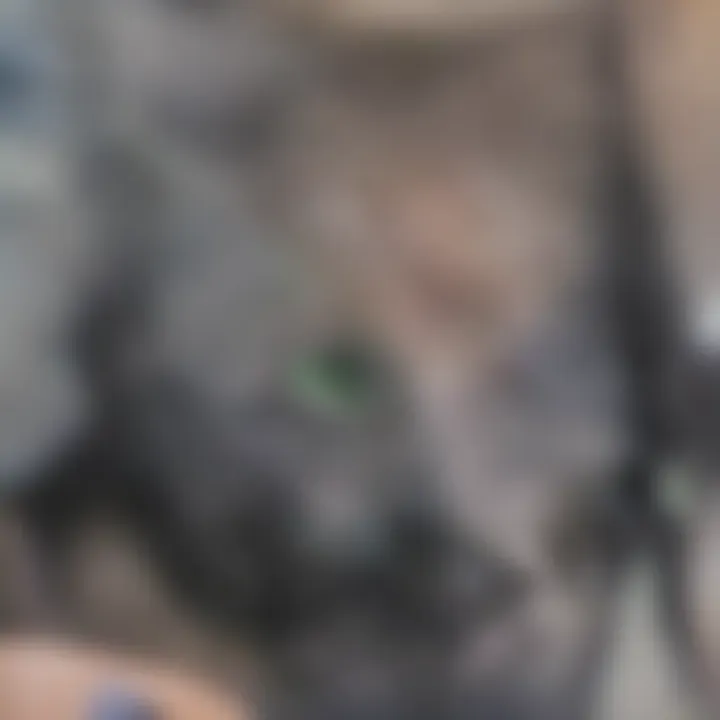
- Differing growth rates. Some cell lines may proliferate faster than others, which can affect experimental timelines.
- Distinct responses to stimuli. Different astrocyte cell lines might react differently to the same neurotransmitters or drugs, leading to inconsistent results.
- Altered expression profiles. Gene expression can vary widely, impacting studies of function and disease modeling.
Researchers must account for this variability by validating their results across multiple astrocyte cell lines and considering genetic background when interpreting data. Employing techniques like single-cell RNA sequencing may also help in identifying subpopulations of cells within the lines that might exhibit divergent phenotypes.
In summary, while astrocyte cell lines are invaluable tools in neuroscience research, their limitations and challenges require careful consideration. Maintaining physiological relevance and addressing genetic and phenotypic variability are critical for ensuring the integrity and applicability of research findings.
"Awareness of the limitations of astrocyte cell lines ensures more reliable and meaningful research outcomes."
Combining the insights from these discussions can improve experimental design and foster advancements in our understanding of astrocytic functions in health and disease.
Future Directions in Astrocyte Research
The study of astrocytes is continuously evolving. Future directions in this research area are critical for advancing our understanding of brain health and disease. New approaches can potentially transform how researchers explore the roles of astrocytes in neurological conditions. This section will highlight important elements and considerations in this field.
Emerging Technologies in Astrocyte Study
Emerging technologies are paving the way for breakthroughs in astrocyte research. Techniques such as single-cell RNA sequencing are allowing researchers to dissect the cellular behavior of astrocytes. These technologies help in understanding the heterogeneity within astrocyte populations.
- Optogenetics provides precise control over astrocytic activity, aiding in the study of their role in neural circuits.
- CRISPR-Cas9 gene editing is facilitating the manipulation of specific genes in astrocytes, helping to uncover genetic contributions to various diseases.
By employing these advanced techniques, scientists can examine astrocytic responses in real-time and in physiological environments. This shift might uncover new therapeutic targets and strategies for treatment.
Key Considerations:
- Ethical implications of genetic manipulation should be carefully evaluated.
- The choice of technology must match the research question to ensure reliable outcomes.
Potential for Clinical Translation
Clinical translation of astrocyte research holds promising potential. Insights gained from laboratory studies could lead to innovative treatments for neurological disorders. For instance, understanding how astrocytes respond to injury may inform approaches for enhancing recovery after stroke.
Several factors influence the path to clinical application:
- Scalability of cell culture methods to produce sufficient astrocyte lines for therapy.
- Safety and efficacy in animal models before initiating human trials.
- Collaboration between research institutions and pharmaceutical companies to facilitate the transition from bench to bedside.
"The complexity of brain functions means that any treatment derived from astrocyte research will likely be multifaceted."
This statement underscores the importance of a multidisciplinary approach.
Closure
The conclusion of this article emphasizes the crucial role of astrocyte cell lines in advancing neuroscience research. By integrating insights from various subfields, this section synthesizes the knowledge acquired throughout the article, highlighting how these cell lines serve as a bridge between fundamental discoveries and practical applications.
In summary, astrocyte cell lines enable scientists to investigate complex neuronal interactions and cellular mechanisms in controlled environments. They provide a platform for drug testing and reveal how astrocytes contribute to neurodegenerative diseases such as Alzheimer’s and multiple sclerosis. Through these models, researchers can better understand the pathophysiology of neurological disorders.
Summary of Key Insights
Astrocyte cell lines offer multiple insights into brain health and disease. Some key points include:
- Versatility: These cell lines are adaptable for various experimental setups, including studies on neuroinflammation and synaptic physiology.
- Modeling Disease: They provide crucial information about astrocytic involvement in diseases like Alzheimer's and how they might affect therapeutic outcomes.
- Research Tool: Researchers can manipulate these cell lines genetically, allowing for deeper investigation into specific cellular functions and pathology.
Thus, astrocyte cell lines have become indispensable in current neuroscientific research, helping to bridge the gap between basic science and clinical application.
The Importance of Ongoing Research
Continuing to refine and explore astrocyte cell lines is essential. The evolution of techniques in this field offers promising avenues for discovery. Ongoing research endeavors should aim at:
- Improving Models: Enhancement of existing cell line models to more closely mimic in vivo conditions will increase their relevance.
- Investigating Variability: Addressing genetic and phenotypic diversity within cell lines will lead to more reliable experimental outcomes.
- Translational Research: Focusing on translating findings from astrocyte studies into clinical practices is vital for the development of new therapies.
"Astrocytes play an essential role in the brain, yet their functions are still being uncovered. The potential for future discoveries in this domain remains profound."
The quest to understand astrocytes through cell lines underscores the importance of this research area. It helps clarify their contributions to the broader neural networks, ultimately paving the way for innovations in treating neurological disorders.