Additive Polymerization: Mechanisms and Applications
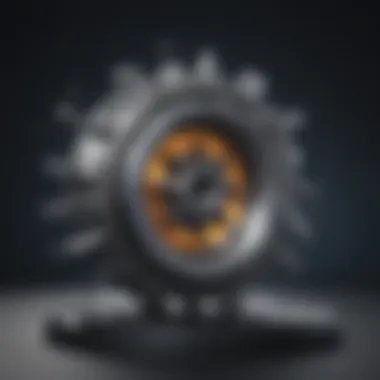
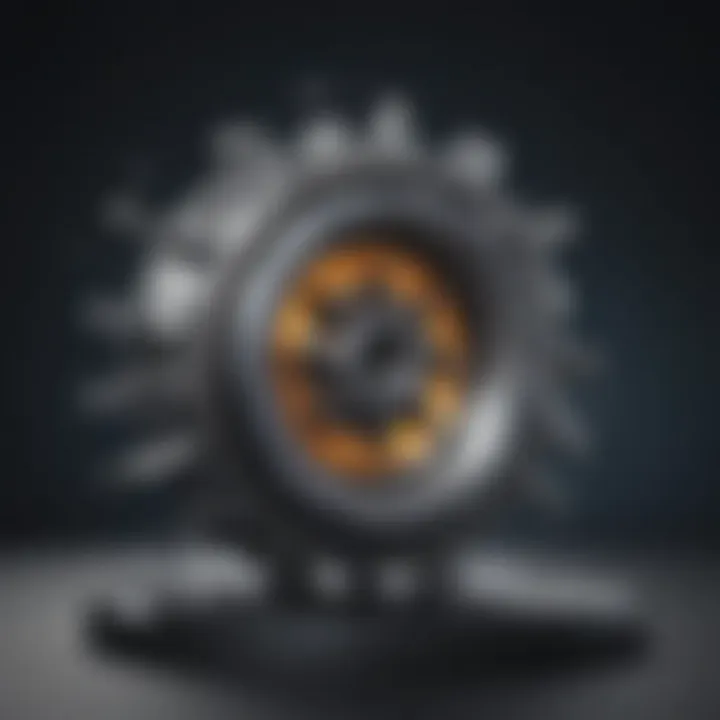
Intro
Additive polymerization is a technique that plays a central role in the field of polymer science. It's a method that allows for the growth of polymers through the addition of monomer units one by one. This contrasts with other processes like condensation polymerization, where there is a loss of small molecules during the reaction. The core of additive polymerization lies in its ability to create well-defined structures, making it a favorite among chemists and engineers.
Understanding this process isn't just for those knee-deep in the chemistry lab. The implications of additive polymerization stretch across various industries—think materials science, biotechnology, and even environmental management. It unlocks pathways to developing new materials, such as biodegradable plastics or advanced composites, which have become increasingly vital as the world grapples with sustainability challenges.
In the following sections, we will explore the various mechanisms that underpin additive polymerization, delve into its applications, and consider the broader implications of this crucial process.
Key Findings
- Mechanisms of Growth: Additive polymerization primarily occurs through chain growth mechanisms, which can be initiated in several ways, most notably through heat, light, or chemical initiators.
- Types of Polymers: This process gives rise to a range of polymers, including polystyrene and polyethylene, which are foundational to many products in everyday life.
Significance of findings within the scientific community
The significance of these findings goes beyond establishing a foundational understanding. Researchers have explored a variety of additives and modifications that can improve the properties of the resulting polymer. These advancements contribute immensely to both theoretical studies and practical applications.
"Additive polymerization is more than a synthesis method; it's a transformative approach that could redefine material utilization in modern society."
Implications of the Research
Applications of findings in real-world scenarios
The application of additive polymerization is vast. Industries utilizing these processes include:
- Medicine: Biodegradable polymers created via additive polymerization are revolutionizing drug delivery systems. They can be tailored to release medication over a specific duration, enhancing patient care.
- Construction: Advanced plastics optimized through this method contribute to more durable and sustainable building materials.
- Environmental Management: Efforts are underway to develop polymers that can break down after their useful life, aiming to significantly reduce plastic waste.
Potential impact on future research directions
Looking toward the future, research in additive polymerization is likely to focus on:
- Innovative Green Chemistry: Emphasis on environmentally benign practices to reduce toxic waste.
- Smart Polymers: Development of responsive materials that can adapt to their surroundings, holding potential in fields as diverse as healthcare and logistics.
- Recycling and Circular Economy: Enhancing the recyclability of polymers produced through additive polymerization, thereby supporting more sustainable practices in material usage.
In summary, the exploration of additive polymerization reveals a process that is as complex as it is beneficial. Each advancement opens new avenues for innovation, underlining its importance in the ongoing drive for sustainable development and technological progress.
Intro to Additive Polymerization
Additive polymerization serves as a cornerstone in the realm of polymer science, being pivotal to the development and application of myriad materials utilized across various industries. Understanding this process is key for students, researchers, educators, and professionals alike as it enlightens them on how complex structures can evolve from the simple act of joining small molecules, known as monomers. The importance of this topic in this article leads to a close examination of both the mechanisms and real-world implications of additive polymerization.
Notably, additive polymerization can result in high-performance materials with properties finely tuned to specific applications, ranging from everyday plastics to sophisticated biomedical devices. By delving into fundamental principles, mechanisms, and the roles played by various instigators of the polymerization process, this article aims to provide a holistic view that not only highlights practical applications but also addresses the broader environmental impacts.
Definition and Overview
Additive polymerization, often aligned with the term "chain-growth polymerization," is a process whereby monomer units add successively to form a polymer. A defining characteristic is that the addition process initiates at reactive sites within a monomer, leading to growing chains without the formation of byproducts. This uniqueness brings about a number of advantages:
- Rapid Synthesis: The mechanism is efficient, enabling the swift production of polymers.
- Structural Control: The process allows for a high degree of control over the polymer structure and functionality.
The underlying reaction steps include initiation, propagation, and termination, each contributing to the characteristics of the final product. The understanding of these phases is crucial for controlling polymer properties, making these principles essential for innovation in polymer science and engineering.
Historical Context
The narrative of additive polymerization traces back to the early 20th century, when scientists began uncovering the mysteries of polymer chemistry. Key developments marked this journey:
- In 1933, the German chemist Hermann Staudinger theorized that polymers were macromolecules formed by repeated monomer units, laying groundwork for modern polymer science.
- The invention of polystyrene and polyvinyl chloride in the 1940s marked significant applications of additive polymerization in consumer products.
Throughout the latter half of the century, research burgeoned with the advent of synthetic methods like radical polymerization, which revolutionized the industrial approach to material production. As time marched on, the touches of science began reshaping entire sectors, leading to a surge in plastic usage, the backbone of modern conveniences. The evolution of these concepts is essential in understanding today's diverse applications of additive polymerization.
Fundamental Principles of Polymerization
Understanding the core principles of polymerization is essential to fully grasp the mechanisms and applications discussed in this article. These foundational elements set the stage for the complex interactions that occur during the formation of polymers, which play critical roles in various industries today. Whether one is delving into industrial production or research in materials science, a solid grasp of polymerization principles becomes indispensable.
Monomer Characteristics
Monomers are the building blocks of polymers, and their unique characteristics determine how they will behave during the polymerization process. Factors such as molecular weight, functional groups, and steric hindrance drastically influence the efficiency and the type of polymer that forms. For instance:
- Molecular Weight: High molecular weight monomers tend to form more complex structures, impacting the physical properties of the end polymer.
- Functional Groups: The presence of specific functional groups can lead to varied polymerization pathways. For example, monomers with vinyl groups readily engage in radical polymerization, while those with hydroxyl groups might be more suitable for step-growth mechanisms.
- Steric Hindrance: The spatial arrangement around a monomer’s reactive sites is a crucial factor. Bulky substituents can hinder access to these sites, slowing down the reaction or altering the conditions needed for polymerization.
Understanding these properties not only clarifies the choice of monomers in a particular application but also guides the optimization of polymerization conditions to achieve desired characteristics in the final product. For instance, in the case of poly(methyl methacrylate) (PMMA), its optical clarity and resistance to environmental stress are intimately tied to the specific properties of its monomers.
Polymer Structure
The structure of a polymer can vary widely based on its monomer composition and the polymerization technique employed. In this context, several points merit attention:
- Chain Length and Molecular Weight Distribution: The length of polymer chains in a given sample affects its mechanical properties, such as tensile strength and elasticity. A broader molecular weight distribution can lead to a composite with improved toughness.
- Crystallinity vs. Amorphousness: The arrangement of polymer chains affects not only the physical appearance but also thermal and electrical properties. Polymers with high crystallinity, like polyethylene, exhibit rigidity and strength, while amorphous polymers, such as polystyrene, are generally softer and more flexible.
- Copolymerization: Combining two or more different types of monomers can generate materials with tailored properties. For instance, alternating copolymers might exhibit unique properties that neither homopolymer could offer alone, such as improved thermoplastic characteristics.
Recognizing your polymer structure is vital, as it determines how materials will interact under stress or during exposure to various environmental factors. The durability, thermal stability, and chemical reactivity of polymers are often linked directly to their structural characteristics, revealing the intricacies of polymer science and its applications in real-world scenarios.
The relationship between monomer properties and polymer structure is fundamental in an era where engineered materials are pivotal for innovation across multiple sectors, from aerospace to biomedicine.
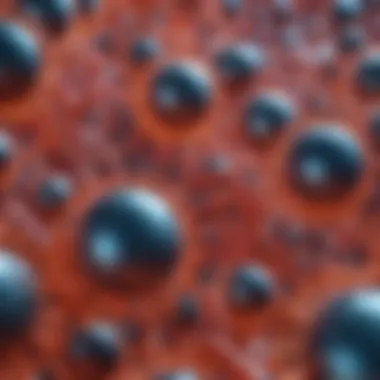
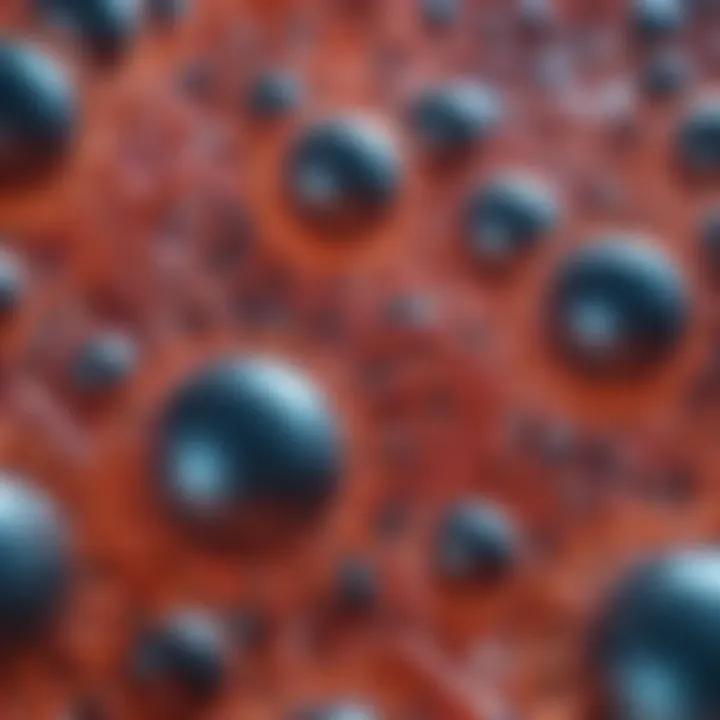
In sum, having a keen understanding of both monomer characteristics and polymer structure will lay the groundwork for deeper exploration into polymerization mechanisms, applications, and their broader implications in our world.
Mechanisms of Additive Polymerization
Understanding the mechanisms of additive polymerization is crucial as it lays the foundation for various applications in the material science landscape. This section delves into the intricate processes that govern how polymers form, providing insight into the properties and functionalities of the resulting materials. Each step in the polymerization journey—initiation, propagation, and termination—holds significance not just academically, but also in practical applications across diverse industries. The revelations from studying these mechanisms can lead to advancements in sustainable practices, revolutionary materials, and optimized processes.
Chain Initiation
Chain initiation is the first and a pivotal step in the realm of additive polymerization. This is the stage where the monomer molecules are activated to start the polymer chain. Various methods can bring about this initiation, including the use of heat, light, or chemical initiators. For instance, when applying heat, thermal initiators decompose, generating free radicals that initiate the polymerization process.
Initiators can be typically categorized into two groups: free radical initiators and ionic initiators. Free radicals are, quite literally, radicals—they jumpstart the polymerization process by breaking bonds in the monomers. On the contrary, ionic initiators create positive or negative charges in the monomers, leading to a different set of interactions.
"The initiation step reflects the delicate ballet where energy meets molecular structures, setting the stage for the grand performance of polymer synthesis."
The choice of initiator can significantly impact the resulting polymer's characteristics, such as molecular weight and distribution. Understanding the initiation phase gives researchers the leverage to engineer polymers with desired attributes, whether they seek flexible materials or robust composites.
Chain Propagation
Once initiation has taken place, the process shifts gears into chain propagation. This is where the real magic happens: active sites on the growing polymer chain react with additional monomer units, adding them to the chain. Essentially, each reaction links a new monomer to the existing chain, facilitating a rapid and ongoing growth cycle.
The speed of chain propagation can depend on several factors, including temperature and the concentration of monomers. A higher temperature generally leads to faster reactions as molecules move more quickly and collide with greater energy. However, there's a balancing act here; if the temperature is too high, it can cause the polymer to degrade before it fully forms.
Factors within the reaction environment can also dictate the efficiency of this propagation. For instance, if the concentration of monomers dwindles, the growth rate can slow down, leading to possible chain termination before reaching an optimal molecular weight.
Chain Termination
Chain termination is the final stage of the polymerization process and can occur in a few different ways. Often, it leads to the conclusion of the polymer chains, shaping the final properties of the material. As the name suggests, this step signifies the halting of monomer addition to growing chains.
Termination can happen through several mechanisms. One common method is combination, where two growing chains collide and join, effectively terminating their individual growth. Another method is disproportionation, which involves the transfer of a hydrogen atom from one chain to another, simultaneously terminating one while altering the other.
The conditions under which termination occurs can radically influence the structure and stability of the final polymer. Adjustments in the polymerization process can lead to materials with unique attributes or functionality, making this an essential consideration for researchers and industrial applications alike.
In summary, the mechanisms of additive polymerization—from initiation through propagation to termination—are not merely steps in a process but are core elements that define how polymers behave, perform, and interact. A comprehensive grasp of these mechanisms is integral for exploring new avenues in polymer research and development.
Types of Additive Polymerization
The landscape of additive polymerization is as varied as the applications it serves. Understanding the different types of additive polymerization is crucial because each type presents its own unique mechanisms, advantages, and challenges. This section will explore three predominant types: radical polymerization, cationic polymerization, and anionic polymerization. By diving into their characteristics, we aim to illuminate how these processes impact the broader spectrum of polymer science, and why they matter in both industrial and research contexts.
Radical Polymerization
Radical polymerization is the most widely employed type of polymerization and is notable for its efficiency and versatility. In this process, the formation of reactive radicals initiates the chain reaction that leads to polymer growth. These radicals can be generated through thermal means, photochemical processes, or redox reactions, allowing for various starting conditions.
One might think of radical polymerization as a race where every runner's entry drastically changes the outcome. The runners here are the reactive monomers that come together in a chain reaction. This dynamic activity is crucial when one considers the sheer multitude of products that can be produced—from plastics like polystyrene to high-tech materials such as polyacrylonitrile, which is used in carbon fiber production.
Benefits of radical polymerization include:
- Speed: The chain initiation step is exceptionally quick, allowing for the large-scale production of polymers.
- Diversity: A wide range of monomers can participate, offering versatility in polymer design.
- Simplicity: The process is straightforward and adaptable for various applications.
However, this type is not free from challenges. For instance, the process needs careful control to avoid unwanted termination reactions or side reactions that can compromise product quality.
"Radical polymerization is like cooking with a pinch of salt; a bit too much, and you can ruin the dish."
Cationic Polymerization
Cationic polymerization relies on positively charged ions to initiate the polymerization process. Here, the initial cationic species reacts with the monomer, creating a new cation that continues to react with additional monomers, leading to the formation of the polymer chain. This method is typically slower than radical polymerization but allows for greater control over molecular weight and structure.
In the realm of cationic polymerization, epoxy resins and rubber (like polyisobutylene) take center stage, showcasing flexible and durable materials for various applications. The ability to fine-tune the conditions—such as solvent choice and temperature—gives cationic polymerization a significant edge when precision is required.
Some points to consider:
- Controlled Structure: It allows precise tailoring of polymer architecture, which can enhance specific material properties.
- Wide Array of Monomers: Can accommodate different monomers that may not polymerize effectively through other means.
- Low Side Reactions: The process generally minimizes by-products compared to some other methods.
Yet, this pathway has its drawbacks, including sensitivity to moisture and the potential for premature termination by additives or impurities. Thus, maintaining stringent conditions is paramount for successful outcomes.
Anionic Polymerization
Anionic polymerization involves the use of anions to propagate the polymer chain. Anions serve as active sites, capturing monomers. This type allows for the formation of high molecular weight polymers with very narrow molecular weight distributions, making it ideal for producing precise materials like polystyrene-b-polyethylene oxide block copolymers used in drug delivery.
One distinguishing feature of anionic polymerization is its ability to create living polymers, meaning the polymer chains can continue to grow when new monomers are introduced. This characteristic provides a considerable advantage in creating advanced materials with specific design criteria.
Key considerations include:
- High Precision: Produces well-defined polymers that meet tight tolerances.
- Versatile Viscosity Management: Tailors the viscosity for specific applications, particularly important in coatings and adhesives.
- Control Over Molecular Weight: Excellent control allows for customized material properties.
However, anionic polymerization requires an inert atmosphere to avoid moisture interference, adding complexity to the process. Researchers must also handle highly reactive anionic intermediates with care.
As you can see, understanding the nuances of these types of additive polymerization not only enhances our knowledge but also opens up a pathway for innovative applications and material designs. The choice of polymerization method can fundamentally alter the properties and performance of the resulting material, indicating that no one size fits all in the world of polymers. This deep dive into the types of additive polymerization sets the stage for further exploration of their applications and implications in the following sections.
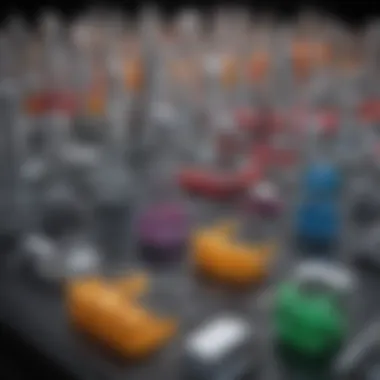
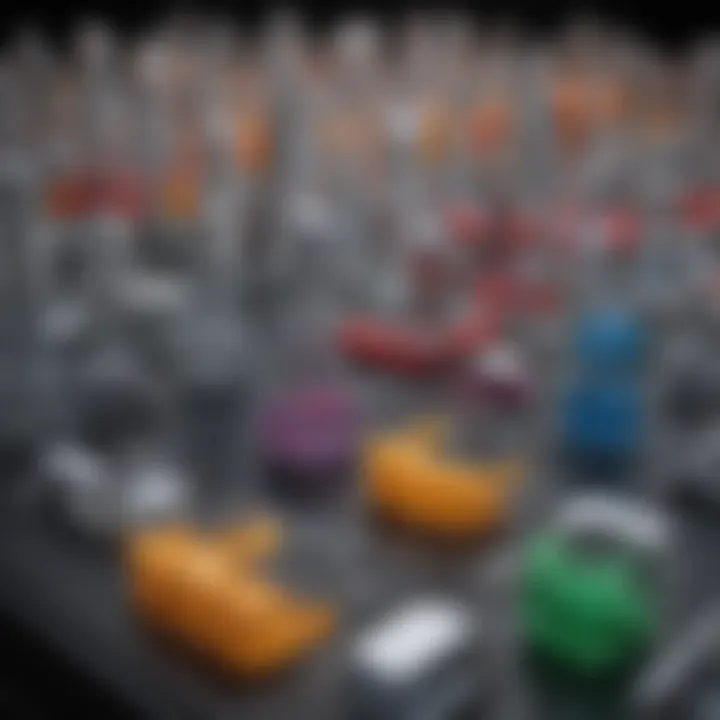
Role of Initiators in Polymerization
Initiators play a crucial role in the world of additive polymerization, acting as the catalysts that set the entire reaction into motion. Without these agents, the polymerization process wouldn't even get started, much like trying to light a fire without a spark. The significance of initiators in polymerization cannot be overstated; they determine not only the efficiency of the reaction but also influence the properties of the resulting polymer.
Types of Initiators
There are several types of initiators utilized in additive polymerization, each with its own characteristics and suitable uses:
- Radical Initiators: These are perhaps the most common type. They generate free radicals, which are highly reactive species capable of initiating polymer chains. Common examples include benzoyl peroxide and azobisisobutyronitrile (AIBN).
- Cationic Initiators: In these cases, the initiator donates a proton to initiate the polymerization, making it particularly useful for creating polymers that require acidic conditions. Protons can come from sources like Lewis acids, such as boron trifluoride.
- Anionic Initiators: These are useful for polymerization involving monomers that can stabilize anionic species. Unlike the previous types, they create negatively charged species to kick off the reaction. Common examples include butyllithium.
Each of these initiators has its unique context, depending on the polymerization desired and the monomers in use.
Initiation Mechanisms
The mechanisms of polymer initiation shed light on how these initiators coax monomers into joining forces:
- Thermal Decomposition: This involves heating a radical initiator until it breaks apart, forming radicals that react with monomer molecules. It’s often applied in bulk polymerizations.
- Chemical Reaction: So, let’s say a suitable reagents are mixed. In this method, certain chemicals interact to create radicals or ions that start the polymer chain. For example, in cationic polymerizations, an acid might react to produce the necessary proton for initiating the reaction.
- Photoinitiation: Here, light does the heavy lifting. Special initiators absorb ultraviolet or visible light, creating radicals that react with monomers to propagate the polymer chain. This method is advantageous in applications where controlled or localized polymerization is necessary.
Initiators can make or break the polymerization process; understanding how they work helps in tailoring specific polymers for various applications.
The choice of initiator and the mechanism of initiation can dramatically influence the molecular weight and structure of the resulting polymer. A deeper understanding of these elements leads to enhanced methodologies in polymer synthesis, equipping chemists and industries alike with the knowledge to innovate and improve existing materials.
Conditions Affecting the Polymerization Process
Understanding the conditions that affect the polymerization process is not just a footnote in the grand narrative of additive polymerization; it’s a cornerstone concept that can make or break the efficiency and effectiveness of polymer synthesis. Temperature and pressure, in particular, play pivotal roles in controlling the rate of polymerization and the characteristics of the resulting polymers.
Effectively, tweaking these conditions can lead to entirely different outcomes. For example, altering the temperature may influence the mobility of monomers and reactants, thereby impacting how quickly polymer chains form. This section will delve into the intricacies of these variables and their significant influence on polymerization, highlighting why paying attention to them is critical for both research and practical applications.
Temperature Influences
Temperature can be likened to the conductor in an orchestra—the right warmth or chill can lead to a symphony of polymer growth or a discordant halt. As temperature escalates, molecular motion increases, enhancing the likelihood of monomer collisions. This can boost the reaction rate and, consequently, polymerization speed. However, there’s a catch: too much heat might result in undesirable side reactions, yielding off-specification products that do not meet intended application standards.
- Optimal Range: Each polymerization method has a sweet spot when it comes to temperature. For instance, radical polymerization often operates efficiently at moderate temperatures, while cationic processes may require lower temperatures to minimize chain termination.
- Thermal Decomposition: Another key concern is the risk of thermal decomposition of the initiator or monomers at elevated temperatures. This outcome can jeopardize the entire polymerization process. Therefore, it’s essential to find that delicate balance where reaction speeds are maximized without triggering breakdown.
- Viscosity Changes: Temperature fluctuations can also lead to changes in viscosity. A lower viscosity at higher temperatures may improve mixing, but when the reaction cools, viscosity increases, potentially leading to a gel-like state that complicates further processing.
Pressure Effects
Pressure, much like the pressure of public expectations, can have a profound impact on polymerization reactions. In environments where gases are involved, such as with certain gas-phase polymerizations, increased pressure can heighten reaction rates. Adding pressure can alter the physical properties of the monomers, influencing their solubility and, therefore, their ability to react effectively.
- Enhanced Reactivity: Increasing pressure often results in enhanced reactivity and increased yield. This is closely related to Le Chatelier’s principle, where a system at equilibrium adjusts to counteract changes in conditions, favoring product formation.
- Physical State Changes: Pressure variation can cause phase changes, which may promote or inhibit polymerization. For example, a gas-phase reaction may become liquid under high pressure, which can sharply alter the dynamics of the system.
- Design Considerations: Pressurized environments require specific design adaptations for reactors. Engineers must consider how the apparatus can safely withstand increased internal pressures, while maintaining effective control over temperature fluctuations that may accompany pressure modifications.
Conclusion: Temperature and pressure are not mere footnotes in the polymerization narrative; they are integral to the orchestration of the polymerization process. Adjusting these conditions can unlock new realms of polymer properties and functionalities, making the science behind conditions in polymerization both a practical and an exciting area of study.
In the realm of additive polymerization, the implications of these conditions stretch from the lab bench to industrial applications, accentuating their relevance across the board.
Applications of Additive Polymerization
The realm of additive polymerization extends well beyond the laboratory bench, spilling into various industries that rely on polymer materials to innovate and solve real-world problems. Understanding the applications of this chemical process is essential, as it underpins the foundational technologies across a multitude of sectors. Not only does this process facilitate the creation of materials with tailored properties, but it also influences economic growth and addresses critical societal needs. Below, we’ll explore the significant applications of additive polymerization in industrial settings, biomedical fields, and the rapidly advancing fields of nanotechnology and materials science.
Industrial Applications
In the industrial landscape, additive polymerization plays a crucial role in producing plastics and composites that serve a range of functions. For example, companies such as DuPont utilize this process to develop strong, lightweight materials used in automotive and aerospace sectors. Here are some critical points related to industrial applications:
- Production of Plastics: Additive polymerization is behind the creation of common polymers such as polyethylene and polystyrene, essential in packaging, consumer goods, and construction materials.
- Customizable Materials: Businesses can tailor the properties of polymers based on specific application needs—like selecting the right flexibility, transparency, or thermal resistance.
- Cost-Effectiveness: The scalability of additive polymerization allows manufacturers to produce large volumes of uniform product efficiently, minimizing both waste and production costs.
- Environmental Considerations: With increasing awareness of sustainability, many industrial sectors are exploring bio-based polymers derived from renewable resources. This shift is reshaping manufacturing practices and supply chains across industries.
"The customizable nature of additive polymerization allows industries to innovate rapidly, tailoring materials for ever-evolving market needs."
Biomedical Applications
The contributions of additive polymerization to the biomedical field are profound. This process leads to the development of polymers that are pivotal in medical devices, drug delivery systems, and tissue engineering. Some important aspects include:
- Medical Devices: Polymers created via additive polymerization are essential in devices like stents, catheters, and prosthetics, enabling enhanced biocompatibility and functional performance.
- Drug Delivery Systems: The ability to control the release rate of medications through specialized polymer design makes additive polymerization significant in treating chronic illnesses and improving patient outcomes.
- Tissue Engineering: A growing area of research, here polymers serve as scaffolding for cell growth, providing a structure that can mimic natural tissues and facilitate regenerative medicine initiatives.
- Safety and Efficacy: By using polymers that prioritize bio-compatibility, researchers can minimize harmful reactions in medical applications, thereby improving patient safety and treatment efficacy.
Nanotechnology and Materials Science
As we venture into the future, the intersection of additive polymerization with nanotechnology and materials science presents a very exciting prospect. The manipulation of materials at the nanoscale opens up new avenues for tech advancements. Key points include:
- Nanocomposites: Polymers integrated with nanoparticles enable the creation of materials with unique properties—higher strength, lighter weight, or improved conductivity—ideal for electronics and aerospace applications.
- Smart Materials: Through additive polymerization, researchers are developing materials that can respond dynamically to environmental stimuli, leading to smarter, more efficient products.
- Sustainability Innovations: In this domain, polymer research also focuses on creating eco-friendly materials that degrade over time while maintaining functionality during their lifecycle.
- Interdisciplinary Approaches: Collaborations across chemistry, engineering, and even biology are allowing for breakthroughs that further advance the understanding and capabilities of polymer materials.
Embracing the vast applications of additive polymerization not only catalyzes technological advancements but also brings forth discussions about responsibility and innovation with an eye on sustainability. Each sector is not just utilizing additive polymerization; it’s transforming its potentials into solutions for the future.
The Environmental Impact of Polymerization
The significance of understanding the environmental impact of polymerization cannot be overstated, especially as our world grapples with the consequences of plastic pollution and resource depletion. Additive polymerization is no exception; while it offers remarkable advantages in material properties and functionality, its environmental footprint remains a critical concern. By exploring how polymerization processes interact with environmental principles, we can uncover potential paths toward more sustainable practices in polymer science.
Sustainability Concerns
The sustainability concerns surrounding additive polymerization stem primarily from several factors:
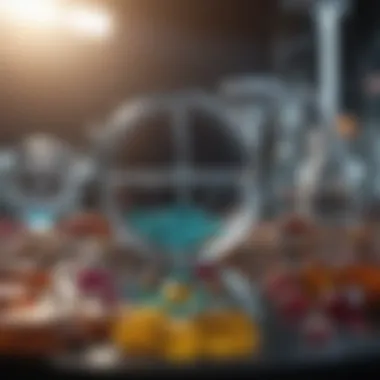
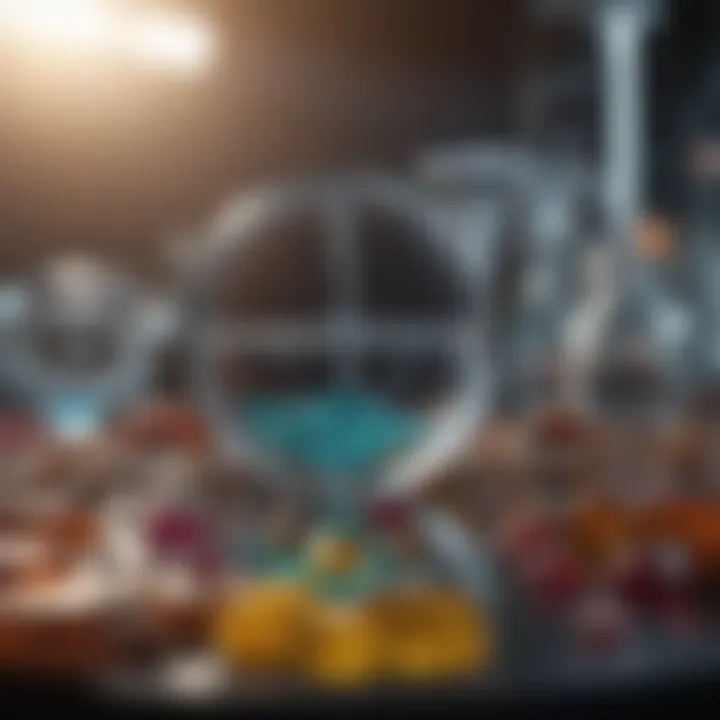
- Resource Depletion: The starting materials for many polymers, particularly those produced using conventional petroleum-based methods, come from non-renewable resources. The extraction and processing of fossil fuels have significant ecological consequences, contributing to habitat destruction and climate change.
- Energy Consumption: The synthesis of polymers through additive methods often requires substantial amounts of energy. High temperatures and pressures are commonplace in several polymerization techniques, resulting in considerable carbon footprints.
- Waste Generation: The polymerization process, depending on the method used, can lead to the generation of hazardous by-products. Often, these waste products are not biodegradable and can persist in the environment, adding to pollution concerns.
Moreover, the potential for polymers to contribute to problems like microplastic pollution cannot be overlooked. As larger plastic items break down, they can fragment into smaller pieces that contaminate oceans and waterways, posing threats to wildlife and human health. Thus, the discourse around polymerization must also include innovative solutions to mitigate these impacts, perhaps through recycling and reprocessing initiatives.
Biodegradable Polymers
The emergence of biodegradable polymers represents a beacon of hope in the efforts to counteract the adverse environmental effects of traditional polymerization methods. These materials are designed to decompose into natural substances when exposed to specific environmental conditions. Here are a few salient points regarding biodegradable polymers:
- Types of Biodegradable Polymers: Commonly used biodegradable polymers include polylactic acid (PLA), polyhydroxyalkanoates (PHA), and starch-based polymers. Each has unique properties that make them viable alternatives for various applications, particularly in packaging and agricultural films.
- Environmental Benefits: Many biodegradable polymers break down in composting systems, returning valuable nutrients to the soil. This closed-loop system can help foster sustainability by reducing waste while promoting soil health.
- Market Growth: The demand for biodegradable options is on the rise, driven by increased consumer awareness. Industries are starting to proactively adopt these materials to align with sustainability goals, pushing the boundaries of traditional practices.
"Adopting biodegradable polymers is essential for the future; they offer not only the potential to reduce waste but also a more harmonious relationship with our environment."
In summary, while additive polymerization presents certain environmental challenges, advancements such as biodegradable polymers signal a shift towards more sustainable practices. By understanding the intricate relationship between polymer chemistry and environmental impact, researchers and industries can embark on innovative paths that leverage the benefits of additive polymerization without compromising our planet's health.
Emerging Trends in Polymer Research
Emerging trends in polymer research shine a light on how this field is evolving, tapping into new potentials and addressing modern challenges. As societies grow increasingly reliant on sophisticated materials, innovation in polymer science becomes a pivotal point of focus. Researchers and industry professionals alike are keen on understanding how these trends not only enhance existing applications but also pave the way for future breakthroughs. By exploring these patterns, we can better appreciate the shifts happening in the landscape of additive polymerization.
Innovative Techniques in Polymer Synthesis
The landscape of polymer synthesis is undergoing a transformation, largely driven by innovative techniques that are continuously being developed. Various approaches are gaining traction, such as:
- Living Polymerization: This technique allows for precise control over molecular weight and distribution. It promotes the synthesis of narrow polydispersity which is crucial for producing high-performance materials.
- Click Chemistry: Its simplicity and efficiency in forming polymers make it a popular choice. This method is significant for creating functionalized polymer architectures that can be tailored for specific applications.
- Microfluidics: An emerging area, this involves manipulating fluids at a microscale. It enables high-throughput screening of polymers, accelerating material discovery and optimization.
- Green Polymer Chemistry: This focuses on minimizing environmental hazards. Techniques that use bio-renewable feedstocks and reduce solvent waste are being explored, which aligns with global sustainability goals.
These techniques not only refine the traditional methods of synthesis but also broaden the scope of what can be achieved with polymers. With a growing emphasis on efficiency and sustainability, these approaches are expected to have lasting impacts.
Interdisciplinary Approaches
A distinctive feature of modern polymer research is the interdisciplinary collaboration that fuels innovation. The integration of knowledge from different fields researchers enhances the depth and breadth of polymer science. Some critical interdisciplinary trends include:
- Nanotechnology: Melding nanotech with polymer science opens doors to creating materials with enhanced properties. Applications in electronics, medicine, and catalysis are particularly promising.
- Biomaterials: Collaborations with bioengineering are crucial in developing polymers that mimic natural tissues, resulting in significant advancements in biomedical applications.
- Computational Modeling: This plays a vital role in predicting polymer behavior and properties before physical synthesis, making the research process faster and more efficient.
- Data Science: Leveraging big data to analyze polymer properties and performance patterns assists researchers in making informed decisions in the early stages of development.
The horizon of polymer research is broadening, fueled by these collaborations which yield innovative solutions to complex problems. The beauty of these interdisciplinary approaches is that they unite a myriad of perspectives, driving forward the science of polymers in ways we might not have envisioned before.
"A promising future for polymers awaits, as innovative techniques and cross-disciplinary partnerships unlock new possibilities in synthesis and application."
This comprehensive dive into emerging trends underscores the dynamic nature of polymer research, making it an exciting area for students, researchers, educators, and professionals. The ongoing developments are not just about refining old methods; they are about reimagining what is possible in the world of polymers.
Future Perspectives in Additive Polymerization
As we stand at the cusp of significant advancements in polymer science, the future perspectives in additive polymerization unveil a tapestry of opportunities and challenges. This segment aims to unpack what lies ahead, focusing on specific elements that can drive the field forward while highlighting considerations for researchers and practitioners.
Additive polymerization is more than just a method of creating materials; it is a landscape ripe for innovation. The importance of exploring future perspectives in this realm is paramount. One pivotal aspect is the potential for developing polymers that are not only functional but also sustainable. Researchers are increasingly looking into eco-friendly monomers and processes, which can help lessen the environmental footprint of polymer production. This shift towards sustainability can yield benefits in terms of regulatory compliance, brand loyalty, and customer demand for greener products.
Another critical element to consider is the role of interdisplinary collaboration. By blending expertise from fields such as chemistry, engineering, and computer science, we pave the way for breakthroughs in polymer design and application. An illustration of this could be the synergy between polymer chemists and material engineers to harness advanced properties in polymers, leading to highly specialized applications across various industries, from aerospace to healthcare.
However, alongside these opportunities arise challenges. As the complexities of polymer behaviors are understood better, there arises a need for more sophisticated analysis and modeling techniques. This requires ongoing investment in research and development, as well as a commitment to education and training within the field. Moreover, balancing innovation with sustainability is no small feat, as some applications demand materials that may still carry environmental costs.
In summary, the exploration of future perspectives in additive polymerization not only brings forth insight into next-generation materials but also provokes critical thinking on how we can address real-world issues through scientific advancements. Navigating these waters will demand a blend of creativity, rigor, and adaptability.
Challenges and Opportunities
To navigate the future of additive polymerization effectively, it’s essential to identify the challenges and opportunities that lie ahead:
- Scalability: Transitioning from laboratory-scale successes to industrial applications is often fraught with obstacles. Efficiencies need to be improved to ensure that new polymer processes can be adopted widely.
- Cost-Effectiveness: Developing advanced materials must also consider the economic side; finding ways to produce innovative polymers at lower costs will be key.
- Market Demand: As industries evolve, the complexity of consumer demands increases. Understanding upcoming trends and varying needs is crucial to inform research directions.
Role of Artificial Intelligence in Polymer Chemistry
Artificial Intelligence (AI) is set to revolutionize how we approach the field of polymer chemistry. By analyzing vast sets of data and recognizing patterns that may not be immediately obvious, AI can enhance polymer discovery and optimization. Some important avenues include:
- Predictive Modeling: AI can help predict polymerization outcomes based on initial conditions, aiding in the design of materials with desired properties, thus saving time and resources.
- Machine Learning: By utilizing machine learning algorithms, polymer scientists can efficiently sift through experimental data, helping to identify successful formulations much faster than traditional methods.
- Automated Syntheses: AI can assist in automating and optimizing synthesis processes, leading to increased control and reproducibility while reducing the scope for human error.
As these technologies progress, the integration of AI into polymer chemistry is expected to catalyze not just efficiency but perhaps even creativity in polymer design. Embracing these advancements will likely be central to overcoming the challenges ahead.
"The future of additive polymerization will be shaped by our ability to harness technology, fostering a culture of innovation while ensuring sustainability at every step."
In summary, the future of additive polymerization holds great promise, with advances driven by a blend of innovative research, sustainability, and AI. Being vigilant about the challenges while keeping an eye on the opportunities will be key for unlocking new frontiers in this pivotal field.
Culmination
The conclusion serves as a vital component of this article, tying together the various elements of additive polymerization discussed throughout. Understanding how polymer synthesis operates is essential, particularly as we lean further into an era where these materials—once considered simple compounds—hold revolutionary potential across numerous fields.
Summary of Key Points
- Definition and Mechanisms: Additive polymerization is characterized by the sequential addition of monomer units, facilitating larger and more complex polymer structures. By focusing on mechanisms such as chain initiation, propagation, and termination, we gain insight into the operational framework of these reactions.
- Types and Applications: The different types of additive polymerization—radical, cationic, and anionic—each have unique characteristics that make them suitable for specific applications, from industrial manufacturing processes to advanced biomedical solutions. These distinctions showcase how versatile additive polymerization can be.
- Environmental Considerations: The discussion has also highlighted sustainability issues inherent in polymer production. With the rise of biodegradable polymers, there lies an opportunity to balance the benefits of additive polymerization with a conscious approach toward environmental preservation.
- Future Research Directions: The unfolding landscape of polymer research, particularly through interdisciplinary approaches and innovative techniques, promises to push the boundaries of what is possible with additive polymerization. Leveraging advancements in technology, such as artificial intelligence, could lead to more efficient and sustainable practices in the field.
Ultimately, the importance of additive polymerization transcends the chemistry discipline and delves into broader implications that affect industries and our environment. Understanding these complexities equips researchers and professionals with the necessary tools to innovate responsibly in a world increasingly driven by synthetic materials.
Call for Further Research
As we conclude, it's imperative to recognize the vast territory yet to be explored in the domain of additive polymerization. The need for ongoing research can’t be overstated; from refining existing techniques to discovering new applications, the possibilities are endless.
- Focus on Sustainability: Future investigations should consider environmental impacts more rigorously. Questions surrounding the lifecycle of polymers, their long-term ecological footprint, and efficient recycling methods need thorough exploration to ensure that advancements don't come at the planet's expense.
- Interdisciplinary Collaboration: The intersection of polymer science with fields such as materials science, biotechnology, and even artificial intelligence is ripe for exploration. How can polymer chemists and material engineers work together to develop smarter and more functional materials?
- Emerging Technologies: The role of cutting-edge technologies in polymer synthesis could transform the field. For instance, experimenting with 3D printing techniques could yield customized polymer designs tailored for specific applications, a frontier that warrants diligent research.
Encouraging further scrutiny and dialogue within the scientific community will pave the way for innovations that address current challenges while enhancing the usability and performance of polymers. In a world where each synthesis could have far-reaching implications, let’s aim for a future where our advancements align with the needs of our environment.