The Intricacies of mRNA Production and Function
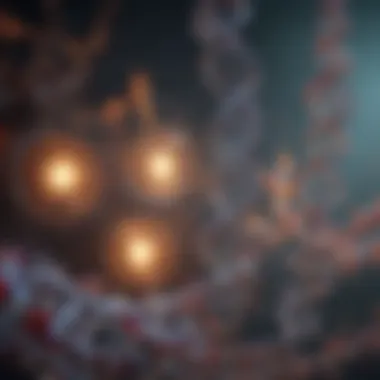
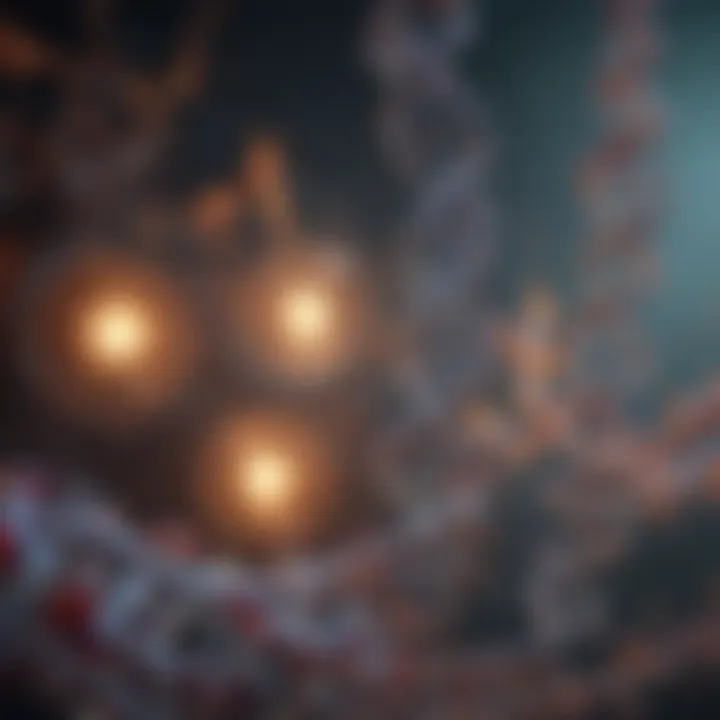
Intro
The study of messenger RNA (mRNA) is vital to understanding how genetic information is translated into functional proteins. mRNA acts as a crucial intermediary in the process of transcription, which converts the information encoded in DNA into a usable format within cells. This dynamic interplay between various cellular components is critical for life and underpins many applications in biotechnology, medicine, and genetics.
The synthesis of mRNA involves multiple steps and relies on numerous factors, including enzymes, cellular compartments, and regulatory mechanisms. Understanding how these elements interact provides insight into gene expression and the implications of mRNA in numerous biological processes.
As we delve into this detailed exploration of mRNA production, we will examine the key features of transcription, the relevant cellular mechanics, and the broader implications of this research for future life sciences developments.
Understanding mRNA
The comprehension of mRNA is fundamental to grasping molecular biology and its implications in various fields. mRNA, or messenger RNA, is a key player in translating genetic codes from DNA into functional proteins. In this section, we will explore the critical elements of mRNA, its essential functions, and the broader implications it holds in biological and biotechnological contexts.
Understanding mRNA involves recognizing its role not just as a messenger but as a critical connector between genetics and functionality in living cells. Without mRNA, the instructions encoded in DNA would remain dormant and inactive. This lack of activity is crucial as proteins are the workhorses of the cell, involved in virtually every cellular process.
This article discusses several specific aspects of mRNA, including its definition and function, the process it undergoes for synthesis, its structure, and its importance in protein synthesis. The intricacies of these topics are relevant for students, researchers, and professionals interested in genetics, biochemistry, and biotechnology.
Through examining these elements, readers will gain a deeper understanding of how mRNA operates within the cellular environment, influencing everything from gene expression to potential applications in medicine and technology.
What is mRNA?
Messenger RNA (mRNA) serves as a crucial intermediary in the central dogma of molecular biology, where information flows from DNA to RNA and then to proteins. It is transcribed from DNA in the nucleus and then transported to the cytoplasm where it directs protein synthesis. mRNA carries the genetic instructions that dictate cellular function and development.
The formation of mRNA involves copying a segment of DNA, which is accomplished through the process of transcription. Each mRNA molecule is typically specific to a gene, making it unique in terms of the protein it codes for. This specificity is essential as it allows the cell to produce exact amounts of proteins based on its metabolic needs.
The Role of mRNA in Protein Synthesis
mRNA plays a pivotal role in the synthesis of proteins, a process that occurs mostly in the ribosomes found in the cytoplasm. After mRNA is produced in the nucleus, it migrates into the cytoplasm where ribosomes read the sequence of nucleotides in the mRNA strand.
Proteins are composed of amino acids, and the sequence of nucleotides in mRNA determines the order in which these amino acids are assembled. This process happens in two main stages:
- Translation: The ribosome reads the mRNA sequence and translates it into a specific sequence of amino acids.
- Post-Translation: The newly formed protein may undergo modifications to become fully functional.
Therefore, the role of mRNA extends beyond mere information transfer; it is integral to the process that facilitates biological function.
Structure of mRNA
The structure of mRNA is intricately designed to support its function in protein synthesis. An mRNA molecule consists of several key components:
- 5' Cap: A modified guanine nucleotide added to the 5' end of the mRNA. This cap protects the mRNA from degradation and is important for the initiation of translation.
- Coding Sequence: This region is made up of codons, which are triplets of nucleotides that specify an amino acid in the resultant protein.
- Poly-A Tail: A sequence of adenine nucleotides added to the 3' end of the mRNA after transcription. This tail enhances the stability of the mRNA and regulates its translation.
These structural elements of mRNA are critical for its stability, functionality, and ultimately for ensuring proper protein synthesis.
Understanding the structure of mRNA can provide insights into genetic regulation and the dynamics of protein synthesis, which are essential for advancements in fields like genetic therapy and molecular biology.
The Cellular Environment of mRNA Production
Understanding the cellular environment where mRNA production occurs is vital for comprehending the overall processes of gene expression and regulation. The cellular environment encompasses numerous components that facilitate the transcription of DNA into mRNA, which serves as the crucial intermediary in the synthesis of proteins. This environment is defined by specific structures, functions, and interactions between cellular elements that contribute to effective mRNA production. In short, these cellular contexts influence how genes are expressed and how proteins are ultimately formed. The importance of this topic lies in its implications for various fields, including genetics, molecular biology, and biotechnology.
Key Cellular Components
The cellular environment relies on several key components:
- DNA: This is the source of the genetic code that is transcribed into mRNA. It is located in the nucleus and contains regions known as genes.
- RNA Polymerase: An enzyme essential for the transcription process. It reads the DNA template and synthesizes the complementary RNA strand.
- Nucleotides: The building blocks of RNA that RNA polymerase uses to construct the mRNA strand.
- Transcription Factors: Proteins that bind to specific DNA sequences to regulate the transcription of mRNA.
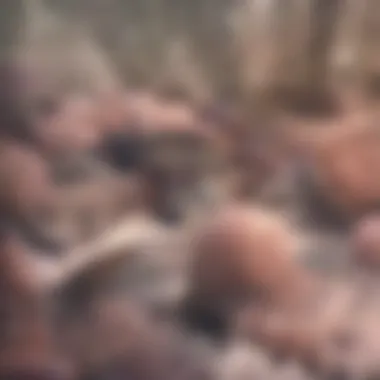
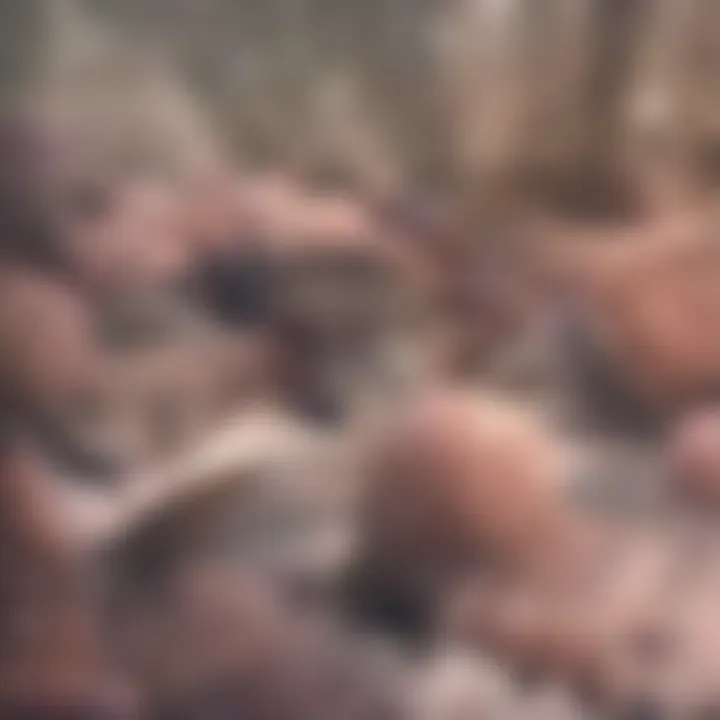
These components work together to ensure the efficient production of mRNA, which is necessary for maintaining cellular functions and responding to environmental changes.
Nucleus: The Site of Transcription
The nucleus serves as the central hub for mRNA production. It houses the cell's DNA and is the site where transcription occurs. During transcription, RNA polymerase binds to the promoter region of a gene and begins synthesizing an mRNA strand based on the complementary DNA template.
The process of initiation takes place in the nucleus. Once the mRNA is synthesized, it undergoes several modifications before it can be exported from the nucleus. These modifications include capping, splicing, and polyadenylation, which collectively prepare the mRNA for translation in the cytoplasm. This makes the nucleus not just a structural component, but a dynamic space vital for the proper expression of genes.
Cytoplasm: The Role in Translation
Once the modified mRNA exits the nucleus, it enters the cytoplasm. This is where translation occurs, transforming the mRNA sequence into a functional protein. The role of the cytoplasm is equally significant. Here, ribosomes, which are the molecular machines that synthesize proteins, bind to the mRNA.
During translation, the ribosome reads the mRNA sequence in sets of three nucleotides, known as codons. Each codon corresponds to a specific amino acid, and as the ribosome moves along the mRNA, it assembles a growing polypeptide chain. This link between mRNA and protein synthesis highlights the crucial interplay between the nucleus and cytoplasm in the life of a cell.
"In summary, the cellular environment is a carefully coordinated setting where key components interact to ensure that mRNA is accurately produced and utilized for protein synthesis."
Understanding how cellular compartments influence mRNA production and its subsequent role in translation is fundamental to advancements in genetic studies and related biotechnologies.
Transcription Process
The transcription process is pivotal in the journey of mRNA from genetic material to functional product. During this process, the information encoded within DNA is transcribed to produce messenger RNA (mRNA). This is a key step in the central dogma of molecular biology, wherein DNA serves as a template to create RNA, which subsequently translates into protein. Understanding the transcription process is essential not only for comprehending the mechanics of gene expression but also for exploring its implications in various fields, including biotechnology and medicine.
Transcription involves several critical steps: initiation, elongation, and termination. Each step is orchestrated by a complex interplay of molecules, ensuring accuracy and efficiency in the generation of mRNA. The significance of transcription extends beyond merely copying genetic information; it also offers insight into how genes are regulated, how cellular responses are initiated, and how errors in this process can lead to diseases.
Initiation of Transcription
The initiation phase marks the beginning of transcription and is fundamental for the successful synthesis of mRNA. During this stage, RNA polymerase, the enzyme responsible for synthesizing RNA, locates and binds to a specific region of DNA known as the promoter. The promoter region contains essential signals that compel RNA polymerase to start transcribing the genetic sequence.
Certain transcription factors play a crucial role here. These proteins can either promote or inhibit the recruitment of RNA polymerase to the promoter. Once the transcription factors have correctly aligned with the DNA and the RNA polymerase is attached, the DNA strands unwind, exposing the coding sequence that will be transcribed into mRNA.
Elongation of mRNA Strand
Following the initiation, the elongation phase of transcription proceeds with the RNA polymerase moving along the DNA template. It catalyzes the addition of ribonucleotides complementary to the DNA sequence. As RNA polymerase advances, it unwinds the DNA, synthesizing the mRNA strand by linking together nucleotides in a sequence dictated by the DNA template.
The elongation phase is characterized by high speed and accuracy. RNA polymerase can synthesize RNA at impressive rates, processing hundreds of nucleotides per second in eukaryotic cells. Despite this speed, mechanisms are in place to correct errors during this process, ensuring the fidelity of mRNA synthesis.
Termination of Transcription
Termination of transcription is the final step in the mRNA synthesis process. This phase is triggered when RNA polymerase encounters specific sequences in the DNA known as termination signals. These signals direct RNA polymerase to detach from the DNA template, releasing the newly synthesized mRNA strand.
In eukaryotic cells, the process is a bit more complex. After termination, the mRNA undergoes several modifications, such as capping and polyadenylation, before it can be transported to the cytoplasm for translation. These modifications are essential for the stability, export, and translational efficiency of mRNA.
In summary, the transcription process is a multi-step mechanism that is vital for gene expression. Each phase plays a unique role in ensuring that the genetic blueprint is accurately transcribed into mRNA, setting the stage for subsequent protein synthesis.
Regulation of mRNA Production
Regulating mRNA production is a critical part of cellular function. It governs how the genetic information in DNA is translated into proteins, influencing various biological processes. Precise regulation ensures that the right proteins are produced at the right time and in the right amounts. A disruption in this balance can lead to diseases like cancer and genetic disorders.
There are three main mechanisms through which mRNA production is regulated: transcription factors, epigenetic modifications, and feedback mechanisms. Each plays a unique role in ensuring the cell responds appropriately to internal and external signals.
Transcription Factors
Transcription factors are proteins that bind to specific DNA sequences to control the transcription of genetic information from DNA to mRNA. They can be classified into two categories: activators and repressors. Activators promote transcription, while repressors inhibit it.
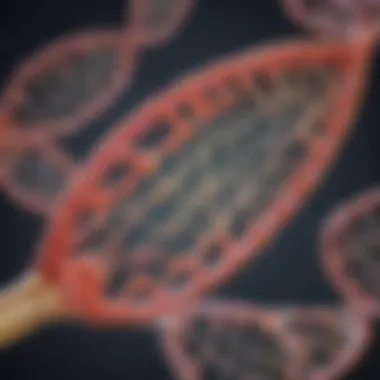
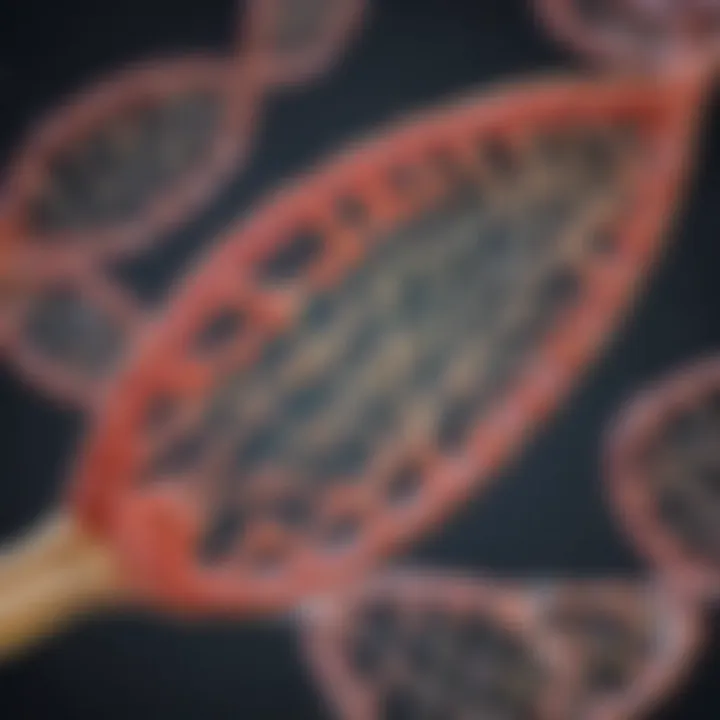
The presence of specific transcription factors can trigger the expression of certain genes, leading to the production of mRNA. This process allows cells to adapt to different conditions. For instance, during stress or changes in nutrient availability, cells can upregulate genes responsible for protective proteins. On the other hand, certain conditions may lead to the suppression of unnecessary gene expression.
Key aspects to consider regarding transcription factors include:
- Specificity: Different tissues express different sets of transcription factors, allowing for specialized functions.
- Interactions: Transcription factors can interact with each other, creating a complex regulatory network that fine-tunes gene expression.
Epigenetic Modifications
Epigenetics refers to changes in gene expression that do not involve alterations to the DNA sequence itself. These modifications can be inherited and are influenced by environmental factors. Two main types of epigenetic changes are methylation and acetylation.
- Methylation: The addition of a methyl group to DNA can silence gene expression, preventing transcription factors from accessing the gene.
- Acetylation: This modification usually results in activation, easing access for transcription machinery to the DNA.
Epigenetic modifications allow for a dynamic response to external stimuli. For example, changes in diet or environmental conditions can lead to reversible modifications in gene expression through these processes.
Feedback Mechanisms
Feedback mechanisms are critical for maintaining cellular homeostasis. Once a sufficient quantity of a certain mRNA or protein is produced, these mechanisms act to inhibit further production. This can occur through various pathways, including:
- Negative feedback: The end product inhibits its own production by interfering with transcription factors or other signaling molecules.
- Positive feedback: In some cases, the product may increase its own production under specific conditions, promoting rapid cell response.
Maintaining a balance through feedback mechanisms is crucial for preventing overexpression of proteins, which can lead to cellular dysfunction.
Understanding the regulation of mRNA production sheds light on the complex interplay between genes and environmental factors. With advances in research, insights into these regulatory mechanisms could lead to novel therapeutic approaches in medicine and biotechnology.
Post-Transcriptional Modifications
Post-transcriptional modifications are critical steps that occur after the initial transcription of pre-mRNA. These modifications play a vital role in preparing the RNA for its final functions, mainly influencing the stability, localization, and translational efficiency of mRNA strands. They are essential for producing functional mRNA molecules and ensuring the fidelity of gene expression. With these modifications, the cells can control when and how much protein is synthesized, adapting quickly to various cellular demands.
Capping and Polyadenylation
Capping involves adding a modified guanine nucleotide at the 5' end of the pre-mRNA transcript. This cap protects the RNA from degradation by exonucleases and serves as a recognition signal for several proteins involved in mRNA processing and translation. Additionally, the cap is necessary for the efficient initiation of translation, marking the mRNA as ready for use by ribosomes.
Polyadenylation occurs at the 3' end of the pre-mRNA, where a stretch of adenine nucleotides is added. This poly(A) tail does several things. It enhances mRNA stability, extends the lifespan of the molecule in the cytoplasm, and aids in the export of mRNA from the nucleus. It is also crucial for translation efficiency, as many translation initiation factors recognize the poly(A) tail and facilitate the assembly of the translation machinery at the start codon.
In summary, capping and polyadenylation are essential processes that set the stage for effective gene expression. They ensure that mRNA remains intact and functional, maximizing the potential for protein synthesis.
Splicing of Pre-mRNA
Splicing is another important post-transcriptional modification that ensures the maturation of pre-mRNA into functional mRNA molecules. Pre-mRNA contains non-coding sequences called introns and coding sequences known as exons. During splicing, introns are removed, and exons are joined to create a continuous coding sequence that can be translated into proteins.
This process is performed by a complex known as the spliceosome, which consists of small nuclear RNAs (snRNAs) and a variety of proteins. The precision of splicing is crucial, as incorrect splicing can lead to the production of nonfunctional proteins or disease states. Therefore, the mechanism of splicing must accurately identify and remove introns while preserving the integrity of the coding sequences.
Moreover, splicing can be regulated. Alternative splicing enables a single gene to produce multiple protein isoforms by varying the combination of exons included in the final mRNA. This adds a layer of complexity to gene expression and allows for the generation of diverse proteins from a limited number of genes.
Through capping, polyadenylation, and splicing, pre-mRNA undergoes significant modifications that ultimately equip the RNA for its important roles in the cell, affecting everything from post-transcriptional regulation to translational dynamics.
mRNA Transport and Localization
Understanding the transport and localization of mRNA is critical for appreciating its role in protein synthesis and the overall function of the cell. Once mRNA is synthesized in the nucleus, it must be accurately transported to the cytoplasm. This transport is not arbitrary; it is highly regulated. Proper localization ensures that the mRNA is available where it is needed for translation, impacting cell efficiency and functionality.
Export from the Nucleus
The journey of mRNA begins in the nucleus where transcription occurs. After the mRNA is synthesized and processed, it needs to exit the nucleus to reach the cytoplasm. This export process primarily takes place through nuclear pores. These pores act as gateways, controlling what enters and leaves the nucleus. The export of mRNA is mediated by proteins known as exportins. They bind with mRNA and facilitate its movement through the nuclear pore complexes.
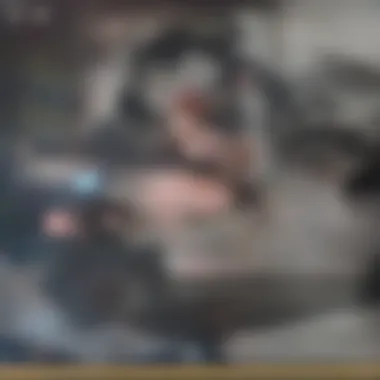
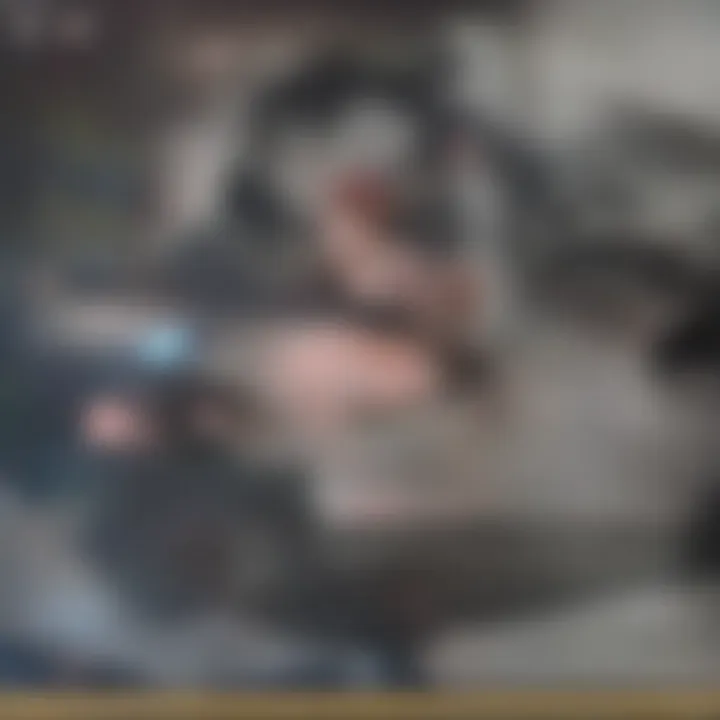
Once mRNA is bound to the exportin, a signal, called the nuclear export signal, becomes critical. This signal ensures that only mature mRNA is exported, preventing premature or defective mRNA from being translated. The energy required for this transport is provided by the GTP-binding protein, Ran. This process is efficient and largely prevents errors. The selective nature of mRNA export underscores its importance in maintaining cellular integrity and functionality.
Localization in the Cytoplasm
After crossing into the cytoplasm, mRNA doesn't simply float freely. Its localization is crucial for ensuring that translation occurs at the right time and place. The cytoplasm is a complex environment with various compartments, and mRNA must reach specific locations to effectively direct protein synthesis.
Several mechanisms dictate mRNA localization in the cytoplasm. The mRNA molecules often contain specific sequences in their untranslated regions, which interact with cytoskeletal elements. These elements assist in guiding mRNA to specific sites within the cell. For instance, dendritic mRNA localization is essential for synaptic function in neurons, impacting learning and memory.
Additionally, localized mRNAs can be stored and translated in response to various signals. This storage can be regulated by proteins that bind to the mRNA, forming structures known as mRNA granules. These granules allow the cell to respond swiftly to changes. The ability to control mRNA localization directly influences the efficiency of protein synthesis, which is fundamental for maintaining cellular homeostasis.
"mRNA transport and localization are indispensable for cellular function, linking transcriptional output to translational success, thus driving cellular processes."
In summary, mRNA transport from the nucleus and its subsequent localization in the cytoplasm represent critical stages in the life of an mRNA molecule. These processes are essential for ensuring that the right proteins are made at the right time and place within the cell.
mRNA and Biotechnology
mRNA plays a crucial role in biotechnology, serving as a fundamental component for a variety of applications. Understanding how mRNA functions provides significant insights that drive innovation in fields such as vaccine development, gene therapy, and personalized medicine.
One remarkable application of mRNA technology is its use in the development of vaccines, particularly highlighted during the COVID-19 pandemic. The Pfizer-BioNTech and Moderna vaccines utilize synthetic mRNA to instruct cells to produce a harmless piece of the virus’s spike protein. This builds an immune response without exposing individuals to the actual virus. The speed and effectiveness of utilizing mRNA for vaccine technology showcase its transformative potential in the face of public health challenges.
In addition to vaccines, mRNA technology has significant implications for gene therapy. Here, synthetic mRNA can be designed to replace or supplement malfunctioning genes. It essentially becomes a vehicle for delivering therapeutic proteins inside target cells. This strategy holds immense promise for treating genetic diseases and disorders where direct gene editing is challenging.
Applications of mRNA Technology
- Vaccine Development: As mentioned, mRNA vaccines are a recent breakthrough. They provide rapid development times compared to traditional methods. Researchers can quickly modify the mRNA sequence to target new pathogens.
- Cancer Immunotherapy: mRNA can stimulate the immune system to recognize and attack cancer cells. By encoding tumor-specific antigens, therapies can leverage the body’s immune response to fight malignancies.
- Protein Replacement Therapy: This use case addresses diseases caused by protein deficiencies. By delivering the right mRNA, patients can produce necessary proteins that their bodies lack.
- Diagnostic Tools: mRNA can be harnessed in diagnostics, where the presence of specific mRNA sequences can indicate diseases, including infections and cancers, thereby improving early detection methods.
Future Directions in mRNA Research
The future of mRNA research is promising, with various avenues being explored to enhance its efficacy and expand its applications. Key areas of focus include:
- Enhanced Stability: Researchers are investigating methods to improve the stability of mRNA molecules in the bloodstream. Such advancements can lead to longer-lasting therapeutic effects.
- Targeted Delivery Systems: Developing more effective delivery systems for mRNA is vital. Lipid nanoparticles are currently used, but alternative carriers might optimize delivery to specific cells and tissues.
- Expanding Therapeutic Areas: Future research is likely to explore the application of mRNA beyond vaccines and cancer. This includes autoimmune diseases and metabolic disorders.
- Informed Consent and Ethical Considerations: As mRNA applications expand, discussions around informed consent and ethical implications of gene therapy will become increasingly important.
"The innovative use of mRNA technology signifies a pivotal shift in the landscape of biotechnology, leading to remarkable advancements in medicine and health."
As we delve deeper into the potentials of mRNA, it is essential to consider both the scientific and ethical paradigms that will shape its trajectory in the coming years.
Culmination
The study of mRNA synthesis and its cellular mechanics is essential for understanding many biological processes. This conclusion draws attention to several key aspects discussed in this article. First, the importance of mRNA cannot be overstated. It serves as the intermediary that carries genetic information from DNA to ribosomes, where proteins are synthesized. Recognizing this function illuminates the pathways of gene expression and regulation, providing insights into how cells maintain their functions and respond to their environment.
Understanding mRNA contributes significantly to the field of molecular biology. Researchers focus on how disruptions in the mRNA processes can lead to diseases. For instance, mutations in mRNA or the pathways governing its production can cause severe health conditions. Such knowledge is vital for developing targeted therapies and diagnostic tools.
The Importance of mRNA Understanding
Understanding mRNA and its synthesis enhances our grasp of various biological phenomena. When we delve into this subject, we realize that mRNA is not a mere messenger; it is a crucial player in the intricate game of gene regulation and protein synthesis. The mechanisms that control mRNA transcription, modification, and translation are tightly regulated, reflecting the cell's needs. Consequently, any alteration in these processes can lead to maladaptive cellular responses.
The interplay of mRNA with transcription factors and its post-transcriptional modifications are of paramount interest. By analyzing these interactions, scientists can decipher how genes are turned on and off and how cells respond to external signals. This understanding is particularly beneficial in areas such as developmental biology, where the timing and regulation of gene expression are crucial.
Thus, a detailed comprehension of mRNA biology not only enriches our knowledge of basic cellular functions but also contributes to advances in various scientific disciplines.
Implications for Science and Medicine
The implications of mRNA understanding are profound, extending well into the realms of science and medicine. Advances in mRNA research are paving the way for groundbreaking therapeutic strategies. For example, mRNA vaccines, such as those developed for COVID-19, highlight the application of this knowledge in real-world contexts. These vaccines utilize synthetic mRNA to instruct cells to produce a harmless piece of the virus, stimulating an immune response without causing the disease itself.
Moreover, mRNA-based therapies hold promise for treating a range of diseases, including genetic disorders and cancers. Researchers are exploring ways to utilize mRNA to produce therapeutic proteins that can correct cellular deficiencies or target specific pathological processes. This innovative approach could provide hope where traditional therapies have failed.
In addition, mRNA's role in gene editing technologies, such as CRISPR, underscores its significance. These technologies rely on RNA molecules to guide the editing process, making them powerful tools for manipulating genetic material and correcting mutations.
As we advance our understanding of mRNA, the potential applications in science and medicine continue to expand, promising exciting developments in the future.