Exploring the Significance of mRNA Complementary Codons
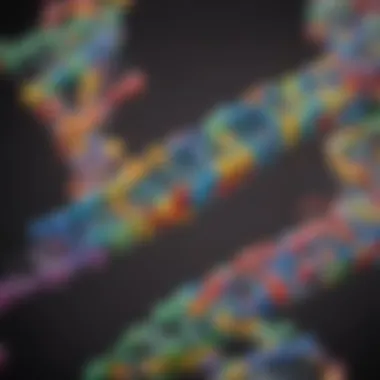
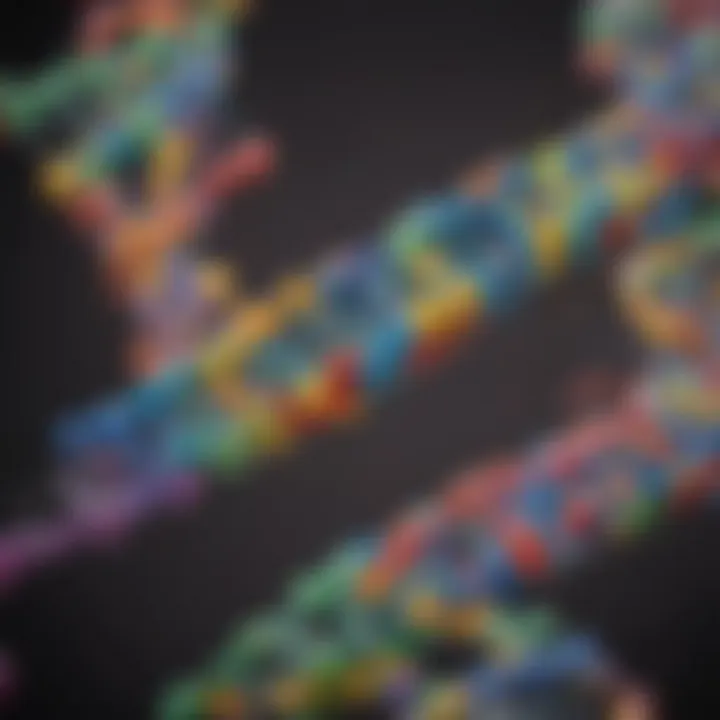
Intro
mRNA complementary codons are a critical component of the protein synthesis process. They play an essential role in translating genetic information into functional proteins. Understanding them is vital for students, researchers, educators, and professionals interested in molecular biology. This section lays the groundwork for a more detailed exploration of codons, their structure, function, and significance in genetic expression.
Codons are sequences of three nucleotides found in messenger RNA (mRNA). Each codon specifies a particular amino acid or signals the termination of protein synthesis. This specificity is crucial, as the overall function of proteins is determined by their amino acid sequence. The reading of codons occurs within the ribosomes, where transfer RNA (tRNA) molecules bring the corresponding amino acids as directed by the mRNA sequence. In many ways, the process is a finely tuned orchestration that underpins cellular function.
In this article, we will delve into the key elements of mRNA codons, revealing their complex structures and the essential roles they play in the machinery of life. The detailed exploration will encompass how codons are read, the implications of codon usage across different organisms, and potential applications in various biotechnological fields.
The significance of this topic extends beyond academic interest; it has real-world implications in medicine, genetics, and biotechnology. As we aim to enhance understanding of mRNA codons, we will highlight discoveries, discuss potential applications, and explore future research directions. By doing so, we encourage a deeper appreciation of how genetic information translates into the biological functions that sustain life.
Intro to mRNA and Codons
The study of mRNA and codons serves as a foundation for understanding cellular processes essential for life. mRNA, or messenger RNA, plays a critical role in conveying genetic information from DNA to the ribosomes, facilitating protein synthesis. This process is fundamental to various biological functions, making the exploration of codons vital for students, researchers, and professionals across various fields.
What is mRNA?
Messenger RNA is a single-stranded molecule that carries genetic instructions from DNA in the nucleus to ribosomes in the cytoplasm. Unlike DNA, which is a double helix, mRNA is more dynamic, allowing it to serve as a template for translating genetic information into proteins. This translation process is central to gene expression and regulation. mRNA consists of nucleotides arranged in a specific sequence that corresponds to the genetic code determined by the DNA.
Defining Codons
Codons are triplets of nucleotides within mRNA that encode for specific amino acids, the building blocks of proteins. Each codon consists of three of the four nucleotide bases (adenine, cytosine, guanine, and uracil). The interplay between different codons allows for the synthesis of a vast array of proteins, each with unique functions and structures. Understanding codons is critical, as they directly determine the amino acid sequence in proteins.
Importance of Codons in Protein Synthesis
Codons are indispensable for the process of translation. Ribosomes read the mRNA sequence in sets of three nucleotides, identifying the corresponding amino acids using codon tables. This reading frame ensures accurate translation of genetic information into functional proteins.
Furthermore, codons are essential for maintaining the integrity and fidelity of protein synthesis. Any mutation in the codon sequence can lead to the production of faulty proteins, which may result in cellular dysfunction or disease. Thus, a comprehensive understanding of codons enables insights into not only fundamental biology but also the mechanisms underlying various genetic disorders.
Codons are not merely biological symbols; they are the very language of life, dictating how proteins are formed and function within the cellular milieu.
In summary, an exploration of mRNA and codons reveals the intricate mechanisms that sustain life, emphasizing their role in protein synthesis and genetic expression.
Structure of mRNA Codons
Understanding the structure of mRNA codons is essential in grasping how genetic information is translated into functional proteins. Codons, being sequences of nucleotides, serve as the fundamental building blocks in this translation process. Each codon consists of three nucleotide bases, which correlates directly to a specific amino acid or a functional stop signal during protein synthesis.
The composition and arrangement of these nucleotide sequences have direct implications for both the efficiency and accuracy of protein production. A detailed investigation into the various components of codons provides insight into how genetic codes translate into biological roles in cellular activity and development.
Nucleotide Composition
Each codon is made up of a combination of four nucleotide bases: adenine (A), cytosine (C), guanine (G), and uracil (U). The unique arrangement of these bases determines the specific instructions encoded within the mRNA, as they correspond to amino acids. The redundancy of the genetic code is noteworthy; multiple codons can code for the same amino acid.
This characteristic allows for flexibility in genetic coding, minimizing the potential impact of mutations. For example, the amino acid leucine can be encoded by six different codons. This redundancy not only illustrates the robustness of the genetic code but also emphasizes the importance of nucleotide composition in maintaining organismal integrity.
Triplet Nature of Codons
The triplet nature refers to the grouping of nucleotides into sets of three. Each triplet, or codon, specifies a particular amino acid in the protein synthesis process. The requirement for three nucleotides arises because there are only four different bases available. Consequently, to cover the 20 standard amino acids used in protein synthesis, a minimum of 64 unique combinations is necessary.
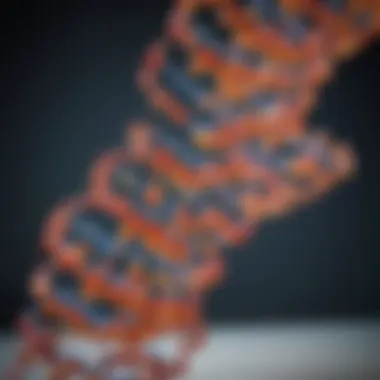
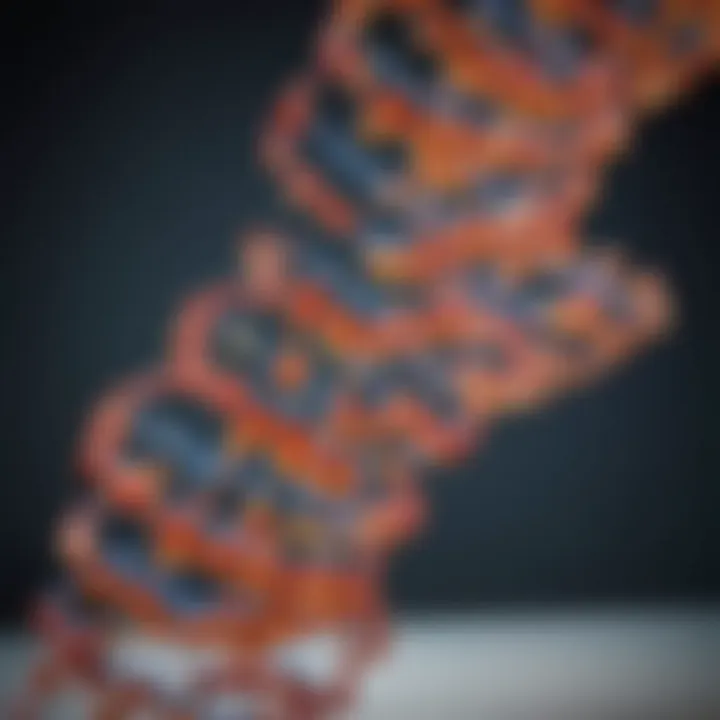
This triplet arrangement is fundamental in explaining how languages of nucleotides translate into the language of proteins. The reading frame in mRNA must be established correctly, as any shift can lead to vastly different sequences of amino acids, potentially changing a harmless protein into a toxic one.
Start and Stop Codons
Start and stop codons are integral to the translation process. The start codon, which is AUG, not only signals the beginning of translation but also codes for the amino acid methionine. This particular codon sets the reading frame for subsequent codons, ensuring that the protein is synthesized in the correct sequence.
On the opposite end, stop codons are critical for termination of protein synthesis. These codons do not code for any amino acids but signal the ribosome to halt translation. Key stop codons include UAA, UAG, and UGA.
Important Note: The presence of start and stop codons makes reading mRNA a well-defined process.
The Mechanism of Translation
Translation is a crucial process in the realm of molecular biology. It serves as the mechanism through which the genetic code contained in mRNA is deciphered to produce proteins. The fundamental nature of translation involves several key elements that collaborate to ensure that proteins are synthesized accurately and efficiently. This section underscores how translation operates and its relevance to understanding mRNA codons in detail.
Role of Ribosomes
Ribosomes are large molecular machines that play a primary role in protein synthesis. They are composed of ribosomal RNA and proteins, forming a complex structure. Ribosomes can be found either freely floating in the cytoplasm or bound to the endoplasmic reticulum, where they are often referred to as rough ER.
The structure of the ribosome consists of two subunits, a larger and a smaller, which come together during translation. The smaller subunit is in charge of reading the mRNA sequence. It ensures that the correct codon is matched with its complementary tRNA. The larger subunit facilitates the formation of peptide bonds between amino acids, thereby building the polypeptide chain that will eventually fold into a functional protein.
Ribosomes also have specific sites where the tRNA molecules attach. The A site, P site, and E site are critical for the translation process. The A site receives the incoming tRNA, the P site holds the tRNA that carries the growing polypeptide chain, and the E site is where the empty tRNA exits the ribosome.
"Ribosomes are essential to the translation process; without them, the synthesis of proteins would not occur."
tRNA and Anticodons
Transfer RNA (tRNA) acts as the adaptor molecule during translation. Each tRNA molecule carries a specific amino acid that corresponds to a triplet codon in the mRNA. The key feature of tRNA is the anticodon, a set of three nucleotides that is complementary to the codon on the mRNA strand. This complementary pairing is essential for the correct amino acid to be added to the growing polypeptide.
The structure of tRNA is distinct, with a cloverleaf shape that allows it to efficiently deliver the amino acid to the ribosome. Each tRNA is specific to an amino acid, and thus, multiple tRNAs exist to ensure every codon in the mRNA can be translated. This specificity plays a vital role in maintaining the fidelity of protein synthesis.
Translation Process
The translation process can be divided into several stages: initiation, elongation, and termination.
- Initiation: This stage begins when the ribosome assembles around the mRNA strand. The first tRNA, carrying methionine, binds to the start codon, usually AUG.
- Elongation: During elongation, the ribosome travels along the mRNA, reading codons in sequence. For each codon read, the corresponding tRNA brings an amino acid to the A site. A peptide bond forms between the amino acids at the P site and A site. After the bond forms, the ribosome shifts one codon down the mRNA, allowing the process to repeat until a complete polypeptide chain is formed.
- Termination: This stage occurs when a stop codon is reached. Since stop codons do not have corresponding tRNAs, the process ceases. Release factors bind to the ribosome, prompting the release of the completed polypeptide chain, which will soon fold into a functional protein.
In summary, the translation process is intricate and finely tuned. The collaboration of ribosomes, tRNA, and codons ensures that genetic information is effectively and accurately translated into proteins, the building blocks of life.
Codon-Amino Acid Relationship
Understanding the relationship between codons and amino acids is critical for grasping how genetic information is translated into functional proteins. Each codon, consisting of three nucleotides, corresponds to a specific amino acid or a stop signal during protein synthesis. This relationship adds another layer of complexity to the genetic code, emphasizing the precision needed during translation.
Codon Tables
Codon tables serve as essential tools in molecular biology. They provide a comprehensive mapping of codons to the amino acids they encode. The standard genetic code consists of 64 codons, which include 61 that code for amino acids and 3 that signal termination of translation. Different organisms may have variations in their codon usage, but the core table remains relatively consistent across species.
For example, the codon AUG not only codes for Methionine but also serves as the start codon. The codon tables present each triplet and the corresponding amino acid, making it easier to understand protein synthesis and predict the sequence of amino acids in a polypeptide.
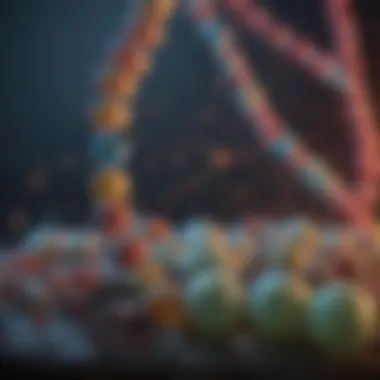
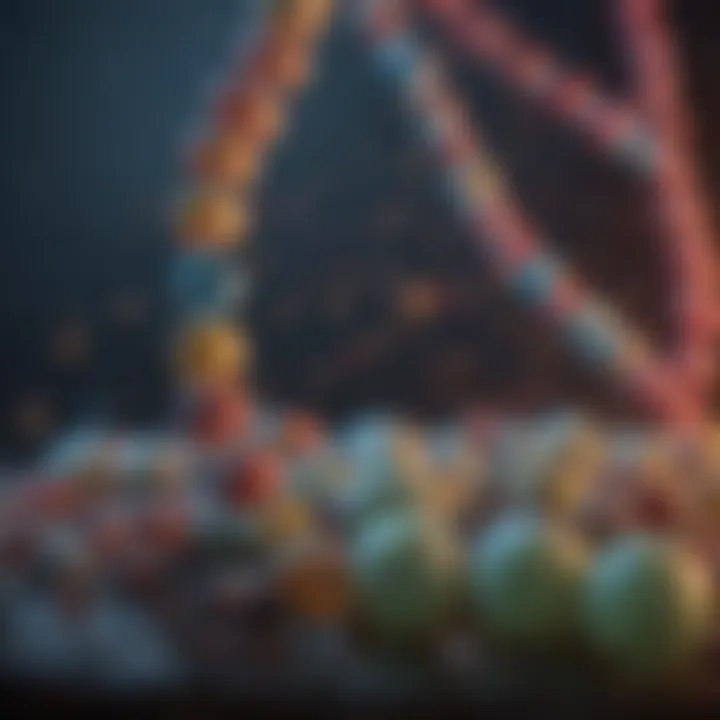
Key Points:
- Codons are triplet sequences of nucleotides.
- 64 possible codons map to 20 distinct amino acids.
- Codon tables facilitate quick reference for researchers.
Degeneracy of Codons
The degeneracy of the genetic code refers to the fact that multiple codons can encode the same amino acid. For instance, the amino acid Leucine can be coded by six different codons: UUA, UUG, CUU, CUC, CUA, and CUG. This redundancy is advantageous. It allows for some tolerance in mutations that may occur in DNA. Such mutations could result in a change in base pairs while still coding for the same amino acid, thus minimizing the impact on the protein structure and function.
The degeneracy of codons is not uniform; some amino acids are coded by more codons than others. Understanding this feature is vital for genetic engineering and synthetic biology, where the design of codon-optimized sequences can enhance protein production in host organisms.
Impact on Protein Structure
The relationship between codons and the resulting amino acids has profound implications for protein structure and function. The sequence of amino acids dictates the protein's folding and stability, which in turn determines its functionality within the cell. A single change in a codon can have cascading effects on the final protein product. This concept is particularly crucial when considering how genetic variations can lead to diseases.
Moreover, the way codons are used can affect not just the sequence but also the kinetics of translation. For example, codons that are less frequently used may slow down the translation process, impacting the overall protein synthesis rate. Understanding these dynamics is essential for researchers in the fields of biochemistry and molecular biology.
"The codon-amino acid correlation is foundational for understanding biological processes at a molecular level. Without it, the complexities of life would remain obscured."
Evolutionary Perspectives
Understanding the evolutionary perspectives of mRNA complementary codons provides insight into how genetic information is conserved and adapted across various species. The significance of this analysis lies in its ability to explore how different organisms utilize codons for optimal protein synthesis, and how these preferences may influence evolutionary trajectories. This examination of codon usage bias and comparative genomics reveals critical aspects of molecular biology and the evolutionary mechanisms impacting genetic expression.
Codon Usage Bias
Codon usage bias refers to the preferential use of certain codons over others in the coding sequences of organisms. This phenomenon can greatly affect gene expression levels, as not all codons translate with the same efficiency. For instance, the species-specific prevalence of synonymous codons—codons that encode the same amino acid but differ in sequence—can lead to variation in protein production.
In different organisms, such as Escherichia coli or human cells, certain codons may be favored due to the abundance of corresponding tRNA molecules. This bias enhances the speed and accuracy of translation. It's essential to consider the implications of codon usage bias on evolutionary adaptability. Species that can optimize their codon usage are often better at reacting to environmental changes.
- Benefits of Codon Usage Bias:
- Increased translation efficiency
- Enhanced fitness in specific environments
- Regulation of gene expression
Observed biases can also indicate evolutionary pressures, where certain codons are selected for based on their necessity in protein function. This aspect provides a lens through which to view evolutionary history and biological diversity.
Comparative Genomics
Comparative genomics involves analyzing the genome sequences of different species to identify genetic similarities and differences. This analysis is paramount in understanding codons from an evolutionary viewpoint. It allows us to reconstruct the evolutionary relationships among organisms and understand how codon usage varies.
By comparing genomes, scientists can pinpoint conserved regions that are crucial for essential functions. This can reveal how certain codon sequences have been maintained through evolutionary time. Different organisms often develop distinct codon preferences in response to their environments; understanding these patterns sheds light on evolutionary pressures.
The study of codon usage and comparative genomics illustrates the dynamic interplay between genetic code and evolutionary adaptation, offering insights into molecular biology.
Applications in Biotechnology
The significance of mRNA complementary codons extends beyond the realm of basic molecular biology. Their applications in biotechnology represent a frontier where genetic understanding marries practical utility. The mechanisms of protein expression lead to advancements in numerous fields, from agriculture to medicine. Codons play a pivotal role in the design of genetic constructs that enable the manipulation of biological systems. Let's explore key areas in this context.
Genetic Engineering
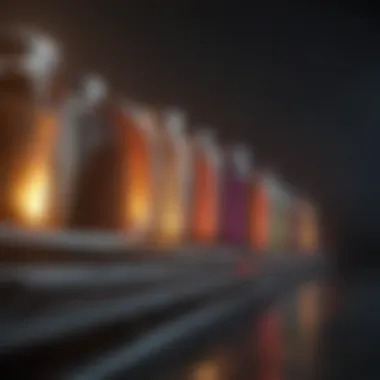
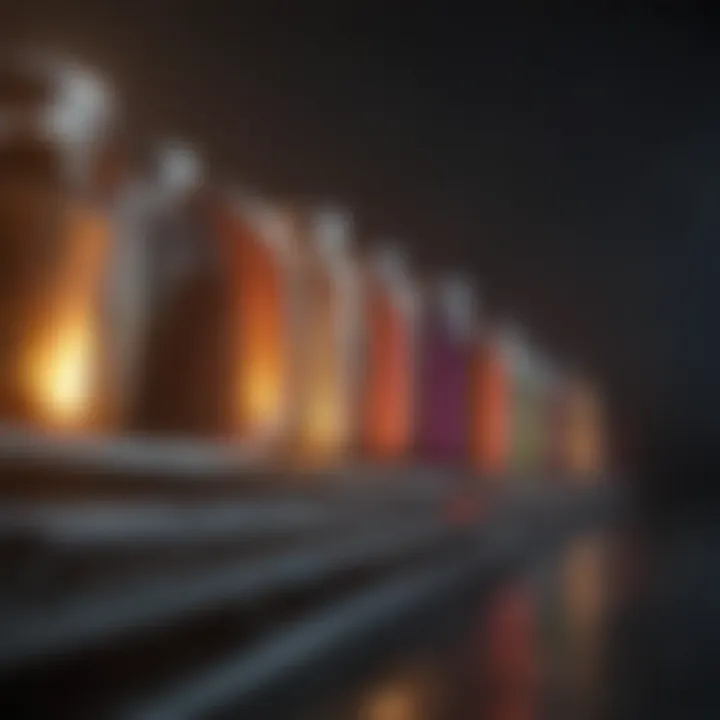
Genetic engineering relies heavily on precise understanding of mRNA codons. Since codons determine the amino acid sequences in proteins, inaccuracies here can result in non-functional or harmful proteins. In biopharmaceutical production, engineered microbes or plants need to express proteins with particular functionality—be it enzymes, antibodies, or therapeutic agents. Creating codon-optimized genes enhances translation efficiency in host organisms. This optimization can lead to higher yields in protein production.
For instance, E. coli is often used as a host for recombinant protein expressions. By modifying the mRNA sequence to favor codon usage that matches E. coli's preference, the result can be a significant increase in protein output. Considerations such as the degeneracy of codons and their context-dependent expression are critical when designing these genetic constructs. Understanding the codon table for specific species allows scientists to predict how well the genetic material will perform within different biological systems.
Synthetic Biology
Synthetic biology merges engineering principles with biology, utilizing codon manipulation as a fundamental tool. Creating new biological systems or redesigning existing ones involves assembling sequences of DNA that align with desired codon specifications. This field can address challenges in medicine, energy, and environmental sustainability.
Synthetic biologists design custom organisms that can produce biofuels or even secrete antibiotics. For these systems to work efficiently, the associated mRNA must be carefully tuned. For example, the construction of a biosensor that detects pollutants might involve integrating codons that initiate expression of reporter genes in response to a specific stimulus.
By employing codon optimization, synthetic biology can thus create life-like systems with specific functions. This has deep implications for developing therapeutics, where engineered organisms could continuously produce necessary compounds or degrade harmful substances. The versatility of codons allows for complex signaling and regulatory circuits to be established, reflecting their importance in the biotechnological exploration of synthetic life forms.
The interplay between codons and biotechnology showcases how fundamental genetic understanding can lead to revolutionary applications in diverse fields.
Health Implications
Understanding the health implications of mRNA complementary codons is crucial, particularly in the landscape of modern medicine and genetics. This section delves into the various ways in which codons can influence health, disease mechanisms, and therapeutic interventions.
Role in Disease
mRNA codons play a pivotal role in the development of various diseases. Mutations in the codons can lead to incorrect amino acids being incorporated into proteins, which can subsequently alter protein structure and function. This misincorporation may contribute to diseases such as cancer, cystic fibrosis, and certain neurodegenerative disorders.
For instance, in some cancers, specific point mutations in codons can lead to the production of overactive oncogenes or inactive tumor suppressor proteins. This shift in protein function creates an environment conducive to uncontrolled cell growth. Understanding the relationship between codon mutations and these diseases can aid in identifying genetic predispositions and developing targeted therapies.
Moreover, the study of codons reveals insights into the biological processes of diseases. Researchers focus on how certain codons might affect gene expression levels and protein folding. Such insights help unravel the complexities of protein synthesis and its direct impact on health.
"Investigation of mRNA codon mutations not only highlights critical pathways in disease progression but also opens doors for novel treatment strategies."
Therapeutic Applications
The therapeutic applications of understanding mRNA codons are vast and continually expanding. One prominent area involves the development of mRNA vaccines, which utilize codons to code for specific antigens. These vaccines can stimulate an immune response without causing disease, exemplified by the Pfizer-BioNTech and Moderna COVID-19 vaccines that use mRNA technology.
Furthermore, gene therapy strategies are leveraging our knowledge of codons. By designing sequences that carry correct codons, researchers can potentially correct genetic disorders at their source. This approach aims to replace faulty genes and adjust protein synthesis to restore normal physiological functions.
Some potential benefits and considerations of employing codon-focused therapies are:
- Precision: Targeting specific codon mutations can lead to more effective treatments.
- Personalization: Therapies can be tailored to individual genetic makeups.
- Speed: mRNA-based therapies can be developed more rapidly compared to traditional methods.
End
The conclusion of this article encapsulates the importance of mRNA complementary codons in understanding protein synthesis. By illustrating how codons operate, we highlight their foundational role in translating genetic information into functional proteins. This process is crucial for all cellular activities and impacts various biological functions. Codons enable the determination of amino acid sequences, affecting protein structure and function.
Summary of Key Points
- Codons Read by Ribosomes: mRNA codons are essential for the accurate translation process in cells. Ribosomes utilize these codons to synthesize proteins based on the genetic blueprint.
- Codon-Amino Acid Relationship: Each codon corresponds to a specific amino acid, underscoring the linear translation of genetic information into protein primary structure.
- Implications in Health and Disease: Understanding codons contributes to insights into genetic disorders and opens avenues for therapeutic applications.
- Biotechnological Applications: The knowledge of codon function is pivotal in synthetic biology and genetic engineering, leading to innovations in medicine and agriculture.
Future Directions in Research
The landscape of research surrounding mRNA complementary codons is vibrant and full of potential. This area holds many possibilities:
- Exploration of Codon Bias: Investigating how different organisms favor certain codons over others could deepen our understanding of evolutionary biology.
- Therapeutic Innovation: Further studies in codons can lead to new drugs targeting specific proteins involved in diseases.
- Synthetic Biology Advances: As biotech progresses, optimal codon usage and design will be crucial for developing more efficient biological systems.
- Environmental Impact: CODON research could influence projects aimed at genetic modification of plants for sustainability and resilience against climate change.
Understanding mRNA codons is not just an academic exercise; it bears significant implications for biology, medicine, and our treatment of various medical conditions.