Mastering PCR Primer Annealing Temperature
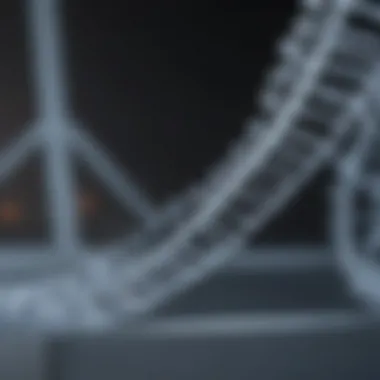
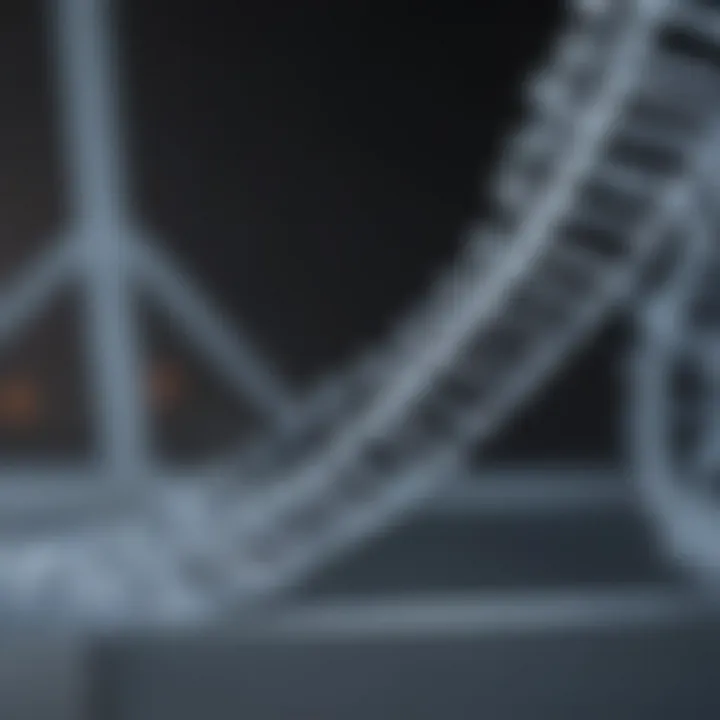
Intro
Polymerase chain reaction (PCR) is a cornerstone technique in molecular biology, enabling the amplification of specific DNA sequences with remarkable precision. At the core of this method lies the primer annealing temperature, a parameter that can determine the success of an experiment. Understanding the intricacies of this temperature is crucial for researchers seeking to amplify DNA effectively and efficiently.
When performing PCR, primers serve as the starting points for DNA synthesis. Their binding affinity to the target DNA strands is influenced heavily by the temperature at which they hybridize to their respective sequences. If the annealing temperature is too high, the primers may not bind adequately, failing to initiate the amplification process. Conversely, if it's too low, the risk of non-specific binding increases; meaning that the primers could latch onto unintended DNA regions, creating a chaotic mix of products that can muddle results.
Navigating through the temperature ranges involves understanding the basic principles of molecular interactions and enzyme kinetics. The knowledge gained from this concept can lead to significant advancements in genetic research and practical applications, whether in clinical diagnostics, forensic analysis, or biotechnology.
The importance of achieving the right balance in annealing temperature can't be overstated; it not only enhances specificity but also influences the yield of amplicons. This article aims to illuminate this critical aspect, guiding readers through the biochemical principles of primer design, methods for calculating optimal temperatures, and practical considerations that contribute to successful PCR experiments.
Prelims to PCR and Its Importance
Polymerase Chain Reaction (PCR) has evolved into a cornerstone technique in molecular biology, revolutionizing the ways researchers can amplify specific DNA sequences. In a nutshell, PCR is akin to turning up the volume on a whispering conversation; it takes a minute segment of DNA and makes millions of copies, thus allowing for detailed analysis. The significance of annealing temperature in PCR cannot be understated. Just as a locked door requires the correct key to open, the success of PCR hinges on precisely setting the annealing temperature of primers used in the amplification process.
In the sections that follow, we will explore the intricate details surrounding the polymerase chain reaction. Understanding how primer properties and reaction conditions interplay sets the stage for optimizing PCR. With this knowledge, students, researchers, and professionals can approach their experiments with confidence, knowing they have a strong grasp on the foundations of PCR and its impact.
Overview of Polymerase Chain Reaction
The Polymerase Chain Reaction is a technique developed in the 1980s by Kary Mullis, who realized that it was possible to amplify a specific DNA sequence exponentially. This is accomplished through a series of temperature-controlled steps: denaturation, annealing, and extension. During denaturation, the double-stranded DNA is heated to separate the strands, akin to how boiling water transforms an egg's structure. In the following step, annealing, the temperature is lowered to allow primers to attach to the target DNA sequence. Finally, during extension, a heat-stable DNA polymerase synthesizes new DNA strands from the primers.
This cycle is repeated numerous times—typically 25 to 35 cycles—to achieve significant amplification. The beauty of PCR lies in its precision and versatility, allowing it to be utilized across various fields, from genetics to forensics. It is not limited to research, as its applications extend into medicine, environmental science, and beyond.
Applications of PCR in Research and Medicine
PCR is more than just a lab technique; it is a lifeline in many fields. In research, it enables scientists to analyze gene expression, identify genetic mutations, and even clone DNA sequences for further study. For example, a biologist studying a specific gene related to a hereditary disease can use PCR to amplify that gene directly from a patient’s sample, enabling deeper insight into genetic conditions.
In medicine, PCR plays a pivotal role in diagnostics. Pathogens can be identified quickly through specific target sequences, with the ability to detect viral infections such as HIV or COVID-19 within hours rather than days. It’s not just about speed but also about accuracy, as PCR ensures that minute amounts of viral DNA are detected, leading to prompt treatment decisions.
Furthermore, PCR can be utilized in prenatal testing to identify genetic disorders, showcasing its crucial role in modern healthcare. The significance of this technology extends into legal forensics as well, where it is instrumental in DNA profiling to aid in criminal investigations.
"PCR is a game changer when it comes to understanding the genetic blueprint of life itself."
By navigating through these varied applications, it becomes abundantly clear that mastering the concepts surrounding annealing temperature and other PCR parameters is not only beneficial but essential for achieving precise results across numerous scientific endeavors.
Fundamentals of Primer Design
The design of primers is a cornerstone in the polymerase chain reaction (PCR) process. Without well-thought-out primers, all your efforts to amplify specific DNA sequences might just end up as an exercise in frustration. Understanding the fundamentals of primer design means delving into how primers function, how they are crafted, and the critical characteristics that separate good primers from great ones. This section aims to illuminate why these principles matter not just in theory, but in the practice of molecular biology and genetic research.
Understanding Primers and Their Role
Primers are short strands of nucleotides that provide a starting point for DNA synthesis. They anneal to the template DNA and serve as a foundation for the DNA polymerase to build upon. In effect, primers are the unsung heroes of PCR; without them, the process simply wouldn't occur.
Let’s break it down. In a PCR experiment, two primers are typically used. They flank the target DNA sequence, ensuring that DNA polymerases can extend from these primers. The specificity of your PCR reaction heavily relies on the proper design of these primers, as they dictate the region of DNA to be amplified. If they’re too similar to unintended targets, trouble may loom, leading to non-specific amplification and a muddled result.
When designing primers, it’s crucial to consider their length, melting temperature, and how they interact with the target sequence. A set of well-designed primers can forge the path to accurate and reliable results in PCR.
Key Characteristics of Effective Primers
When it comes to primer design, a few essential characteristics can uplift your PCR game:
- Length: Primers are commonly between 18 to 25 bases long. Shorter primers may anneal too weakly and contribute to poor specificity, while longer primers can amplify more specific targets. A balanced length is fundamental to achieving reliable amplification.
- GC Content: The percentage of guanine (G) and cytosine (C) in your primers can influence their stability and binding properties. An optimal GC content typically ranges from 40% to 60%. Higher GC pairs form three hydrogen bonds compared to two hydrogen bonds from AT pairs, resulting in greater stability.
- Melting Temperature (Tm): This is the temperature at which half of the DNA strands are in double helix form and half are single stranded. Ideally, Tm should be similar for both primers in a pair to ensure that they anneal effectively at the same temperature during PCR. A gap of more than 5°C in Tm can lead to uneven amplification and, ultimately, less reliable results.
- Specificity and Uniqueness: Primers should target unique sequences in the template DNA to avoid non-specific binding. Ensuring that your primers don’t bind to regions other than the intended target is paramount to maintain the accuracy of PCR.
- Avoid Secondary Structures: Hairpins, dimers, or other secondary structures can interfere with the annealing process. Effective primers are designed to minimize these unintended formations, promoting the best chance for proper binding.
"A well-designed primer can make all the difference between a successful PCR and a failed experiment."
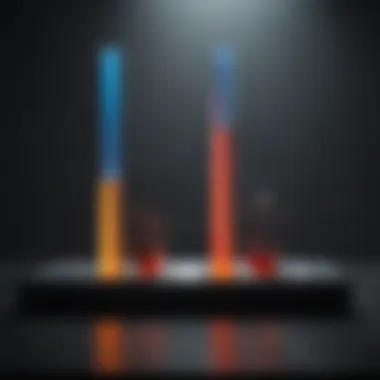
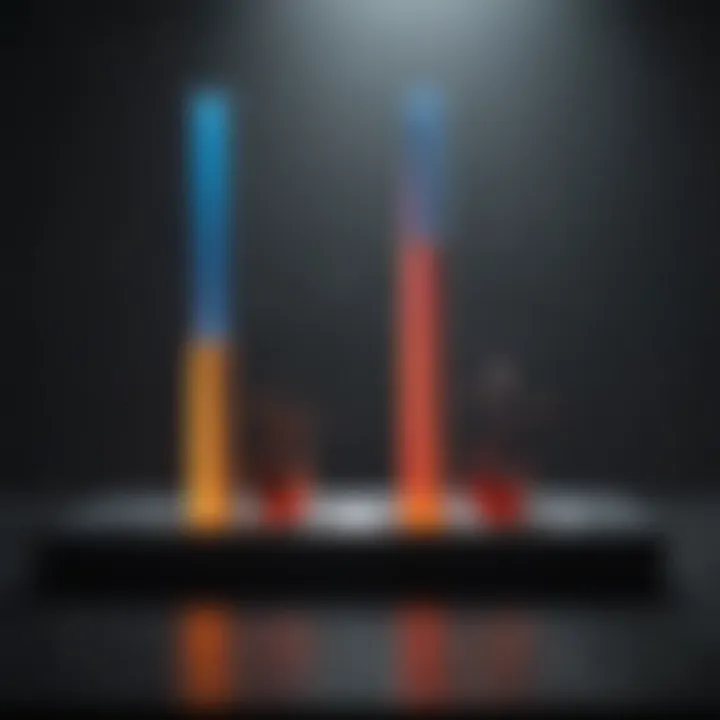
Effectively understanding these parameters can shape the outcome of your PCR experiments. Whether you're diving into research work or developing diagnostic tools, the principles of primer design cannot be disregarded—getting them right means you pave the way for fruitful results in molecular biology.
The Concept of Annealing Temperature
The annealing temperature, a cornerstone in PCR (Polymerase Chain Reaction) protocols, is fundamental to the success of DNA amplifications. As the process of PCR hinges on the specificity of primers binding to their complementary DNA sequences, the right temperature ensures that primers latch on efficiently and effectively. If the temperature is too low, non-specific binding can occur, leading to unwanted products; conversely, if it’s too high, primers may fail to bind altogether. This delicate balance makes it crucial for researchers and practitioners to comprehend the nuances of annealing temperature in their experiments.
Definition and Significance
Annealing temperature refers to the specific temperature at which primers anneal or bind to their target DNA sequences during the PCR cycle. This step comes after the denaturation phase, where the double-stranded DNA is unwound into single strands. The significance lies in its direct impact on the efficiency and specificity of the amplification process. A well-optimized annealing temperature improves the yield of the target DNA while minimizing background noise from non-specific products.
Efficient binding at the correct temperature means that the primers will attach only to the sequences they are supposed to amplify, leading to cleaner results and a more reliable understanding of the template DNA. A failure to identify the proper annealing temperature could spell trouble, resulting in either minimal amplification or undesired artifacts.
"The fine line between optimum results and trial-and-error often boils down to pinning the correct annealing temperature."
Factors Influencing Annealing Temperature
Determining the appropriate annealing temperature isn't just a shot in the dark; several factors come into play that can tilt the balance one way or the other. These include:
Length of Primers
The length of the primers you choose is directly proportional to their annealing temperature. Typically, longer primers (20-30 bases) anneal at higher temperatures than shorter ones (10-15 bases). This increase in temperature aligns with the principle that longer sequences provide more hydrogen bonds between the primer and the target DNA, stabilizing the complex.
A critical characteristic of longer primers is their ability to enhance specificity, meaning that they are less likely to bind to non-target sequences. This precision is vital in studies where accurate amplification matters immensely, such as in forensic analysis or genetic diagnostics. However, there is a trade-off with increased length as longer primers might also lead to increased costs and complications in design.
GC Content
GC content, or the ratio of guanine (G) and cytosine (C) bases in a DNA sequence, also plays a pivotal role in determining the annealing temperature. GC pairs form three hydrogen bonds compared to two bonds for AT pairs, making GC-rich primers more stable.
For example, primers with a GC content of 60% might require a higher annealing temperature than those with a 40% GC content. This characteristic is favorable because it contributes directly to the specificity of the binding, reducing the likelihood of non-specific amplification. It must be noted, however, that extremely high GC content can also lead to hairpin structures or self-dimers, which hinder amplification efficiency.
Presence of Additives
Additives, such as dimethyl sulfoxide (DMSO) or glycerol, can modify the behavior of primers during the annealing phase. These substances often help in stabilizing the reaction, making it easier for primers to bind even in challenging conditions.
The unique feature of these additives is their ability to lower the melting temperature of primer-template duplexes. Consequently, the presence of such compounds can shift the optimal annealing temperature downward, allowing for more flexibility in PCR setups. However, care should be taken, as they can also lead to fluctuating performance outcomes, depending on the specific combination and concentrations used.
Understanding these influencing factors helps in navigating the complexities that come with setting up effective PCR protocols. Introducing the right primers, optimizing their lengths, adjusting GC content, and understanding the role of additives can enhance the overall success rate of PCR—right from the early planning stages to executing the final experiment.
Calculating Optimal Annealing Temperature
Calculating the optimal annealing temperature is a cornerstone of successful PCR. This parameter determines how well the primers bind to the target DNA, significantly affecting the specificity and yield of the amplification. Getting the annealing temperature right isn’t merely a suggestion; it’s essential. If this value is too high, the primers might not bind efficiently, leading to poor amplification or completely no results. Conversely, if it’s too low, nonspecific binding occurs, resulting in unwanted products.
Not only does this affect experiment outcomes, but understanding the process of calculating the optimal annealing temperature also empowers researchers to design better primer sets. Knowing how to navigate through the methods available ensures that professionals can adapt to any changes in experimental conditions and objectives.
Basic Calculation Methods
There are several straightforward methods for calculating the optimal annealing temperature. Two of the pioneering formulas widely used are the Wilson’s formula and the Marmur–Melamede formula.
- Basic formula: A commonly accepted starting point is to calculate the annealing temperature based on the melting temperature (Tm) of the primers. A simplistic approach is:Here, T_a represents the annealing temperature and T_m is derived from your primer sequences.
- Calculation By Length: Another basic rule of thumb is that for every additional GC base pair, 1°C can be added to the Tm. The general formula iswhere A, T, G, and C refer to the respective numbers of the nucleotides.
T_a = T_m - 5°C
T_m = 2(A + T) + 4(G + C)
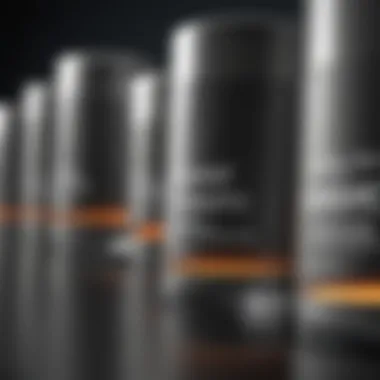
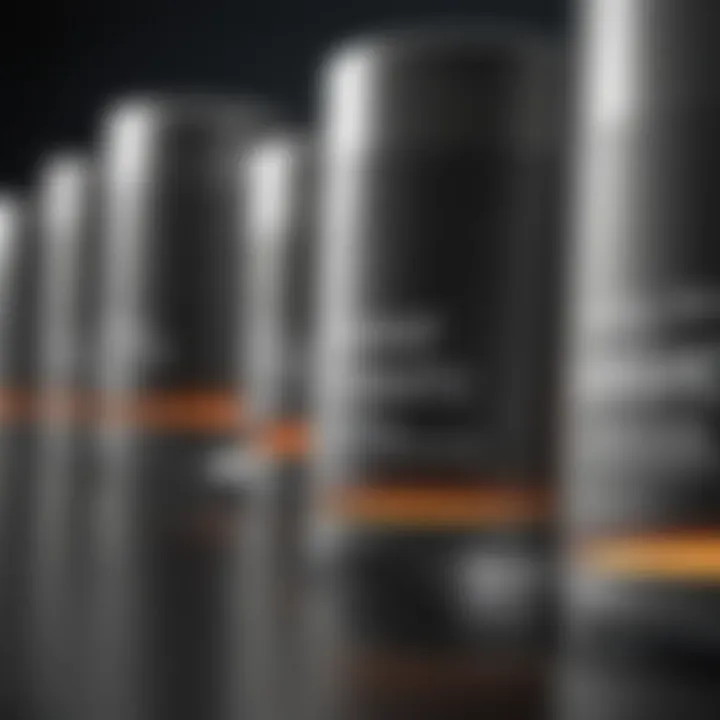
Employing these elementary methods can set a baseline. However, one must account for variations in primer design and reaction environments.
Advanced Approaches and Software Tools
For those looking to go beyond the fundamental calculations, advanced methods exist, often aided by various software tools. These tools incorporate more parameters, leading to a higher precision level in determining optimal annealing temperatures.
- Utilizing Software: Programs like OligoCalc and Primer3 allow users to input sequences and automatically generate Tm and T_a values. The consideration of the buffer components, ionic strength, and even specific salt concentrations can be factored in when using these tools.
- Simulative Approaches: Molecular modeling software that simulates primer-dna interactions can provide insights into how different temperatures may affect binding. This method can be particularly useful when dealing with complex templates or unusual primer designs. Additionally, some platforms calculate potential secondary structures that could impact the reaction.
By leveraging these advanced tools, practitioners can enhance their PCR protocols, paving the way for more robust data and reproducible results.
It's crucial to remember, regardless of the method used, that trial and error often play a part in setting the perfect conditions. Adjusting temperatures based on real experiment outcomes leads to a deeper understanding of one’s specific setups. Thus, while understanding the calculations is vital, practical experience provides the wisdom that guides future experiments.
Practical Considerations for PCR Protocols
When navigating the complex landscape of PCR, practical considerations play a crucial role in ensuring successful amplification of DNA. These considerations extend beyond merely setting the parameters; they encompass a holistic approach that integrates each component of the PCR process. Effective management of these variables can markedly enhance the reliability and efficiency of PCR experiments.
Adjusting Temperature for Different Primer Pairs
Every primer pair often has its own quirks—akin to a mismatched couple on a blind date. The optimal annealing temperature can vary significantly based on the specific characteristics of the primers being used. When dealing with multiple primer pairs, it might be beneficial to run a gradient PCR, where various temperature settings are tested in parallel. This approach helps identify the best working temperatures, making it possible to finetune the conditions specific to each set of primers.
The differences in the sequences, including variations in GC content and length, can affect how well the primers bind to the target DNA. This stipulates that understanding the nuances of how these factors play into temperature adjustments is essential.
Influence of Buffer Systems and Ions
Buffer systems in PCR aren’t mere background players; they significantly influence the efficiency and specificity of amplification. The components of these buffers, such as Tris-HCl and MgCl₂, create an environment where enzyme activity is optimized.
Magnesium ions, in particular, serve as a pivotal element, acting not just as a cofactor for the DNA polymerase but also interacting with both the DNA template and primers. Too much magnesium can lead to nonspecific binding, while too little can result in insufficient enzyme activity. Hence, it’s worth experimenting with different buffer formulations or adjusting magnesium concentrations to find the sweet spot for your particular experiment.
Effects of DMSO and Glycerol in PCR
Dimethyl sulfoxide (DMSO) and glycerol are additives that can be particularly beneficial in challenging PCR scenarios. DMSO is known for its ability to disrupt secondary structures in DNA, importantly aiding in the amplification of GC-rich templates, which can be notoriously tricky.
Conversely, glycerol may help stabilize enzymes and reduce the impact of inhibitors present in complex templates. However, the concentration of these additives must be carefully balanced. For instance, while a small fraction of DMSO can enhance yield, excessive amounts can adversely impact specificity. Achieving the right balance could mean the difference between a successful PCR run and one that yields disappointing results.
"Attention to detail in primer design and reaction conditions is not just common sense—it's essential to unlock the potential of your PCR experiments."
Through understanding and considering these practical aspects of PCR protocols, researchers can enhance their approaches, all but ensuring higher success rates in DNA amplification. The goal is not merely to follow standard protocols but to adapt and refine them to the specific needs of your experiments.
Common Issues and Troubleshooting
When it comes to polymerase chain reaction (PCR), achieving successful amplification of DNA can be like walking a tightrope. Numerous factors can lead to pitfalls in your experiment, making the understanding of common issues and their troubleshooting essential. Addressing these mishaps not only saves time and reagents but also boosts the reliability of your results. In essence, knowledge of troubleshooting can merge technical skill with scientific inquiry, transforming potential setbacks into opportunities for learning.
Non-Specific Amplification
Non-specific amplification occurs when the polymerase binds to unintended regions of the DNA, generating additional products. This can lead to ambiguous results, making it difficult to interpret the data accurately. A good understanding of the causes of non-specific amplifications aids in refining the PCR process.
Causes
One of the most common culprits for non-specific amplification is the misalignment of primers. Primers that are too complementary can hybridize to various regions of the template DNA, producing unexpected amplification products. The key characteristic of this issue is that it often becomes a nuisance when conducting high-precision experiments.
Another aspect to consider is the annealing temperature being too low. With a lower temperature, primers bind easily to non-target sites, resulting in non-specific amplification. This is particularly relevant in experiments requiring specificity. The unique feature of this cause is its variability; the extent can differ based on the template complexity and primer design itself,
Solutions
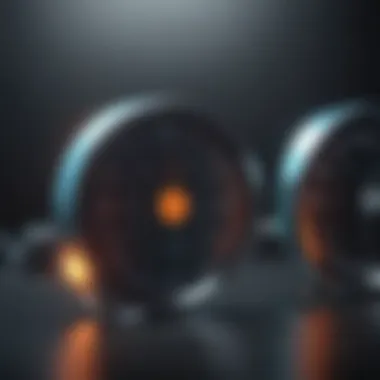
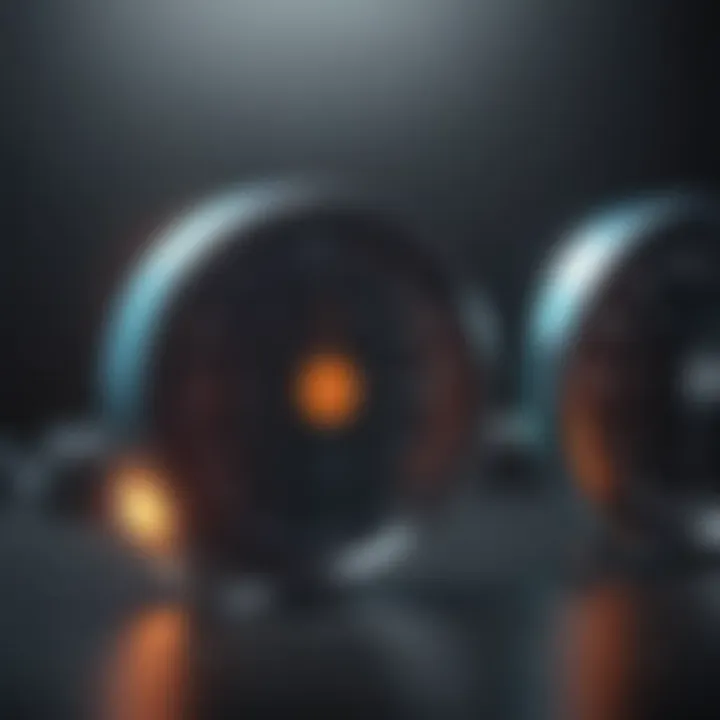
To mitigate non-specific amplification, opt for a primer redesign. Introducing mismatches or utilizing more specific sequences helps in enhancing the selectivity of the primers. This approach often proves effective, as the redesigned primers can eliminate unwanted interactions.
Additionally, increasing the annealing temperature can also alleviate non-specific bindings. While this might seem simple, it is a robust choice for addressing the issue. However, finding the right balance is essential, as too high a temperature might hinder specific binding as well. Here, the solution's unique feature is its dual-functionality—it both improves specificity while maintaining the potential for sufficient amplification.
Poor Yield or No Amplification
A poor yield or complete failure in amplification can be disheartening. Achieving optimal results requires careful consideration of a variety of factors that can influence the efficiency of PCR reactions.
Causes
One possible reason for low yield is subpar quality of reagents, such as enzymes or primers. If these components are compromised, they can severely limit the reaction's effectiveness. The key characteristic of this issue lies in the subtle attributes of reagents that are sometimes overlooked—temperature sensitivity and storage conditions often dictate their performance.
Another significant factor influencing yield is the template concentration. If the starting template concentration is too low, the polymerase might not have enough material to work with. This emphasizes the importance of initial sample quality and quantity, as they directly affect the success rate in amplification.
Solutions
To resolve yield issues, first ensure that all reagents are fresh and of good quality. This may necessitate replacing enzymes or re-evaluating storage conditions. Quality control is critical, as poor-quality ingredients contribute to unpredictable results.
Increasing the template concentration is another effective solution. This often leads to a proportional rise in yield, creating a more robust reaction. The approach's unique feature is that while it is simple to implement, it can have wide-ranging impacts depending on the experiment's requirements.
Optimization Strategies for Complex Templates
When working with complex templates, optimization becomes even more paramount. Variability in DNA sequences can produce a range of amplification outcomes. Adopting optimization strategies tailored to specific challenges can significantly boost the chances of success. Firstly, adjusting the magnesium ion concentration can alter enzyme activity, while modifying the cycle numbers could increase yields. Secondly, implementing a gradient PCR can help identify the best temperature for annealing, minimizing trial and error.
To wrap this section up, success in PCR often hinges on understanding potential issues and effectively troubleshooting them. Addressing these components ensures that you not only enhance the reliability of your results but also deepen your overall comprehension of the PCR process.
Future Directions in PCR Research
The field of Polymerase Chain Reaction (PCR) continues to evolve, driven by technological advancements and an ever-expanding understanding of molecular biology. Future directions in PCR research are pivotal for refining techniques and enhancing applications. Embracing innovations, especially in primer design and the integration of PCR with next-generation sequencing, can open new doors in various scientific fields. This future landscape provides insights that could reshape diagnostic methods and improve genetic research.
Innovations in Primer Design and Modification
Innovative approaches in primer design are becoming increasingly significant. Researchers are exploring various modifications to primers that can improve specificity and efficiency in amplification processes. For example, modifications such as locked nucleic acids (LNAs) or peptide nucleic acids (PNAs) can lead to higher binding affinity and lower dissociation rates. This can be a game-changer in scenarios where traditional primers struggle to generate clear and specific results.
Moreover, the use of bioinformatics tools aids in designing primers that target unique genetic regions, minimizing non-specific binding. The application of machine learning algorithms to predict primer behavior based on sequence data is another exciting trend. With these methods, primers can be customized for specific applications, whether in clinical diagnostics or research.
"Designing primers with these cutting-edge techniques can significantly streamline PCR workflows and enhance assay specificity, ensuring that researchers achieve the best possible results."
Applications of PCR in Next-Generation Sequencing
The link between PCR and next-generation sequencing (NGS) is more vital than ever. PCR serves as a foundational tool in preparing samples for NGS, allowing the amplification of regions of interest in the genome before sequencing. In light of this relationship, research is heading towards optimizing PCR protocols specifically for NGS applications.
The ingenuity in using PCR alongside NGS can facilitate the analysis of complex samples, such as environmental or clinical specimens. For instance, when dealing with metagenomics, the ability to amplify DNA from mixed microbial communities using tailored primers helps researchers decipher the diversity and functionality of organisms in their natural habitats.
Furthermore, new strategies such as PCR-based methods for targeted enrichment are gaining traction. These methods enhance the efficiency of sequencing by allowing specific regions of interest to be amplified and sequenced, thereby improving the overall accuracy of the results.
The synergy between PCR and NGS is crucial, promising to not only speed up research but also improve our understanding of genetic underpinnings in various diseases and ecological systems. With effective combinations of technologies, the future of PCR research looks promising, offering sophisticated solutions to long-standing challenges in molecular biology.
Closure
The conclusion of this article serves as a critical juncture, tying together the various threads associated with PCR primer annealing temperature. Understanding this concept is paramount for anyone engrossed in the field of molecular biology, whether they are seasoned researchers or budding students. The nuances of annealing temperature can significantly influence the specificity and efficiency of DNA amplification, directly impacting research outcomes.
Summary of Key Points
In summarizing the key points, we have walked through:
- Definition of Annealing Temperature: We defined this crucial parameter, illustrating its role in the hybridization process between primers and target DNA.
- Factors Influencing Annealing Temperature: Key factors include the length of primers, GC content, and the presence of additives, all of which shape how primers bind during PCR.
- Calculating Optimal Annealing Temperature: We reviewed basic methods for calculating the perfect annealing temperature, along with advanced software tools that can simplify this process.
- Common Issues: Challenges like non-specific amplification and poor yield were discussed, alongside the troubleshooting strategies necessary to overcome them.
- Future Directions: Innovations in primer design were highlighted, showcasing ongoing research aimed at improving PCR techniques.
Implications for Future Work in Molecular Biology
- Enhanced Techniques: The refinement of PCR protocols can lead to increased precision in genetic research, facilitating breakthroughs in areas such as gene editing and forensic science.
- Next-Generation Applications: As PCR is combined with next-generation sequencing, understanding annealing temperature becomes even more vital, promoting higher fidelity and more reliable results.
- Broadening Accessibility: For educators, a solid grasp of these principles can enhance teaching methods, ensuring that new generations of scientists appreciate the intricate dynamics of PCR.