Mastering Mutagenesis Primer Design Techniques
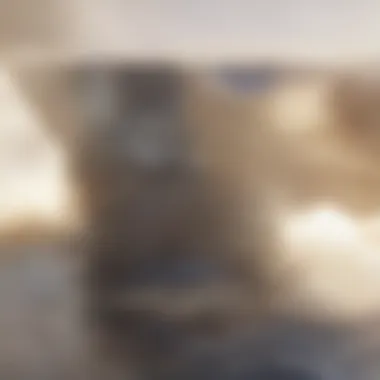
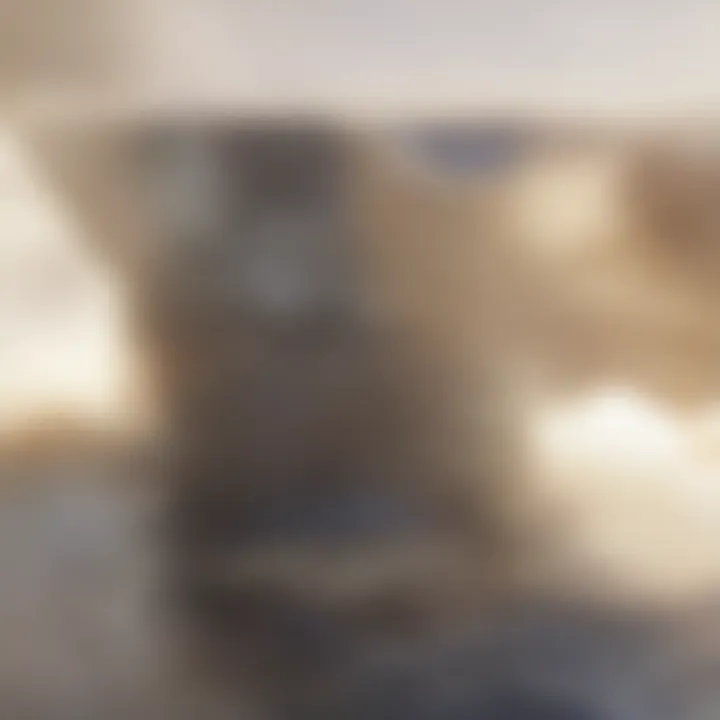
Intro
Mutagenesis primer design is fundamental in molecular biology, especially for those aiming to alter genetic sequences with precision. Researchers utilize primers to create targeted mutations, whether for studying gene function or developing new traits in organisms. Understanding the design of these primers not only improves the success rate of mutagenesis experiments but also enhances the overall reliability of genetic manipulations.
In this guide, we dissect the multifaceted nature of primer design, elaborating on the various mutagenesis techniques available. We explore the characteristics that define effective primers and introduce the computational tools that streamline the design process. This comprehensive overview aims to arm students, educators, and professionals with the knowledge needed to navigate potential obstacles in primer design, thereby ensuring accuracy in gene editing tasks.
Key aspects such as common strategies and inherent pitfalls are addressed to promote a deeper understanding of the subject. The narrative unfolds to highlight the importance of optimizing mutagenesis experiments, making this guide a valuable resource within the scientific community.
Key Findings
Summary of the main results
Through extensive research, it appears that successful mutagenesis primarily depends on several pivotal factors:
- Primer length: Typically, primers should be 18 to 30 nucleotides long to ensure specificity.
- GC content: A balanced GC ratio aids in the stability of the primer-template interaction, with a recommended range of 40% to 60%.
- Melting temperature (Tm): A nearer Tm between primers ensures uniform extension by DNA polymerases.
Using appropriate software tools for designing these primers has shown to increase efficiency in the experimental setup. The analysis also indicates that improper primer design leads frequently to low mutation rates or non-specific amplifications.
Significance of findings within the scientific community
These insights are particularly significant. They not only guide researchers in optimizing their protocols but also contribute to advancements in genetic engineering and synthetic biology. When user create primers that adhere to the established guidelines, they minimize errors and maximize the potential for successful outcomes in experiments. This is crucial in fields like gene therapy, where accuracy is paramount.
Implications of the Research
Applications of findings in real-world scenarios
The insights gained from mutagenesis primer design are applicable across many domains:
- Biomedical research: Improved understanding of gene function can lead to new treatments.
- Agricultural biotechnology: Creating transgenic plants with favorable traits can enhance crop yields.
- Synthetic biology: Designing organisms with novel functionalities for industrial applications.
Potential impact on future research directions
Furthermore, as methodologies progress, the integration of machine learning in primer design shows promise. Enhanced algorithms could analyze large databases and predict optimal primer configurations. This could reshape the landscape of genetic research, making it more efficient and less error-prone.
Prelims to Mutagenesis
The field of mutagenesis plays a vital role in molecular biology, primarily due to its ability to facilitate targeted modifications in genetic sequences. Understanding mutagenesis is essential for researchers and practitioners in various disciplines, including genetics, bioengineering, and pharmaceutical development. By altering specific genes, scientists can elucidate their functions, investigate disease mechanisms, and develop novel therapeutic strategies.
Definition and Importance
Mutagenesis is defined as the process by which an organism's genetic information is changed, leading to mutation. This can occur naturally or can be induced deliberately through various techniques. The importance of mutagenesis is underscored by its applications in gene therapy, where it can correct faulty genes responsible for diseases. It is also fundamental in producing genetically modified organisms (GMOs), which have applications in agriculture and environmental management.
Researchers utilize mutagenesis in protein engineering to enhance or modify the properties of proteins, improving their performance in industrial and medical applications. Thus, a comprehensive understanding of mutagenesis impacts a wide range of scientific domains and offers numerous benefits, including:
- The ability to understand gene function and interaction.
- Development of new biotechnological products.
- Insights into evolutionary processes.
Historical Context
The exploration of mutagenesis dates back over a century. The discovery of the role of DNA as the genetic material in the early 20th century laid the foundation for further research. In the 1940s, the concept of induced mutations using chemical agents became prominent. Scientists like George W. Beadle and Edward Tatum demonstrated that specific genes could be targeted, establishing a relationship between genes and phenotypic traits.
With the advancement of molecular biology techniques in the 1970s and 1980s, methods such as site-directed mutagenesis emerged. These sophisticated approaches allow precise alterations to specific locations in the DNA sequence. Over the years, numerous tools and techniques have been developed to improve the efficiency and accuracy of mutagenesis, making it an indispensable component of modern biological research. The continuous evolution of technologies, including CRISPR and NGS, has further propelled the field, enabling researchers to manipulate genomes with remarkable precision.
Mutagenesis has revolutionized our understanding of genetics and remains central to cutting-edge studies in gene editing and therapy.
Understanding the history and importance of mutagenesis provides essential context as we delve deeper into the core principles of primer design and application in experimental settings.
Fundamental Principles of Primer Design
Understanding the fundamental principles of primer design is crucial for ensuring the success of mutagenesis experiments. Proper primer design serves as the foundation for specific and efficient amplification or modification of target DNA sequences. It is essential to consider various elements that influence the overall effectiveness of primers, as well as their impact on the quality of the resulting mutagenesis.
These principles focus on several essential characteristics of primers. Each characteristic significantly contributes to the durability and specificity of the binding process, allowing for effective manipulation of genetic material. Therefore, recognizing these features can greatly facilitate designing effective primers that yield high-quality results.
Understanding Primer Functionality
Primers are short sequences of nucleotides that initiate the synthesis of DNA during the polymerase chain reaction (PCR). The functionality of a primer hinges on its ability to hybridize specifically to the target sequence under controlled conditions. Once bound, DNA polymerase extends the primer complementary to the template strand, leading to the amplification of the desired DNA segment. Understanding the specific binding capabilities and hybridization properties of primers is crucial, as improper design can lead to non-specific binding or ineffective amplification, resulting in poor experimental outcomes.
Key Characteristics of Effective Primers
Several characteristics define effective primers, impacting their performance in mutagenesis and PCR applications. Three key factors include length, GC content, and melting temperature.
Length
The length of a primer typically ranges from 18 to 30 nucleotides. This specific aspect is vital because longer primers may provide increased specificity in binding due to more potential interactions with the target sequence. Longer primers can be a beneficial choice, as they reduce the likelihood of non-specific binding, which can compromise the validity of results. However, if a primer is too long, it might be more prone to form secondary structures, potentially hindering the amplification process. Therefore, maintaining an optimal length is essential for enhanced performance in mutagenesis.
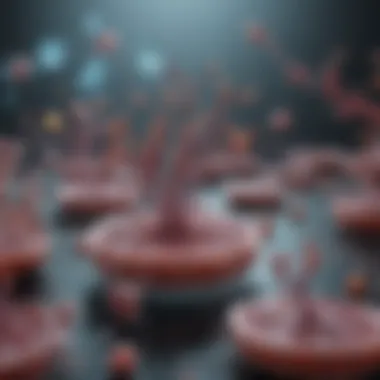
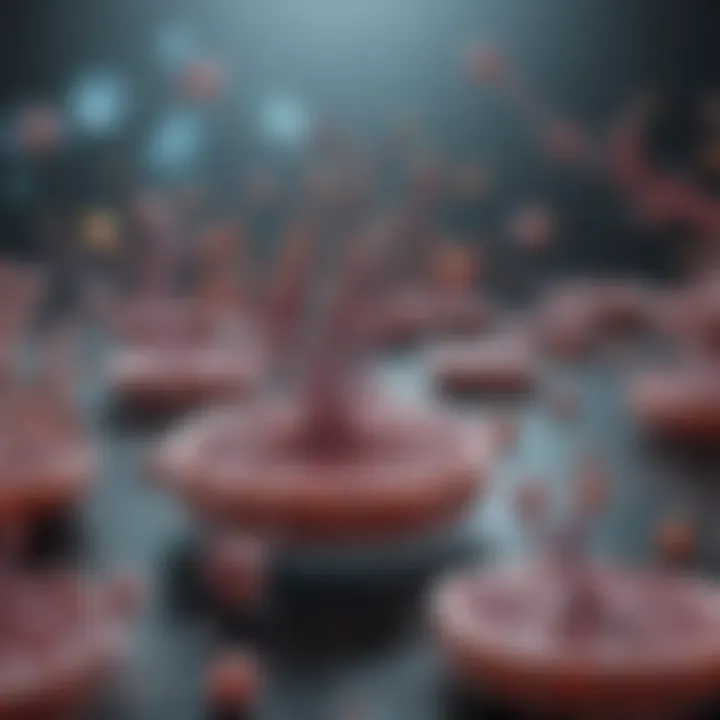
GC Content
GC content refers to the proportion of guanine and cytosine bases within the primer sequence. This characterisatic plays a critical role in primer stability, with higher GC content leading to stronger hydrogen bonding at the binding site. A balanced GC content, around 40-60%, often offers optimal binding stability, which is advantageous for ensuring specificity during amplification. However, primers with excessively high GC content may increase the risk of forming secondary structures, which can reduce efficiency in the amplification process.
Melting Temperature
Melting temperature (Tm) is a measure of the temperature at which half of the DNA strands are in the double-helix state and half are in a single-stranded state. Tm is influenced by both primer length and GC content. It is crucial to design primers with compatible Tm values to ensure simultaneous binding during PCR. The typical range for Tm in effective primers is about 50-65°C. Primers with a Tm outside this range may lead to inconsistent binding and amplification efficiency. Moreover, having Tm values within 2-5°C of each other for paired primers maximizes the chances of successful amplification.
Summary:
Effective primer design is characterized by specific features such as length, GC content, and melting temperature. Each factor impacts the overall success of mutagenesis and PCR applications. Properly addressing these principles enhances the reliability of experimental outcomes.
Types of Mutagenesis Techniques
Understanding the various mutagenesis techniques is vital for researchers aiming to modify genes. Each technique serves distinct purposes, ranging from specific point mutations to broader genomic alterations. This knowledge helps in selecting the suitable method based on the goals of the experiment. Below are the primary types of mutagenesis techniques.
Site-Directed Mutagenesis
Site-directed mutagenesis is a powerful method that allows precise alterations at specific locations within a gene. This technique is essential in protein engineering, where researchers might need to investigate the role of single residues or modify the properties of proteins.
The process typically involves designing a primer that contains the desired mutation along with the complementary sequence for the target gene. After PCR amplification, the mutated fragment can be incorporated into a plasmid. The accuracy of this technique minimizes undesired changes while enabling specific experimental outcomes.
The advantages of site-directed mutagenesis include:
- Precision: Specific changes can be made with high fidelity.
- Versatility: Applicable across various organisms and systems.
- Insight into Function: Enables exploration of protein function by changing specific amino acids.
However, one must consider the potential challenges, such as the design of effective primers and maintaining template fidelity during amplification.
Random Mutagenesis
Random mutagenesis, as the name suggests, introduces alterations throughout a gene or a genomic region without a predetermined target. This approach can be particularly useful when the effects of multiple mutations on a phenotype need to be studied, such as in directed evolution experiments.
The general methodology involves using a mutagen, such as chemical agents or error-prone DNA polymerases, to create a library of variants. Researchers can subsequently screen these variants to identify those possessing desirable traits, such as enhanced stability or activity.
Key points to note about random mutagenesis are:
- High Variation: Generates many mutants, increasing the chance of finding effective variants.
- Broad Applications: Useful in enzyme engineering and functional studies.
- Screening Necessary: Due to randomness, successful identification of desired mutants requires efficient screening methods.
Error-Prone PCR
Error-prone PCR is a specialized form of random mutagenesis that focuses on generating mutations during the amplification process. By manipulating the PCR conditions, such as using specific DNA polymerases with lower fidelity, researchers can deliberately introduce errors into the amplified DNA.
This technique allows for the creation of genetic diversity, facilitating the evolution of new functions in proteins. Researchers often use error-prone PCR in combination with selection methods to enrich for variants that display improved properties or new activities.
Considerations for error-prone PCR include:
- Controlled Mutation Rate: Mutations can be adjusted by varying enzyme concentrations or cycling conditions.
- Diversity: Produces a wide range of genetic variants.
- Selection Needed: Post-amplification selection is necessary to isolate beneficial mutants.
In summary, choosing the right mutagenesis technique is crucial for achieving specific experimental goals, whether that be through precision, randomness, or a combination of both. Each technique has its benefits and challenges, influencing the outcomes of genetic manipulation efforts.
These insights into mutagenesis techniques provide a solid foundation to develop robust and effective primer designs suited for any genetic experimentation.
Designing Primers for Mutagenesis
Designing primers for mutagenesis is a fundamental aspect of molecular biology that can significantly impact the success of experiments involving genetic modifications. Properly designed primers ensure the specificity and efficiency of amplifying target sequences while introducing desired mutations. This section outlines the critical elements of primer design, the benefits it brings, and important considerations that researchers must keep in mind.
Strategically, the process involves meticulous planning and allows researchers to precisely target specific genes or regions in the genomic DNA. Effective primer design can prevent common pitfalls, such as non-specific bindings that lead to unwanted amplification products. Understanding the nuances of primer characteristics is essential, especially when aiming for high specificity and efficiency in mutagenesis experiments.
Additionally, a focused primer design can enhance downstream applications, like cloning or sequencing, resulting in more reliable and reproducible outcomes. The design process encompasses various steps, requiring both theoretical knowledge and practical experimentation.
Moreover, utilizing specialized software tools simplifies the process and streamlines primer design. These tools are vital in achieving the balance between primer specificity and efficiency, accommodating complex requirements of mutagenesis protocols.
Step-by-Step Primer Design Process
The primer design process consists of several precise steps that ensure the successful creation of effective primers for mutagenesis. These steps include:
- Identifying the target sequence: Knowing the gene or region of interest is crucial. This often involves examining existing genomic data to determine the location and type of mutations needed.
- Determining the primer length: Generally, primers should be between 18 and 25 base pairs to maintain specificity during amplification.
- Calculating GC content: An ideal GC content of around 40-60% enhances the stability of the duplex during PCR.
- Setting the melting temperature: Aim for a melting temperature of 50-65°C, ensuring that both primers used in the reaction have similar melting temperatures to optimize performance.
- Checking for secondary structures: Use software tools to identify potential hairpins or dimers which could interfere with the PCR.
- Testing for specificity: A blast search can confirm that the primers solely bind to the intended target sequence.
By following these systematic steps, researchers can enhance the likelihood of successful amplification and subsequent mutagenesis.
Software Tools for Primer Design
Utilizing software tools can greatly facilitate the primer design process, providing researchers with powerful resources to validate their designs effectively. Here are three widely used tools:
BioEdit
BioEdit is a versatile biological sequence alignment editor that offers various tools for analyzing DNA and protein sequences. One key characteristic of BioEdit is its user-friendly interface, which allows researchers to visually explore primer sequences in conjunction with their target genes. This functionality makes it a beneficial choice for primer design, especially for newcomers in the field.
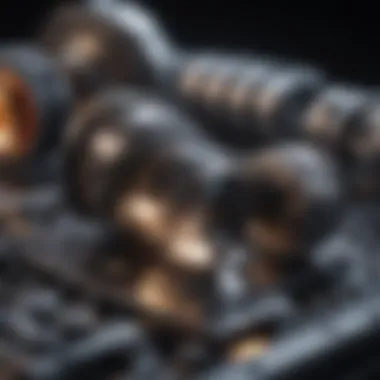
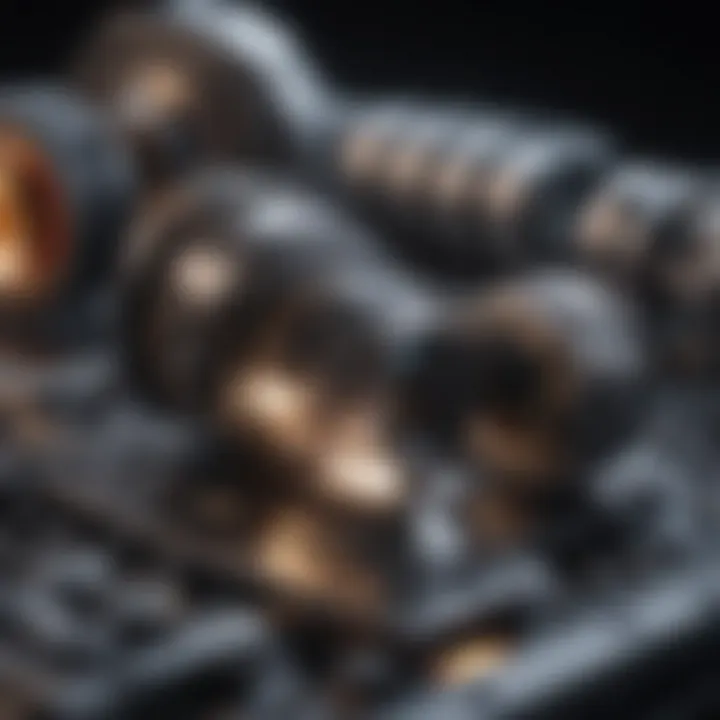
BioEdit simplifies primer optimization by allowing users to analyze sequences for potential secondary structures and calculating melting temperatures. However, while BioEdit is suitable for basic primer design tasks, it may lack some advanced features found in dedicated primer design software, which could limit its use in more complex scenarios.
Primer3
Primer3 is a widely recognized software that focuses specifically on primer design. Its key characteristic is the robust algorithm that helps generate optimal primers based on user-defined criteria. This makes Primer3 a popular choice among researchers for various projects, including mutagenesis.
One unique feature of Primer3 is its ability to allow users to set specific parameters such as product size and GC content limits, offering great flexibility in primer design. While it provides comprehensive outputs, some users may find its interface slightly less intuitive compared to BioEdit.
Geneious
Geneious is a comprehensive bioinformatics platform known for its extensive features that encompass not just primer design but also whole analysis workflows. Its key characteristic lies in its integration of multiple functions, allowing for cloning, alignment, and visualization of sequences all in one platform, streamlining the entire research process.
For primer design, Geneious incorporates advanced algorithms that calculate melting temperatures and potential secondary structures efficiently. Its intuitive interface makes it easy for users to navigate and apply various features. However, the subscription cost may be a barrier for some users, particularly those in academic settings with limited budgets.
In summary, selecting the right software tool for primer design is essential. Each tool has unique advantages and limitations, and the choice largely depends on the complexity of the project and the user’s familiarity with bioinformatics software.
Optimizing Primer Design
Optimizing primer design is a foundational step in mutagenesis experiments. It is crucial because the effectiveness of mutagenesis largely depends on the quality of the primers used. Properly designed primers increase the likelihood of successful amplification and mutation while reducing experimental errors. This enhancement is essential in achieving precise modifications in genetic sequences, which can ultimately lead to significant breakthroughs in research and biotechnology.
There are several specific elements that researchers must consider when optimizing primers. These include avoiding secondary structures and increasing the specificity of the primer sequences. Addressing these elements helps ensure successful mutagenesis outcomes.
Avoiding Secondary Structures
Secondary structures in primers can pose significant challenges during the PCR process. These structures occur when complementary regions within the primer bind to each other, leading to the formation of hairpins and loops. Such structures can affect the availability of the primer for hybridization with the target DNA, thus reducing amplification efficiency.
One approach to avoid secondary structures is to use primer design software, which can predict potential structures before the primers are synthesized. Additionally, researchers can manually check primer sequences for complementary regions and adjust them accordingly. For example, ensuring that the ends of primers are not complementary can greatly reduce the formation of unwanted structures. Here are a few more tips to avoid secondary structures:
- Keep primer lengths between 18-25 nucleotides.
- Aim for a balanced GC content around 40-60%.
- Utilize a tail sequence that lacks complementarity.
By implementing these strategies, researchers can enhance the likelihood of successful amplification, which is critical for effective mutagenesis.
Increasing Specificity in Design
Increasing specificity in primer design is paramount to achieving targeted mutagenesis. Specific primers bind only to their intended target sequence, minimizing the chances of non-specific amplification. Non-specific binding can lead to undesired mutations or artifacts, complicating the interpretation of results.
To enhance specificity, there are several considerations to keep in mind. First, researchers should ensure that the primer's melting temperature (Tm) is appropriate. This can help maintain a stable annealing temperature during PCR, which in return supports specific binding. Additionally, designing primers with unique sequences that are not present in other areas of the template might further minimize non-specific interactions.
Here are some additional strategies to increase specificity:
- Design primers that are at least 18 base pairs in length.
- Use a 3' end that is not complementary to any other sequences in the template DNA.
- Conduct a BLAST search to verify the specificity of the primer sequence against the target genome.
By adhering to these principles in primer design, the overall success and accuracy of mutagenesis experiments can be significantly improved. This optimization not only enhances the quality of experimental results but also contributes to the body of knowledge in genetic research.
Common Challenges in Mutagenesis Primer Design
The design of mutagenesis primers can often present researchers with numerous challenges. Understanding these challenges is essential for achieving successful experimental outcomes. By identifying and addressing potential problems in the primer design process, one can enhance the reliability and specificity of the mutagenesis. This section will discuss specific elements that can lead to difficulties, emphasizing their implications on the experimental results.
Sequence Homology Issues
A significant challenge in mutagenesis primer design is related to sequence homology. When designing primers, it is important to ensure that they bind specifically to the target sequence without unintended interactions with similar sequences in the genome. If a primer exhibits homology to other regions, it can amplify non-target DNA, leading to ambiguous results and poor interpretability of data.
To mitigate these issues, researchers need to:
- Perform thorough sequence alignments: Utilize tools like BLAST to identify potential homologous sequences in the target organism's genome.
- Design specific primers: Aim for unique sequences that distinctly match the regions of interest. This will ensure that the primer binds correctly and reduces the likelihood of undesired amplification.
It's also critical to consider the context in which the primer will be used. Often, modifications to the primer can help improve its specificity and reduce the chances of sequencing errors or disruptions in gene function.
Amplification Bias
Amplification bias is another challenge in primer design, which can occur when certain templates preferentially amplify over others during PCR. This phenomenon is influenced by the primer design, the quality of the template, and the PCR conditions used. Amplification bias can skew results and result in misleading data interpretations.
There are several strategies to address amplification bias:
- Optimize PCR conditions: Adjusting factors such as annealing temperature, extension time, and cycle number can greatly influence the outcome. Crtically evaluate the conditions for each specific experiment to improve efficiency.
- Utilize balanced primer concentrations: Ensure that the forward and reverse primers are present at optimal concentrations to minimize bias towards one template over another.
- Use high-fidelity polymerases: When amplification bias is a concern, employing high-fidelity enzymes for PCR can help minimize errors, ensuring accurate representation of the target sequence in the amplification process.
The careful consideration of amplification bias and sequence homology issues during primer design can significantly enhance the accuracy and reliability of mutagenesis experiments.
Ultimately, overcoming these challenges is key to successful mutagenesis, ensuring that the experimental results are both valid and informative.
Validation of Mutagenesis Primers
Validating mutagenesis primers is an essential process in the field of molecular biology. The accuracy of a mutagenesis experiment heavily relies on primer validation, which ensures that the designed primers perform as intended in gene modification. By verifying the functionality of primers, researchers can reduce the likelihood of errors that may arise during experiments. This section highlights critical aspects of primer validation, emphasizing its significance in achieving successful outcomes in mutagenesis applications.
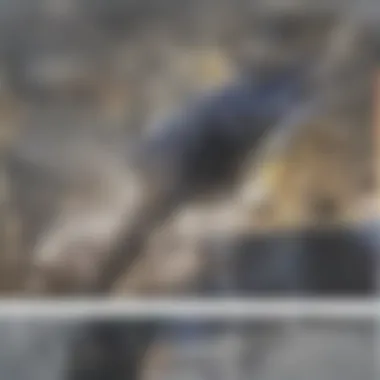
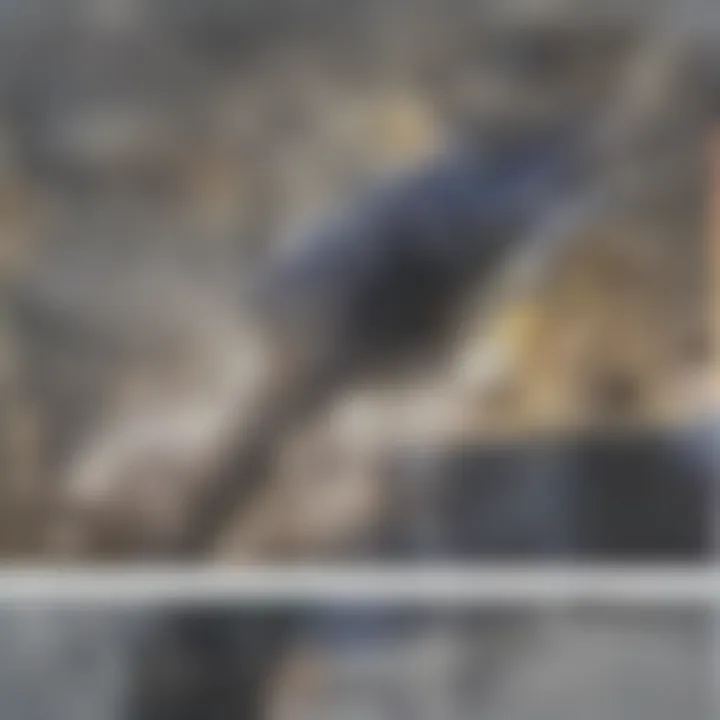
Methods of Primer Testing
The testing of primers is crucial to determine their performance in PCR reactions. Several methods can be utilized for primer testing, each providing specific insights into their effectiveness. Common approaches include:
- In Silico Analysis: Computational tools evaluate potential primer sequences for secondary structures, self-dimerization, and compatibility with target DNA. Tools such as Primer3 and Geneious can assist in predicting the behavior of primers before experimental application.
- Gel Electrophoresis: This technique can visualize PCR products to confirm if the primers are functioning correctly. A successful amplification results in a distinct band appearing on the gel, indicating that primer and template interaction occurred as expected.
- Sequencing: Once PCR is completed, sequencing the amplified products can verify that the desired mutation was successfully incorporated. This method provides definitive proof of the primer's efficacy.
"Validating primers through various testing methods minimizes the risk of introducing unintentional mutations during gene editing, ultimately enhancing the reliability of biological experiments."
Incorporating Control Experiments
Control experiments play a fundamental role in validating mutagenesis primers. These controls serve as benchmarks that help to interpret the results of the main experiment. Common control strategies include:
- Negative Controls: These are reactions that do not include the template DNA. They allow researchers to confirm that any observed amplification is indeed due to the primers and not due to contamination or non-specific amplification.
- Positive Controls: Including a known template that is guaranteed to amplify allows researchers to establish that the PCR conditions are suitable for the intended reactions. If the positive control fails, it indicates potential problems in the reaction setup.
- Reproducibility Testing: Running the same PCR with multiple replicates can demonstrate the consistency of primer performance. Reproducibility is key in scientific experiments, ensuring that the findings are reliable and can be trusted.
These control experiments are vital to the validation of mutagenesis primers. They provide an additional layer of confirmation that the observed results stem from the primer’s activity and not extraneous factors. Through robust validation, researchers can have greater confidence in their experimental designs.
Applications of Mutagenesis
Mutagenesis serves as a vital tool in molecular biology, enabling researchers to explore and manipulate genetic material for various applications. Understanding how to apply mutagenesis techniques effectively is essential for advancing knowledge in genetics, biochemistry, and related fields. In this section, we will discuss two significant applications of mutagenesis: functional gene studies and protein engineering.
Functional Gene Studies
Functional gene studies involve examining the role of specific genes in biological processes. Mutagenesis allows scientists to create specific changes in the DNA sequence, helping to elucidate gene function. This process is crucial for understanding genetic pathways and their effects on phenotypes.
Using mutagenesis, researchers can introduce point mutations, deletions, or insertions in a target gene. By analyzing the resulting phenotypic changes, scientists gain insights into gene function. For example, site-directed mutagenesis is often used to study the impact of particular amino acids on protein function. These experiments can reveal information about gene regulation, interactions, and evolutionary relationships.
Moreover, functional gene studies can also assist in identifying genes linked to diseases. By manipulating genes believed to play a role in a condition, researchers can assess the effects of mutations. Such studies may highlight potential therapeutic targets for gene therapy or precision medicine.
Protein Engineering
Protein engineering is another prominent application of mutagenesis, allowing scientists to design and create proteins with enhanced properties. This field aims to optimize protein function for specific industrial, medical, or research purposes. By introducing mutations, researchers can alter characteristics such as enzyme activity, stability, and specificity.
For instance, in enzyme engineering, mutagenesis can be utilized to increase enzyme efficiency or to create enzymes that operate under extreme conditions. Additionally, targeted mutations can improve the binding affinity of antibodies or other proteins for their ligands. Such advancements have broad implications in areas like pharmaceuticals, diagnostics, and biotechnology.
While protein engineering through mutagenesis offers significant benefits, it also requires careful consideration of the mutations introduced. Random mutagenesis techniques, for example, can lead to unintended consequences if not properly managed. It becomes essential to validate the functional outcomes of engineered proteins thoroughly.
"By strategically applying mutagenesis, researchers can unlock new possibilities in the field of protein design and functional studies, ultimately leading to improved technologies and therapeutic approaches."
In summary, applications of mutagenesis are diverse and impactful in the realm of biological sciences. Through functional gene studies and protein engineering, it enables a deeper understanding of genetic functions and offers innovative solutions to existing challenges. The ongoing advancement of mutagenesis techniques will likely reveal new opportunities for future research and practical applications.
Recent Advancements in Primer Design
Recent advancements in primer design are transforming the landscape of molecular biology. As the field evolves, new methodologies and technologies are enhancing the efficiency and accuracy of mutagenesis primer design. The continual development of these techniques is essential because it allows researchers to work with greater precision, ultimately pushing the boundaries of genetic manipulation. This section will explore novel approaches and the integration of artificial intelligence in the primer design process, underlining their implications and practical benefits for researchers.
Novel Approaches and Techniques
One key advancement in primer design is the rise of innovative approaches that address past limitations. Researchers have introduced various novel techniques that improve specificity and reduce off-target effects.
- Diverse Primer Design Algorithms: Algorithms have been developed to optimize primer sequences effectively. These algorithms factor in numerous parameters such as secondary structures and potential dimer formation, which improves the reliability of amplification reactions.
- Next-Generation Sequencing (NGS): The integration of NGS into primer design allows for more comprehensive analysis of genomic regions. By utilizing large-scale sequencing data, researchers can identify optimal target areas for mutagenesis, making primer design more informed.
- Multiplex Primer Design: This technique allows for simultaneous amplification of multiple targets using a set of primers. It is particularly useful in complex genetic studies where multiple mutations are necessary, enabling streamlined experimentation.
These advancements collectively contribute to more robust and reproducible experimental results. They provide a clearer understanding of how varying designs can influence outcomes in mutagenesis studies.
Integrating AI in Primer Design
The integration of artificial intelligence (AI) into primer design signifies a notable advancement in genetic research. AI models are adept at analyzing large datasets to identify patterns that may not be readily apparent to human researchers.
- Predictive Analysis: AI can predict successful primer attributes based on existing data, facilitating more strategic choices in primer sequences. It analyzes previous experiments to learn which combinations yield favorable results, allowing researchers to bypass trial-and-error phases.
- Dynamic Modifications: AI-driven tools can adapt and evolve based on the results obtained from ongoing experiments. This adaptability ensures that the primer design process becomes increasingly efficient over time.
- Error Reduction: By using machine learning algorithms, AI can minimize errors in primer design and amplify specificity. Such precision is crucial, as errors in primer sequences lead to unwanted mutations or failed experiments.
In summary, the advancements in primer design through novel techniques and AI integration are significant for the future of mutagenesis. They provide researchers with powerful tools to enhance their experimental designs, ultimately achieving better accuracy and consistency in genetic manipulation. > The incorporation of advanced technologies such as AI not only expedites the primer design process but also enables more complex and nuanced studies in molecular biology.
These developments underscore the importance of staying abreast of innovations in primer design for students, researchers, educators, and professionals alike, ensuring that they can leverage these tools effectively in their work.
Finale
The conclusion of this article underscores the pivotal role of mutagenesis primer design in advancing molecular biology. Effective primer design is not merely a technical requirement; it serves as the foundation for successful gene manipulation. By ensuring accurate mutations, researchers can gain invaluable insights into gene functions, disease mechanisms, and the principles of protein interactions. This section encapsulates the essential elements that emphasize the importance of robust primer design principles.
A key takeaway is the intricate balance between specificity and efficiency in primer design. Specificity ensures that primers bind accurately to their intended sequences, minimizing off-target mutations. Efficiency relates to the ability of the primers to work effectively in various conditions, promoting successful amplification of the target DNA. Both factors significantly impact the reliability of mutagenesis outcomes.
Furthermore, as the field evolves, researchers must remain adaptable to new technologies and methods emerging in primer design. The incorporation of advanced computational tools and AI can lead to even more precise and efficient designs. This not only enhances the accuracy of experiments but also saves time and reduces resources.
In summary, the importance of detailed and methodical mutagenesis primer design cannot be overstated. It remains an essential skill for any researcher engaged in genetic modification.
Summary of Key Points
- Mutagenesis Primer Design is crucial for successful gene manipulation and accurate modifications.
- The effectiveness of a primer is determined by its specificity and efficiency.
- Employing advanced software can significantly enhance the design process.
- Adaptation to new techniques ensures researchers stay at the forefront of molecular biology.
Future Directions in Mutagenesis Primer Research
The future of mutagenesis primer research is promising with several avenues ripe for exploration. As genetic engineering becomes more complex, the demand for precise and reliable mutagenesis strategies will grow. Here are some potential directions:
- Integration of Artificial Intelligence: AI can optimize primer design by predicting the best candidates based on sequence data, helping automate and refine the selection process.
- Development of Novel Techniques: Techniques such as CRISPR and its variants could redefine how mutagenesis is approached, providing more straightforward and precise means to achieve desired genetic modifications.
- Focus on High-Throughput Methods: As high-throughput sequencing technologies advance, creating multiplexed mutagenesis systems will enable efficient evaluation of multiple mutations simultaneously.
- Enhanced Understanding of Primer-Template Interactions: More in-depth studies on how primers interact with templates could lead to improved characteristics focusing on minimizing amplification bias.