Household Biogas Plants: Design and Benefits
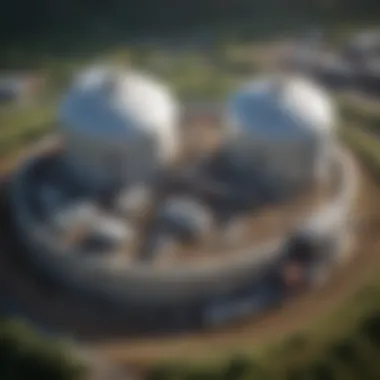
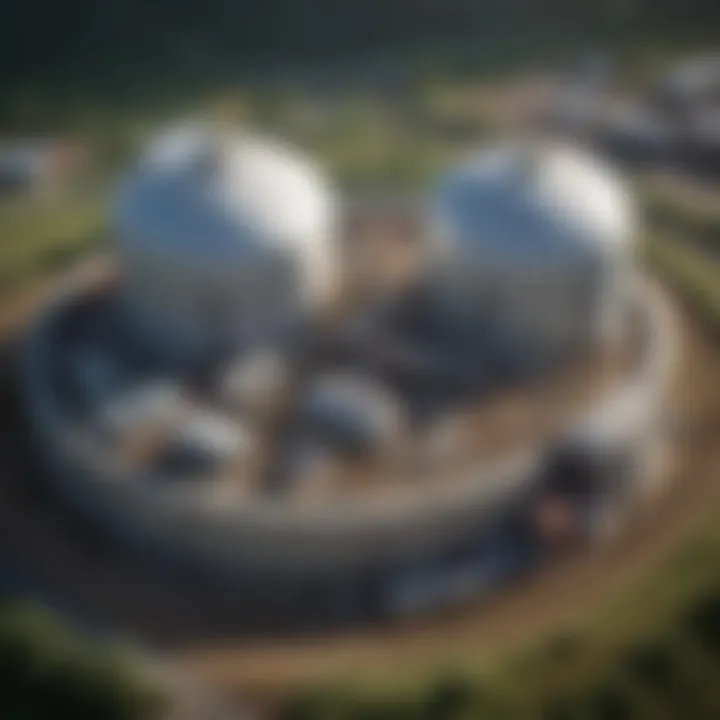
Intro
Household biogas plants represent a fascinating intersection of environmental sustainability and energy independence. As more individuals and families seek ways to reduce their carbon footprints, the appeal of generating fuel from organic waste has grown significantly. This has led to innovative approaches in renewable energy generation—particularly in developing regions where energy resources may be limited. Household biogas systems not only provide an efficient energy source but also tackle waste management issues, creating multiple layers of utility.
In the exploration of household biogas plants, one ventures into the principles of anaerobic digestion, where microorganisms break down organic material in the absence of oxygen. The end products of this process—primarily biogas and digestate—hold immense potential for energy generation and agricultural application.
This article aims to provide a comprehensive understanding of the various aspects associated with household biogas systems, from their design and implementation to their socio-economic effects. The highlighting of practical experiences, case studies, and a look at the technology behind these systems will create a richer understanding of their role in modern energy solutions.
Key Findings
Summary of the Main Results
One of the standout discoveries in the deployment of household biogas plants is their significant capability to convert waste into a clean energy source. Studies indicate that a typical biogas system can produce enough energy to meet the cooking needs of an average household, substantially reducing reliance on traditional fossil fuels. Moreover, the residue from the digestion process, known as digestate, serves as an excellent fertilizer, enhancing soil quality and promoting sustainable agricultural practices.
Significance of Findings Within the Scientific Community
The findings surrounding household biogas systems have implications that extend beyond localized energy generation. They contribute to broader discussions about the circular economy, waste management, and sustainable farming practices. By converting waste into energy, biogas technologies illustrate how resource recovery systems can aid in the development of sustainable food systems, promote climate resilience, and reduce greenhouse gas emissions.
"Biogas production is not just an energy solution; it is a transformative approach to waste that aligns with global sustainability goals."
Implications of the Research
Applications of Findings in Real-World Scenarios
The practicality of household biogas plants can be realized in various contexts around the globe. For instance, rural areas with abundant agricultural waste can effectively leverage biogas systems for energy and waste management. In places struggling with energy shortages, such as parts of sub-Saharan Africa, biogas initiatives can provide an off-grid energy solution, improving the quality of life for many households.
Potential Impact on Future Research Directions
Future research can delve into optimizing biogas designs to enhance gas production rates and exploring diverse feedstock beyond the commonly known materials, such as livestock manure and kitchen waste. Innovations in anaerobic digestion processes and biogas collection methods can further encourage adoption by improving efficiency and reducing costs. This presents an intriguing avenue for new technologies and community-driven solutions to emerge in the field of renewable energy.
Prelude to Household Biogas Plants
The relevance of household biogas plants has never been more pronounced. In an era where sustainable energy solutions are urgently needed, these systems emerge as a beacon of hope for both individual households and communities at large. The various aspects of biogas production, from its design to its implementation, hold promise in addressing not just waste management issues but also energy challenges prevalent in many parts of the world. Here, we plunge into the core of this technology, underscoring its multi-faceted benefits and practical considerations.
Definition and Purpose
Household biogas plants, at their essence, are systems that convert organic waste materials into biogas through a process called anaerobic digestion. This gas, primarily composed of methane, serves as a clean fuel alternative for cooking, heating, and even electricity generation. The purpose behind adopting such systems extends beyond mere energy production; it encompasses waste reduction, resource sustainability, and fostering a circular economy. In rural areas, for instance, these plants transform farm waste into useful energy, creating a significant shift from dependency on fossil fuels. This not only reduces carbon footprints but also cultivates an environment of self-sufficiency.
Historical Development
The journey of biogas utilization can be traced back to ancient civilizations, where natural gas was harnessed from decaying matter. However, the modern concepts of biogas plants began taking shape in the late 19th century when scientists started to explore anaerobic processes more rigorously. The first such structures were small and rudimentary, mainly designed to demonstrate the principle of biogas production. As the years progressed into the 20th century, various countries embraced biogas technology, recognizing its utility during tough economic times, particularly in post-war Europe and developing nations where energy resources were scarce.
Research and advancements during the 1970s and 1980s marked a crucial turning point in the efficiency and effectiveness of biogas systems. Governments began to support their development due to the energy crisis and environmental discussions emerging at that time. With modern engineering practices, today’s household biogas systems are more efficient, user-friendly, and customizable to fit different socio-economic contexts. Recognizing a need for cleaner energy sources, many nations have since implemented policies encouraging the adoption of biogas technologies, leading to a renaissance in interest for this versatile energy source.
The Science Behind Biogas Production
The field of biogas production stands as a fascinating intersection of biology, chemistry, and energy. This science explains how organic material can be transformed into useful energy sources while simultaneously addressing waste management. Understanding this science underpins the practical applications of biogas systems in households. It highlights the mechanisms behind energy generation and its relevance to sustainability, making it a crucial component of this article.
Anaerobic Digestion Process
The anaerobic digestion process is central to how biogas is produced. It happens without oxygen and involves breaking down organic matter by microorganisms. Understanding this process is essential for optimizing biogas production.
Microbial Activity
Microbial activity is fundamental to this process. Microbes are the unseen heroes, deftly consuming organic material and producing biogas as a byproduct. This activity is characterized by its efficiency and ability to break down complex substances into simpler forms. The structural diversity of microbial communities leads to various metabolic pathways that optimize biogas yield.
From a practical standpoint, the ability of these microorganisms to thrive without oxygen makes microbial activity a popular choice in biogas systems. Since they can utilize a wide range of organic materials— from kitchen waste to agricultural byproducts—this flexibility is often seen as a big advantage. However, maintaining the optimal conditions for these microbes, like pH levels and temperature, can be challenging.
Stages of Digestion
The process of anaerobic digestion unfolds in distinct stages: hydrolysis, acidogenesis, acetogenesis, and methanogenesis. Each stage plays a critical role in the breakdown of organic materials. The hydrolysis phase, for example, involves converting complex carbohydrates into simpler sugars, essentially setting the stage for all subsequent processes.
The stages of digestion are beneficial for biogas production as they allow for a progressively deeper breakdown of organic matter. Each unique stage leverages the metabolic capabilities of different microorganisms, enhancing total gas output if done correctly. But coordinating these stages can also present its own set of challenges; imbalances in conditions can slow down overall digestion and reduce biogas yield.
Biogas Composition
Biogas primarily consists of methane and carbon dioxide, along with trace amounts of other gases. The composition of biogas is a reflection of the feedstock and the digesting conditions. Typically, methane, which is flammable and can be used for cooking or generating electricity, represents about 50-70% of the total gas produced.
Understanding biogas composition is crucial because the higher the methane content, the more valuable the gas for energy generation. This characteristic is a driving factor for optimizing feedstock combinations. However, variations in input material or digestion efficiency can lead to fluctuations in the composition, making monitoring an essential aspect of operating a biogas system effectively.
Key Biological Agents
The successful generation of biogas hinges not just on the process but also on the biological agents involved. A diverse group of microorganisms, particularly bacteria, play integral roles throughout the digestion process.
Bacteria Involved
The different groups of bacteria involved in anaerobic digestion contribute significantly to biogas production. For instance, cellulose-degrading bacteria break down plant material, while methanogens produce methane from the digested material. Each type of bacteria has its niche, making the microbial community highly specialized.
These specialized bacteria’s unique features enable them to target specific substrates, maximizing efficiency in biogas production. Nevertheless, the complexity of managing these diverse bacteria presents challenges; if one group falls out of balance, it can impact the entire gas production process.
Role of Enzymes
Enzymes are biological catalysts that aid in speeding up chemical reactions, thus playing a vital role in breaking down organic matter more effectively. They work in tandem with bacteria to facilitate the hydrolysis of complex compounds. This collaborative effort ensures that digestion progresses smoothly through the stages, enhancing biogas outputs.
Leveraging these enzymes can optimize the process, but relying on them comes with risks. Any disruption in enzyme activity— due to temperature changes or pH shifts—can hinder the overall production.
Influence of Temperature
Temperature influences every biological process in anaerobic digestion. Maintaining optimal temperature conditions (usually around 35-55°C) encourages the growth of the most effective microbial communities. Those conditions contribute to a robust energy output.
Conversely, fluctuations in temperature can derail the accustomed pace of digestion. A drop pops the brakes on microbial activity, while excessive heat could kill off sensitive bacteria. Understanding and controlling these temperature variables is thus essential for maximizing biogas production.
Biogas production through anaerobic digestion is a science that, when properly understood and applied, can lead to sustainable energy solutions and effective waste management.
Essential Components of Biogas Systems
When considering the establishment of a household biogas system, understanding the essential components is key. These elements play a crucial role in ensuring the functionality, efficiency, and sustainability of the system. From the digester's design to the gas storage solutions, each aspect contributes significantly to the overall performance and reliability of biogas operations.
Digester Design and Structure
The digester is the heart of a biogas system. It is where the anaerobic digestion occurs, transforming organic materials into biogas. The design and structure of digesters vary, but they all serve the same purpose: to facilitate the digestion process while containing the waste materials safely.
Types of digesters
There are several types of digesters, such as fixed dome, floating drum, and plug flow digesters.
- Fixed dome digesters are popular in many rural areas. Their key characteristic is the dome-shaped structure designed to withstand high pressure. The design allows for efficient gas collection.
- Floating drum digesters have a movable top that rises as biogas collects. This type provides an easy visual of gas production, yet it can be more costly due to the materials used.
- Plug flow digesters are ideal for high-solids feedstock, moving material in one direction, which promotes efficient digestion.
Each type brings its own unique features, advantages, and disadvantages depending on the location and specific needs of the user. It's crucial for potential users to choose the right type based on local conditions and goals.
Materials used
The materials used in constructing digesters affect their durability and efficiency. Common materials include concrete, steel, and flexible plastics.
- Concrete is a favored choice due to its longevity and resilience. It withstands harsh weather and is easily molded for different designs.
- Steel, while durable, must be treated to prevent corrosion in a moist environment. Its strength allows for larger structures but could potentially lead to higher costs.
- Flexible plastics are increasing in popularity, offering light weight and ease of installation, although they may have a shorter life span compared to concrete or steel.
Choosing the right material involves a balance between cost, durability, and the expected lifespan of the digester.
Size considerations
Size matters significantly in biogas digesters. The capacity of the digester must align with the volume of feedstock that a household can provide.
- Small digesters are suitable for households with limited waste output. They require less space but may not yield enough biogas for higher energy demands.
- Larger systems, while providing ample gas, necessitate more room and upfront investment. The key characteristic lies in enhancing gas production while also ensuring operational efficiency for the household.
- A unique aspect of sizing is the need for adaptability. As needs change—be it through an increase in household members or diversifying waste input—having a flexible design can save future costs.
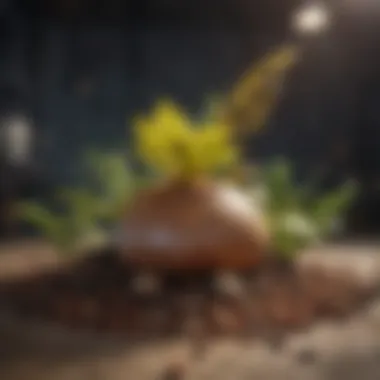
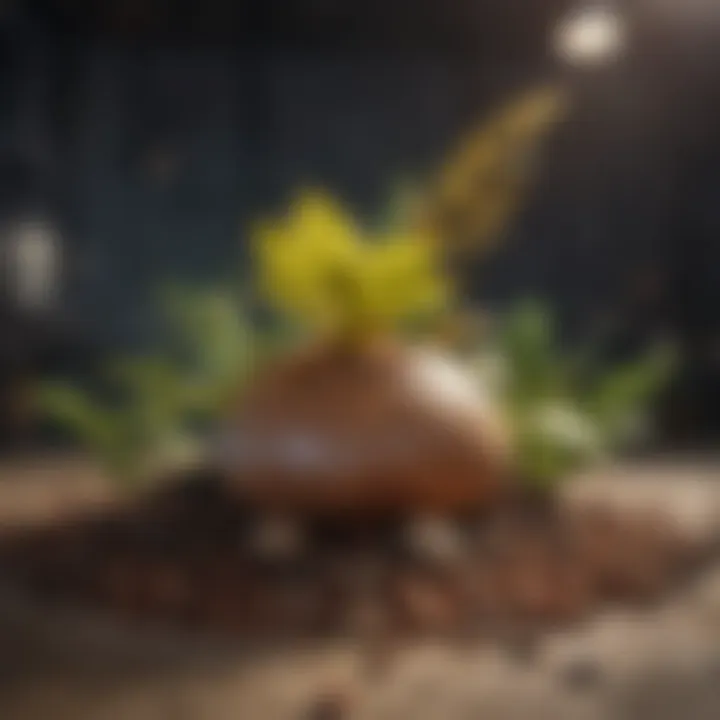
Gas Storage Solutions
Once biogas is produced, proper storage is vital. It ensures a steady supply for cooking, heating, or electricity.
Bags vs. tanks
When considering storage, biogas collection bags and tanks are popular options.
- Bags are often cheaper and easier to install. They flexibly expand with gas production. However, they may not hold as much gas as tanks and could be susceptible to punctures.
- Tanks, on the other hand, provide larger capacities and can be designed for high-pressure storage. Yet, they involve higher initial costs and more complex installation processes.
Assessing practicality and usability can help in deciding which storage solution fits the household's needs better.
Pressure considerations
Safety and efficiency hinge upon managing gas pressure levels effectively. The right amount of pressure can optimize gas availability for use.
- Low-pressure systems are easier to handle, making them safer for small-scale operations. However, maintaining flow and ensuring gas availability can be challenging.
- High-pressure systems allow for larger gas volume and more energy output but require more robust infrastructure and safety measures in place.
Careful monitoring and regular checks can help assess whether the pressure systems are functioning optimally.
Safety measures
Mitigating risks and ensuring safe operations is non-negotiable in biogas systems. Essential measures include:
- Installation of pressure relief valves to prevent excess pressure buildup.
- Routine maintenance checks, which detect issues before they escalate into significant hazards.
- Proper signage around the biogas storage area can warn individuals of potential risks and promote safety awareness in the community.
Feedstock for Biogas Production
Understanding the feedstock for biogas production is like holding the key to the entire process of energy generation in household biogas plants. The type and quality of organic materials processed play a crucial role in determining the efficiency and output of biogas systems. Choosing the right feedstock can not only maximize the energy yielded but also ensure a balanced nutrient supply for any subsequent agricultural applications.
Organic Materials Suitable for Digestion
Agricultural waste
Agricultural waste, such as crop residues and leftover plant material, is a vital contributor to biogas production. One might say it’s a true farmhand in the biogas process. This organic material is not only abundant but also easy to source. The high fiber content is very engaging for the bacteria involved in anaerobic digestion, making this feedstock a popular choice.
A unique aspect of agricultural waste is its diverse forms – it can include straw, husks, and even leaves. These materials contain essential nutrients and can have varying digestion rates, depending on their composition. The main advantage of using agricultural waste lies in its ability to reduce landfill burdens while simultaneously fostering energy generation. On the flip side, the variability in quality can lead to inconsistent biogas yields unless monitored properly.
Food waste
Food waste presents another rich opportunity for biogas production, transforming leftovers into energy. It often includes spoiled fruits, vegetable peels, and even leftover meals, which may otherwise contribute to municipal waste challenges. The key characteristic that makes food waste a beneficial choice is its high moisture content, which facilitates easier digestion and accelerates the biogas production process.
Moreover, food waste offers a unique blend of carbohydrates and proteins that significantly enhances the overall efficiency of the anaerobic digestion process. However, one must be careful with the inclusion of oily or fatty foods, as they could cause operational issues in the digester. Balancing the feedstock to avoid excess fats is essential to ensure smooth functioning and optimal output.
Manure types
Manure types, whether from cows, pigs, or chickens, serve as another noteworthy feedstock for biogas systems. They can be a powerhouse of nutrients — a true feast for the microorganisms. The key characteristic of manure is its inherent balance of carbon and nitrogen, which is essential in producing methane through digestion. Manure is particularly advantageous because it’s often a readily available resource, particularly in agricultural regions.
A unique feature of manure is its ability to be combined with other organic materials to create an ideal feedstock blend. However, high solid content can sometimes pose digestion challenges, necessitating pre-treatment steps to break it down effectively. Thus, while manure provides excellent prospects for biogas production, proper management is crucial to yielding optimal results.
Optimal Feedstock Combinations
Selecting the right mix of feedstock can significantly influence the efficiency of biogas plants. It’s akin to creating a recipe; every ingredient must be in harmony to achieve a delectable outcome.
Carbon-to-nitrogen ratio
The carbon-to-nitrogen ratio is of utmost importance in biogas production. This ratio defines the balance between carbon-rich and nitrogen-rich materials in a mixture. A proper balance, ideally around 25:1 to 30:1, tends to favor optimal microbial activity. Such balance encourages bacteria efficiency, resulting in increased methane production.
One might say a good carbon-to-nitrogen ratio is like a dance; too much of one partner can throw off the rhythm. If nitrogen is in excess, it can lead to ammonia accumulation, which may inhibit digestion. Hence, achieving this delicate balance is pivotal for maximizing outputs.
Moisture content
Moisture content is another critical factor that won’t let you down when it comes to biogas production. Bacteria thrive in a moist environment, making it essential to ensure feedstock contains adequate water – generally between 80% to 90% moisture. This is particularly important for dry materials that could otherwise hinder the digestion process.
However, excessive moisture can lead to dilution of nutrients and epending problems in gas collection. Therefore, managing moisture content is a balancing act. Ideally, feedstock should be treated to maintain that sweet spot conducive to microbial growth.
Pre-treatment methods
Pre-treatment methods are often employed to enhance feedstock digestibility, making them absolutely key for improving biogas yields. Techniques such as thermal, chemical, or biological treatments can effectively break down tougher materials, making nutrients more accessible to the microbes.
By employing pre-treatment, one can maximize the potential of otherwise challenging feedstocks. However, the drawbacks include possible increased costs and complexity of the process, which make careful assessment necessary. Adopting pre-treatment methods can be worth the weight if employed wisely.
Benefits of Household Biogas Plants
Understanding the benefits of household biogas plants is crucial for appreciating their role in renewable energy and sustainable living. These systems not only provide energy but also contribute significantly in areas such as waste management, nutrient recycling, and greenhouse gas reduction. The advantages they bring to a household's daily life reflect a blend of environmental responsibility and economic efficacy, making them a compelling choice for modern living.
Renewable Energy Source
Impact on energy bills
Household biogas plants can notably lighten the financial burden associated with energy bills. By producing biogas, families can harness a source of energy that directly reduces reliance on conventional electricity and gas supplies. The shift can alleviate monthly expenses significantly, especially for those in regions where energy costs continue to climb.
The key characteristic here is the customization; households can tailor the system to their own waste production, resulting in a flexible and potentially self-sustaining energy source. By maximizing the waste generated, whether from kitchen scraps or agricultural by-products, families can create enough gas for cooking or even heating, making it a beneficial arrangement.
Environmental advantages
The environmental benefits of biogas systems are underscored by their ability to mitigate waste disposal problems. When organic waste decomposes, it usually produces methane, a potent greenhouse gas. However, by channeling this waste into a biogas digestor, households can capture the methane and utilize it, thereby reducing harmful emissions.
What makes this approach particularly appealing is its dual function. Houses can solve waste management issues and simultaneously produce energy. This not only conserves resources but also promotes a cleaner planet—a vital component in today’s quest for sustainability.
Reduction of fossil fuel dependency
Biogas plants stand as a promising alternative to conventional fossil fuels, contributing to a lower carbon footprint. Their use helps in promoting energy independence; instead of relying on imported oil or gas, homes can rely on what they already have—organic waste.
This shift not only entails energy self-sufficiency but also aligns with broader environmental goals of reducing dependency on non-renewables. In doing so, households can play a role in fostering a healthier planet for future generations while promoting innovative energy solutions.
Nutrient-Rich Fertilizer Production
Digestate as fertilizer
One intriguing aspect of biogas systems is the digestate produced following anaerobic digestion. This by-product is nutrient-rich and serves as an excellent alternative to chemical fertilizers. It complements soil health, enhancing fertility through natural means. Households can employ this digestate in their gardens, generating a sustainable cycle of nutrient utilization.
The common observation here is that the digestate is cost-effective. Without the need to purchase commercial fertilizers, homeowners can improve their soil quality while maintaining a healthy budget.
Soil health improvement
Integrating digestate into soil management practices directly contributes to enhanced soil health. By enriching soil with organic matter, the digestate boosts microbial growth, improving soil structure and water retention. This is particularly vital in areas suffering from soil degradation, as it aids in restoring balance and fertility.
Soil's increased biodiversity is a vital benefit of using digestate. Such improvements contribute to healthier crop yields, which can be pivotal for households looking for self-sufficiency in food production.
Sustainable agriculture practices
The practices that stem from using household biogas systems dovetail with sustainable agriculture. By creating a closed-loop system, where waste becomes an input for food production, households contribute to a more responsible approach to agriculture.
This holistic method emphasizes sustainability, paving the way for resilient farming practices that can withstand environmental changes. By supporting biodiversity and reducing dependence on synthetic inputs, families take important strides towards ecological balance in their immediate surroundings.
"Investing in household biogas is not just a step towards energy efficiency; it can shape an eco-conscious community focused on sustainability and resilience."
In summary, the benefits associated with household biogas plants highlight their relevance not only in energy generation but also in ensuring a cleaner environment and improved agricultural practices. These systems serve as invaluable tools for both the present and the future, underpinning a promising path towards sustainability.
Economic Considerations
Initial Investment vs. Long-Term Savings
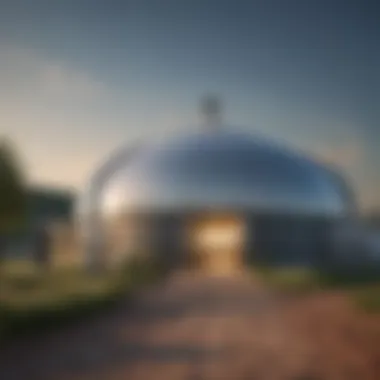
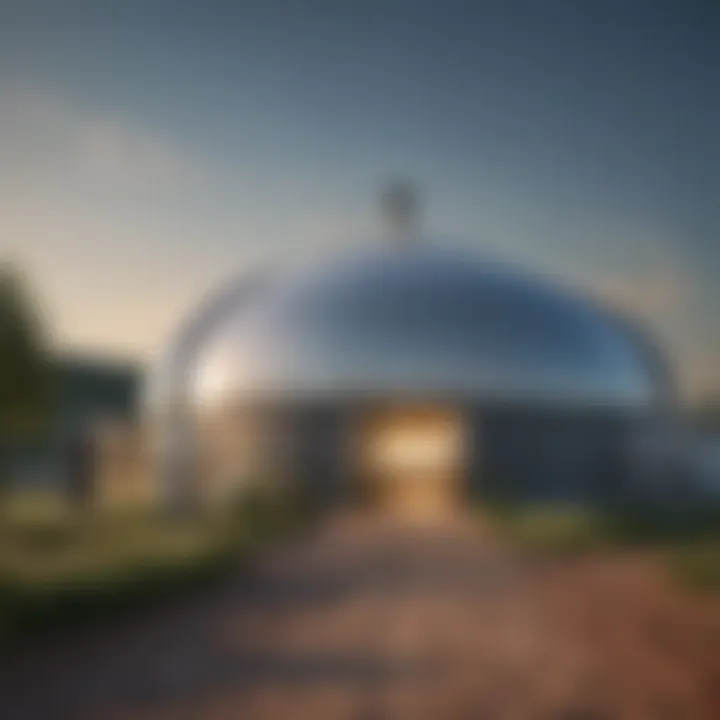
Cost analysis
Cost analysis helps potential users of household biogas plants understand the financial implications associated with installation and operation. It's the backbone of any investment decision. When evaluating costs, folks need to think about the initial investment—things like the digester, piping, and any technology required.
For those looking to adopt biogas solutions, a prevalent choice in this analysis is the breakdown of costs into components. This method clearly illustrates where the money will go and what the ongoing expenses might be, which aids in budgeting for the future. It’s tailored to show that biogas systems can offer a great return on investment over time.
Unique aspects of cost analysis include its capability to project not only upfront costs but also potential savings from reduced utility bills and even the sales of surplus energy or fertilizer. However, while the initial investment can be a tough pill to swallow, the long-term savings might just cover that cost in the blink of an eye.
Financing options
Financing options are a critical piece in the puzzle for many interested in biogas energy. They offer a pathway to make the necessary upfront costs less burdensome. This can involve taking out loans, leasing equipment, or looking into pay-as-you-go schemes tailored for renewable energy projects.
Looking closely, one popular aspect is the accessibility of many financing options for residential projects. They can simplify the initial costs by stretching payments over time and make it feasible for more households to engage.
However, it's essential to scrutinize the terms of these options, as certain financing routes might come with higher interest rates or hidden fees. Buyers should weigh these factors against the benefits of reduced energy costs and environmental impact.
ROI calculations
Return on Investment calculations are vital for those contemplating household biogas systems. ROI gives insight into how long it will take for an investment to pay for itself, which can significantly sway decision-making.
The key feature of ROI calculations is their straightforward nature, providing clarity on whether pursuing biogas is an economically sound decision. For instance, by estimating energy savings, government incentives, and potential revenue from biogas byproducts, one can get a clearer picture.
Nevertheless, the downside could arise if these calculations don’t account for fluctuations in energy prices or possible maintenance costs that stack up over time. It’s a balancing act of sorts, requiring a keen eye to ensure that figures align with expectations.
Government Incentives and Support
Subsidies available
Subsidies are perhaps one of the most effective tools promoted by governments to support renewable energy initiatives like household biogas systems. The money provided can significantly alleviate the burden of upfront costs. Often, there are specific grants that are solely aimed at biogas plant installation.
A significant advantage with subsidies is their ability to encourage adoption among those who might be on the fence due to financial concerns. Not to mention, they also facilitate a more rapid transition away from fossil fuels. However, eligibility can sometimes be a hurdle, as not all programs are accessible to every locality, making it imperative to thoroughly investigate regional options.
Tax benefits
Tax benefits can act as a cherry on top for households implementing biogas plants. The concept revolves around reduced taxes or deductions available to those who invest in renewable energy technologies.
It’s a noteworthy aspect because it can lead to substantial savings over the years, lightening the financial load for households while simultaneously incentivizing cleaner energy choices. However, accessing these benefits can sometimes involve complex procedures, often leaving potential applicants feeling a bit lost in the shuffle.
Grants and aid programs
Grants and aid programs are another essential aspect of the support landscape for biogas adoption. These can provide non-repayable cash, making them particularly attractive for prospective users. Organizations or governments may sometimes even provide training alongside funding, bridging the knowledge gap.
One unique characteristic of these programs is their targeting towards specific user groups—like low-income families or rural areas—ensuring that help is directed where it is needed the most. While they provide great opportunities, navigating the application process can be daunting. Keeping track of deadlines and criteria is crucial for beneficiaries to reap these benefits.
Social Implications of Biogas Adoption
Embracing biogas technology goes beyond mere energy generation; it touches upon the very fabric of social well-being. Household biogas plants present a canvas that can paint a picture of healthier communities, educational advancements, and improved resilience. It’s not just about turning waste into fuel—it's about elevating the quality of life as well.
Community Health Benefits
Reduction of pollution
The impact of biogas plants on reducing pollution is significant. Waste management is a gargantuan task, especially in regions where landfill options thin out. With anaerobic digestion, organic matter is transformed, preventing the release of harmful greenhouse gases and leachate that often escape into the environment. This reduction in pollution contributes to cleaner air and water, pivotal elements for a thriving community. A prime characteristic of pollution reduction lies in its dual benefit; it not only curbs environmental degradation but also promotes public health.
The unique feature of this process is its ability to tackle waste at the source, using local resources to generate renewable energy while minimizing risks associated with waste decomposition. However, while the advantages are evident, implementing biogas technology may require initial community buy-in and educational programs to maximize effectiveness.
Public health outcomes
When we discuss public health outcomes linked to biogas adoption, we uncover a link to diminished disease prevalence. By effectively managing waste, we aim to reduce vectors such as mosquitoes that thrive in stagnant waste environments. Cleaner surroundings enhance overall health standards, leading to less respiratory illness and other health complications. One of the key attributes of enhanced public health outcomes is the immediate nature of these benefits—communities witness change almost instantaneously after the implementation of biogas systems.
A notable angle to consider is the long-term effects. While initial setups can seem daunting, the sustained benefits often outweigh temporary inconveniences. In essence, healthier populations translate to lower healthcare costs.
Community resilience
Building community resilience forms another pillar of biogas’ social implications. As communities adopt biogas systems, they foster independence from external energy sources, making them more self-sufficient. Being able to process waste locally transforms communities from passive observers to active participants in renewable energy production. The very nature of this empowerment helps forge stronger community ties.
A defining characteristic of community resilience is adaptability; households become more flexible in responding to energy demands or crises. One unique aspect of establishing biogas systems is the ripple effect. The engagement of community members in this initiative often leads to other collaborative ventures that address local challenges, further enhancing collective strength.
Educational Opportunities
Training programs
Effective training programs are fundamental. They educate community members on the operation and maintenance of biogas plants, ensuring sustainability. The very act of hands-on learning and skill development creates a sense of ownership. One key trait about training programs is their adaptability—resources can be tailored to specific community needs or contexts, optimizing relevance.
The unique feature here lies in the blend of practical knowledge and theoretical grounding. Programs can cover everything from basic maintenance to advanced troubleshooting of the systems, though some may find the variations daunting.
Community workshops
Workshops serve as a hotspot for knowledge exchange. They gather community members in a collective learning atmosphere, stimulating diverse perspectives and collaborative learning. The interactive nature of community workshops ensures that knowledge is accessible—even to those who might not be inclined toward formal education. A key characteristic is that they can forge relationships among participants, fostering a sense of unity.
However, while workshops can spark enthusiasm, they must be well-structured. Lack of clear objectives or overcomplicating content may hinder participation.
Knowledge dissemination
Knowledge dissemination revolves around the flow of information regarding biogas systems and their benefits. Effective strategies may include online platforms or community bulletins detailing best practices. An important characteristic is the focus on communication; the more accessible information is, the more likely communities are to embrace this technology.
A unique aspect of this approach is the continuous engagement post-implementation. By sustaining interest and providing updates, communities can adapt and evolve their practices, ensuring long-term success. Yet, with knowledge comes the risk of misinformation, underscoring the need for reliable sources.
In summary, the social implications of biogas adoption highlight a path toward healthier, more resilient communities equipped with the knowledge and tools necessary to thrive in a sustainable framework.
Global Perspectives on Biogas Adoption
Adopting biogas technology is not just about generating energy; it’s a multifaceted approach that touches on sustainability, waste management, and social good. Each region brings its own flavor to the biogas table, showcasing unique challenges and successes. Understanding these global perspectives can inform local implementations and highlight the versatility of biogas solutions across different contexts.
Case Studies from Different Regions
Success stories
One of the standout tales comes from India, where a village in Gujarat revolutionized its energy approach through a community biogas initiative. This project enabled households to convert food waste and cow dung into biogas, providing an inexpensive energy source while simultaneously reducing waste. The key characteristic here is community involvement; by engaging residents, the initiative not only promoted renewable energy usage but also fostered a sense of shared ownership among participants.
Such collaborative efforts stimulate both economic development and local empowerment—a powerful combo in a region striving for greater self-sufficiency. Community biogas digesters emerged as a beacon of sustainability, and these models teach us that local solutions can be potent catalysts for change. What is unique about this endeavor is its replication potential. Villages elsewhere can adopt similar models to suit their particular contexts, bolstering the expansion of biogas usage across the nation.
Challenges faced
Not all stories of biogas adoption are wholly positive, though. In Kenya, for instance, many farmers have faced substantial technical and financial hurdles. The upfront costs to set up systems can deter potential users, especially in rural areas where incomes are modest. Moreover, lack of skilled labor to install and maintain biogas plants presents another layer of complexity.
Highlighting the fact that technical expertise is crucial for a successful implementation, some farmers have resorted to makeshift repairs or even abandoned their systems altogether, leading to loss of investments. The unique feature of this scenario lies in the importance of education and training. Addressing these challenges by establishing training programs can significantly improve the prospects for biogas technology in similar environments.
Lessons learned
Across the globe, experiences with biogas have led to several valuable lessons. One notable lesson stems from a project in Germany, where comprehensive policy support and public awareness campaigns were pivotal for successful biogas implementation. Here, legislation provided clarity and incentives, ensuring that biogas plants could thrive as part of a broader renewable energy strategy.
The key takeaway revolves around the necessity of supportive policy frameworks. This situation emphasizes the importance of aligning local initiatives with broader governmental objectives to ensure sustainability. Additionally, it teaches that stakeholder engagement —from local farmers to governmental bodies—has essential implications for overcoming resistance and fostering growth.
Comparative Analysis with Other Renewable Technologies
Solar vs. biogas
When comparing solar energy to biogas production, the first thing that springs to mind is intermittency. Solar energy is prone to fluctuation depending on weather conditions, while biogas offers a consistent energy output. Some households have found it advantageous to combine both systems, harnessing solar power for electricity and biogas for heat and cooking. This synergy ensures that renewable energy can meet daily requirements, rain or shine.
The remarkable aspect of the solar-biogas combination lies in area utilization. Land used for growing crop feedstocks for biogas can also host solar panels. Thus, this integrative approach maximizes renewable energy potential while also promoting land efficiency, showcasing why both technologies can coexist harmoniously.
Wind energy integration
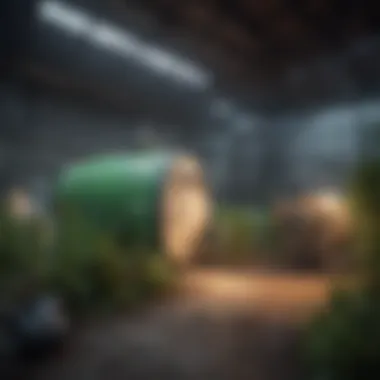
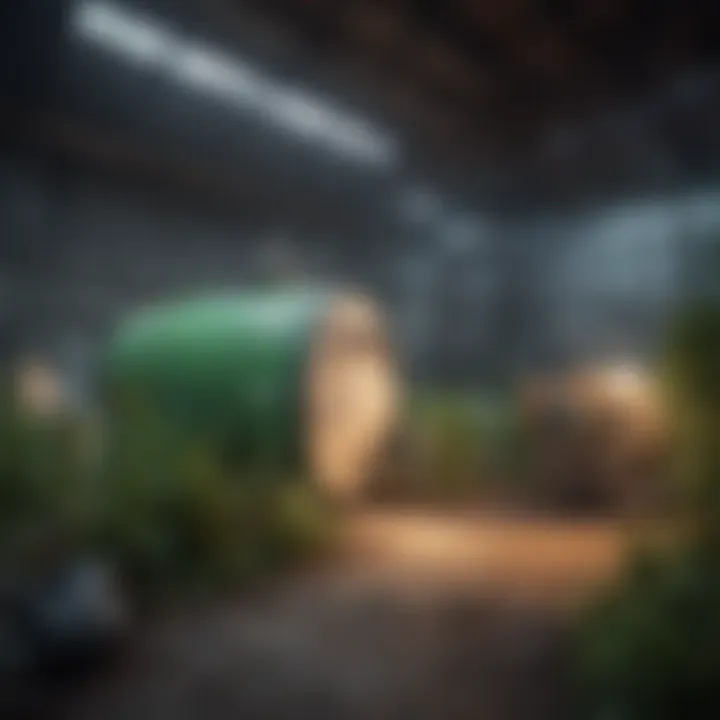
Integrating biogas systems with wind energy is yet another avenue that communities are exploring. Wind energy offers another reliable renewable source, yet is often constrained by geographical limitations. In contrast, biogas can be established almost anywhere.
By pairing biogas with wind systems, especially in windy coastal regions, users can ensure stable energy supply, capitalizing on two complementary resources. Additionally, this dual approach can lead to enhancement in overall energy independence, positioning regions better for future needs.
Hybrid systems
Hybrid systems that incorporate multiple renewable energies are becoming increasingly popular. For example, areas with ample sunlight and significant agricultural output can synchronously use solar panels and biogas systems, thus diversifying energy sources. These combinations not only amplify energy security but also optimize resource use by channeling waste into productive systems.
Ultimately, engaging with hybrid systems offers a pathway toward sustainability. They call for thoughtful planning and local ingenuity to determine which mix works best in a given context. The ability to tailor energy solutions to fit specific needs is a quality that can redefine the renewable energy landscape.
Exploring global perspectives on biogas adoption opens a treasure trove of insights for individuals and communities eager to adopt sustainable practices. Missed opportunities to learn from diverse experiences can leave potential benefits untapped.
Installation Process and Challenges
Installing a household biogas system is no piece of cake, but when done right, it's like striking gold. This section not only walks through the important steps but also dives into the challenges faced along the way. Understanding these can mean the difference between a smoothly running system and a heap of trouble down the line. Here’s a closer look at this essential aspect of biogas plants.
Steps for Installation
Site selection
Selecting the right site is the bedrock of your biogas plant's success. Placement matters. The ideal location should be accessible for maintenance but also separated from living quarters due to odor concerns. An easily accessible spot allows for hassle-free loading of feedstock - that’s the organic matter you’ll be turning into biogas.
A key characteristic of site selection is that it should offer good drainage; you don’t want your facility turning into a swamp after a heavy rain. The unique feature of this aspect is that it greatly influences cost too, since establishing infrastructure on uneven or poorly chosen land can hike expenses significantly, leading to future headaches. If you have the chance to pick a location with plenty of sunlight, that’s a bonus, as it allows for potential solar integration later on.
Permitting requirements
Before the cement is poured and the pipes laid down, securing permits can feel like a bureaucratic gauntlet. In most places, local governments demand permits to ensure environmental safety and compliance with regulations. This can often delay the installation timeline and may require adjustments to your original plans.
A key feature of understanding permitting requirements is that knowing the rules can save you from costly fines down the road. These regulations can vary widely, turning a seemingly simple project into a legal quagmire at times. On the flip side, being diligent about grabbing the right permits can also lend credibility and resilience to your project, opening doors to potential funding down the road.
Construction phase
Once you’ve got your site and permits, it’s time for the nitty-gritty: construction. Here, careful execution is everything. The construction phase is where your ideas start taking shape, and each step—from digging the digester pit to constructing gas storage systems—must adhere to the original designs that you’ve painstakingly drawn up.
A valuable characteristic of this phase is that flexibility can often be needed. Weather conditions, specialized material availability, or even unanticipated site challenges can call for quick thinking and adjustments. One unique feature of construction for biogas plants is that you might have to collaborate with multiple skilled trades, including plumbers and electricians. The ability to navigate these interactions is crucial but can also lead to delays if not managed correctly.
Maintenance and Management of Biogas Plants
Effective maintenance and management of biogas systems are crucial for ensuring their longevity and optimal performance. Without regular oversight, a biogas plant can face problems that may lead to inefficiencies or complete shutdowns. Thus, a well-planned maintenance strategy can greatly influence the success of household biogas plants by safeguarding investments and contributing to a more sustainable energy future. The ongoing upkeep can also extend operational lifespans, while proactive management practices can help in identifying issues before they turn critical.
Routine Maintenance Procedures
Cleaning protocols
Cleaning is not just a matter of aesthetics; it's an essential practice that significantly impacts the performance of biogas systems. Regularly cleaning the digester and associated equipment prevents the buildup of undesirable materials. This cleaning ensures that everything processes smoothly and reduces the risk of blockages that could halt biogas production. A major feature of effective cleaning protocols is their ability to keep microbial activity at optimal levels. By mitigating the risk of potential contamination and maintaining an efficient environment for bacteria, cleaning protocols provide a vital foundation for biogas production.
However, the task of cleaning can be labor-intensive and may sometimes incur additional costs. Still, the benefits of consistent upkeep generally outweigh these drawbacks, illustrating the importance of this crucial maintenance step.
Daily operations
Daily operations encompass the routine tasks and checks that contribute to the efficient running of a biogas plant. These consist of monitoring gas production levels, inspecting equipment, and ensuring the proper flow of feedstock into the system. Establishing a structured daily routine can enhance efficiency by allowing for prompt detection of any anomalies. One of the key characteristics of daily operations is their role in maintaining a steady output of biogas. Having a reliable operational routine ensures that the system runs like a well-oiled machine.
On the downside, maintaining effective daily operations can demand consistent commitment, which might be tough for those with full schedules. Still, the smooth function of the biogas plant often hinges on thorough and diligent daily monitoring, making this aspect indispensable.
Monitoring performance
Monitoring performance revolves around assessing the operational efficiency of the biogas plant over time. This involves keeping track of various metrics, such as the volume of gas produced, digestate quality, and the surrounding environmental conditions. Strong monitoring practices act as a guardrail that keeps the biogas system operating within defined parameters, preventing potential disasters.
A unique aspect of effective performance monitoring is the use of data analysis and visualization tools that can provide insights into system health. Regular data checks help pinpoint areas needing improvement, promoting an optimized process. However, some users might find the establishment of such monitoring systems overly complex or demanding of resources. Nonetheless, the insights gained from well-executed performance monitoring hold significant value, clearly justifying any investment made in this area.
Troubleshooting Common Issues
Troubleshooting in biogas systems addresses the inevitable problems that can arise during operation. A proactive approach can mitigate potential failures and save considerable resources in the long run.
Identifying faults
Identifying faults is a critical element of troubleshooting, as it allows operators to diagnose problems early on. This proactive identification can safeguard against catastrophic failures that could result from ignoring warning signs, thus it’s a popular choice in maintaining the integrity of the system. A major advantage of focusing on identifying faults is that it fosters a better understanding of system dynamics, allowing operators to respond swiftly to emerging issues.
Still, the challenge comes in the form of the experience required for accurate fault identification. A lack of knowledge may lead to misdiagnosis or delayed action, underlining the necessity for continuous learning and experience in this domain.
Preventive measures
Preventive measures are designed to anticipate and address potential issues before they manifest into significant problems. This might include regular system evaluations, calibration of sensors, and checking for leaks in gas storage units. This approach tends to be a favored method, as it’s more cost-effective than dealing with large-scale repairs after a failure.
However, implementing preventive strategies can often require careful planning and consistency, which some may find challenging given the unpredictability of everyday life. Yet, the reduced downtime and maintenance costs typically make this an advantageous route overall.
Repair strategies
Lastly, any biogas plant must be equipped with effective repair strategies to respond to flaws or damages. This includes having a clear plan for repairing equipment and identifying a reliable source of spare parts. Repair strategies can be a double-edged sword; they are vital for addressing issues but may incur significant costs and downtime during repairs.
Successful repair strategies often involve accepted practices that can restore systems to full functionality without undue delay. However, the need for specialized knowledge and tools may act as a barrier for some operators. Overall, a well-thought-out approach can ensure that the plant remains operational, highlighting the importance of repair readiness in maintaining system efficiency.
Future of Household Biogas Systems
The future of household biogas systems is not just a tale of tech advancements; it’s a real game-changer that holds the potential to reshape energy dynamics at the local level. With pressing environmental issues and rising energy costs, these systems are increasingly relevant. The environmental benefits paired with economic opportunities make it a hot topic in discussions around sustainability and energy independence. As society pushes for greener alternatives, household biogas systems emerge as a promising answer, connecting waste management with energy generation.
Technological Innovations
Advancements in digester design
The landscape of biogas systems is evolving, especially in digester design. Recent innovations have introduced modular and compact digesters that can fit within various household sizes and conditions. The key characteristic of these advancements is the ability to efficiently process a wider array of feedstock, ranging from kitchen scraps to agricultural residues.
A standout feature of modern digesters is their increased efficiency; they can yield more biogas per unit of input compared to traditional designs. However, this can also lead to complexities in calibration and management, making them less straightforward for the uninitiated.
Enhancements in gas utilization
Gas utilization improvements are vital for households seeking to maximize outputs from their biogas systems. Modern gas utilization technologies focus on converting raw biogas into a more refined product suitable for cooking, heating, or even electricity generation. The prominent feature of enhanced gas utilization systems is their ability to operate at higher pressures, allowing for greater energy yield.
This means that households can expect more energy from the same amount of input waste, although it does pose challenges in terms of safety and proper maintenance. The financial viability is clear: lower energy costs are possible if utilization efficiencies are realized.
Integration with smart technologies
Smart technology integration in biogas plants presents an exciting forefront. The incorporation of sensors and automated systems allows for real-time monitoring of digestion processes, which can lead to optimized biogas production. The key advantage of such integration is its ability to provide actionable data, guiding users on optimal feedstock management.
While this high-tech approach can provide significant benefits, it also necessitates a certain level of tech literacy that may be daunting for some users, limiting its accessibility.
Potential for Scaling Up
Community biogas initiatives
Community biogas initiatives are capturing attention due to their potential for transcending individual homes and creating a network of sustainable energy solutions. These initiatives, often driven by local governments or NGOs, aim to facilitate group investments in larger biogas systems. A major appeal of such programs is the shared resources and knowledge, making it feasible for more homes to tap into renewable energy.
However, the unique feature of community initiatives is the potential for localized energy independence, which can empower communities to reduce reliance on external energy supplies. The drawback, however, is the need for a strong community commitment and collaboration, which can vary widely.
Policy frameworks
Policy frameworks play a crucial role in the future of household biogas systems. Supportive legislation can foster an environment conducive to innovation and investment in biogas technology. A key characteristic of effective policy frameworks is their flexibility to adapt to regional needs and capacities.
Good policy not only facilitates funding but can also provide incentives for households to adopt biogas systems. An important consideration here is that poorly designed policies may lead to misallocation of resources or unintended outcomes that could stifle innovation instead of encouraging it.
Partnerships for success
Partnerships for success are fundamental to expanding the reach of biogas adoption. Collaborations between government, NGOs, and the private sector can bring in necessary expertise and funding. The key advantage of robust partnerships is the pooling of resources—be it in terms of knowledge, funding, or technology transfer.
What distinguishes effective partnerships is their ability to create tailored solutions that address both local conditions and broader sustainability goals. However, a potential disadvantage can be the complexities in management and coordination among diverse partners, which may slow down initiatives or lead to conflicts.
"The integration of biogas systems into community frameworks not only empowers individuals but also cultivates a broader culture of sustainability."
Through all these innovations and collaborative strategies, the future of household biogas systems appears promising. As challenges of waste management and energy scarcity reinforce the necessity for sustainable practices, these systems are likely to play a pivotal role in the energy landscape to come.