FTIR Characterization: Principles and Applications
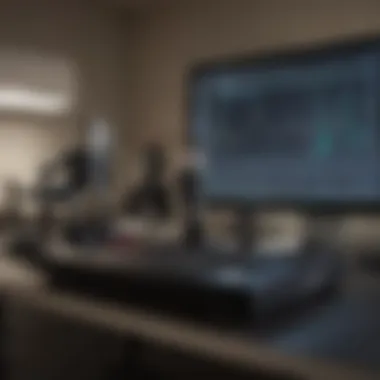
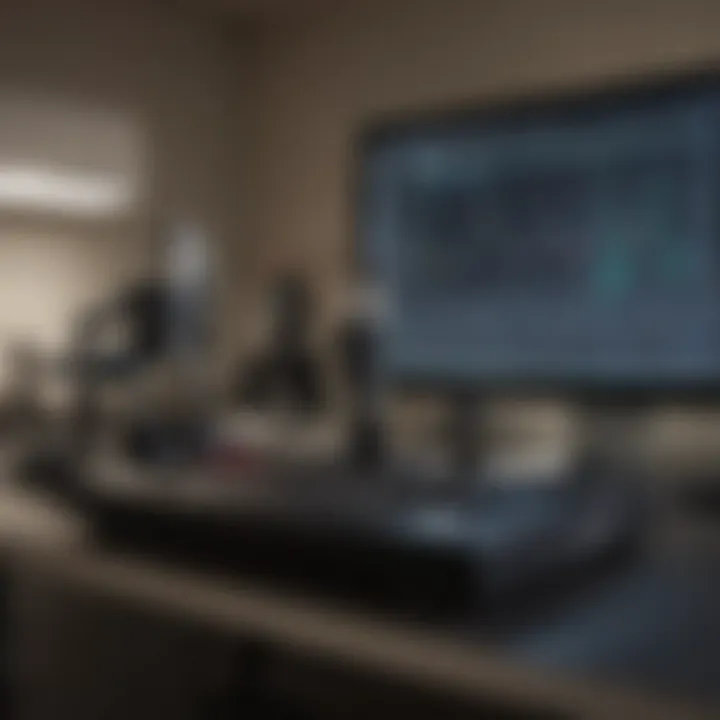
Intro
Fourier-transform infrared spectroscopy, commonly known as FTIR, serves as a groundbreaking tool in the field of material characterization. By analyzing how materials interact with infrared light, FTIR enables scientists to identify molecular structures and interactions. The technology’s versatility extends across various scientific domains, including chemistry, biology, and materials science. Understanding the underlying principles and mechanisms of FTIR is critical for researchers aiming to delve deeper into the molecular realm.
Key Findings
Summary of the Main Result
The examination of FTIR spectroscopy reveals several key findings relevant to researchers and professionals in the scientific community. Firstly, FTIR provides detailed vibrational spectra of molecules, allowing for the identification of functional groups and chemical bonds within samples. This provides a non-destructive way to assess material composition. Secondly, the advancements in FTIR technology enhance data acquisition speed and resolution, which improves the accuracy of molecular characterization.
Significance of Findings within the Scientific Community
The findings related to FTIR spectroscopy are significant due to their wide-ranging implications. For instance, the ability to analyze molecular interactions quickly and accurately can lead to innovations in drug development and materials engineering. Researchers can utilize the detailed information provided by FTIR to perform in situ studies of chemical reactions, shedding light on fundamental processes in both chemistry and biology.
"FTIR spectroscopy acts as a bridge between theory and application, facilitating new discoveries in materials science."
Implications of the Research
Applications of Findings in Real-World Scenarios
The practical implications of FTIR spectroscopy are profound. In chemistry, FTIR serves as a crucial method for analyzing organic compounds. This application aids in quality control for pharmaceuticals, ensuring the purity and consistency of active ingredients. In materials science, FTIR is employed to study polymers, composites, and nanomaterials, leading to improved material design and functionality. In biology, FTIR can aid in understanding protein folding and interactions, providing insights into biological processes.
Potential Impact on Future Research Directions
As FTIR technology continues to evolve, it is expected to impact future research directions significantly. Improved spectral resolution and emerging techniques, such as portable FTIR devices, may allow for field applications in environmental monitoring and biomedical diagnostics. The ongoing integration of FTIR with advanced computational techniques also holds promise for predictive modeling in molecular design. Thus, FTIR is not merely a characterization tool; it is a catalyst for innovation across multiple scientific domains.
Prologue to FTIR Characterization
Fourier-transform infrared spectroscopy (FTIR) is a powerful analytical tool employed for material characterization. This section lays the groundwork for understanding how FTIR operates and why it is essential in various scientific disciplines. The importance of FTIR is evident in its ability to provide molecular insights through vibrational spectra. FTIR effectively bridges the gap between theoretical concepts and practical applications, making it invaluable for researchers and professionals alike.
The immediate benefits of FTIR characterization include its versatility and non-destructive approach. By allowing rapid analysis of samples, FTIR has become a cornerstone in fields such as chemistry, biology, and materials science. It enables scientists to analyze complex mixtures, identify functional groups, and understand molecular interactions. Additionally, FTIR is crucial for developing new materials and improving existing ones.
Considerations for using FTIR effectively involve understanding its foundational principles and methods. Each aspect of FTIR, from its historical development to current applications, must be grasped to utilize this technology fully. The following sections will elaborate on these facets, including what FTIR is, how it has evolved, and its core mechanisms, which together provide a comprehensive overview of its role in scientific analysis.
What is FTIR?
FTIR, or Fourier-transform infrared spectroscopy, is a technique used to obtain an infrared spectrum of absorption or emission of a solid, liquid, or gas. The core principle of FTIR relies on the measurement of the wavelengths at which a substance absorbs infrared light. When infrared light interacts with matter, specific bonds within molecules absorb energy, leading to molecular vibrations. These vibrational events produce a unique spectral signature, serving as a fingerprint for various materials.
FTIR systems typically operate by directing infrared radiation through a sample and detecting the transmitted or reflected light. The output is analyzed using Fourier transformation, converting the raw data into a spectrum that can be further deciphered.
Historical Development of FTIR Techniques
The history of FTIR can be traced back to the development of infrared spectroscopy in the mid-20th century. Initially, early IR spectrometers utilized dispersive methods, which were slow and restricted in their analytical capabilities.
The true revolution in FTIR began in the late 1980s with the advent of Fourier-transform methodologies. This shift introduced significant improvements, allowing for faster scanning times and higher signal-to-noise ratios. As technology advanced, FTIR instruments became more sophisticated, incorporating computers and digital signal processing. Today, modern FTIR systems provide high-resolution data and have been adapted for a myriad of applications.
The evolution of FTIR techniques has not only transformed how materials are analyzed but has also facilitated innovations in research methodologies. As scientists continue to explore the potential of FTIR, the historical context remains vital in understanding its current relevance and future possibilities.
Fundamental Principles of FTIR
Understanding the fundamental principles of Fourier-transform infrared spectroscopy (FTIR) is essential for grasping its significance in scientific research. FTIR is not just a technique but a window into molecular environments, revealing information about chemical bonds and molecular structures. Mastering these principles can lead to successful applications in various fields such as materials science, biology, and environmental studies.
Basic Theory of Infrared Spectroscopy
Infrared spectroscopy leverages the interaction between infrared radiation and matter. When a molecule absorbs infrared light, it undergoes vibrational motions. Different bonds within the molecule resonate at specific frequencies. This characteristic response forms the basis for identifying molecular structures.
One of the key aspects is wavelength and frequency. Infrared radiation is present in several regions, with the mid-infrared range (approximately 4000 to 400 cm⁻¹) being particularly relevant for FTIR analysis.
This technique can be used to analyze gases, liquids, and solids. The data collected provides a spectrum that serves as a fingerprint for the material being studied. Researchers can decipher this fingerprint to gain insights into chemical composition and functional groups.
Fourier Transformation in Spectroscopy
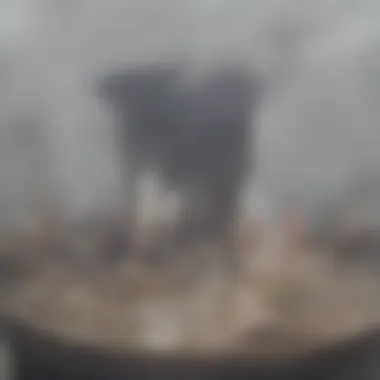
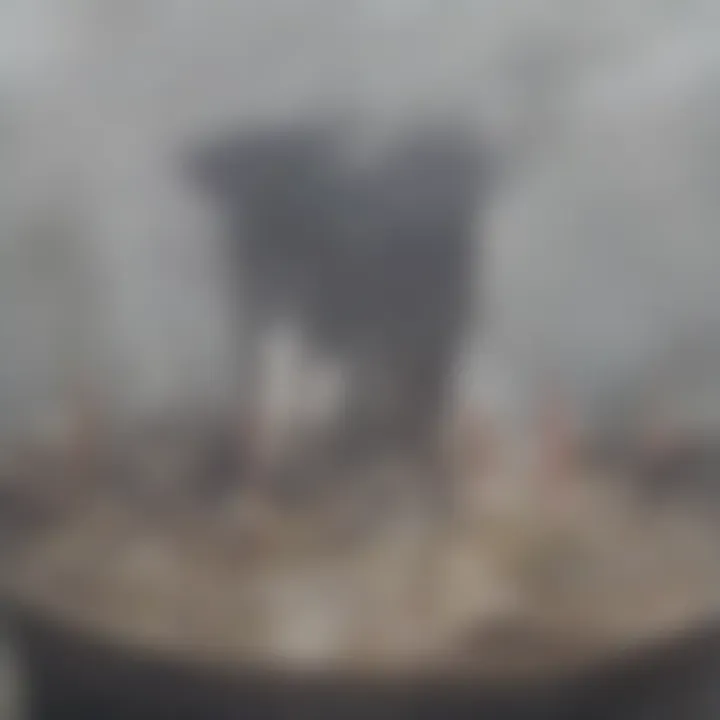
Fourier transformation is a mathematical process that converts time-domain data into frequency-domain data, which is crucial for interpreting spectra in FTIR. During an FTIR measurement, the time-domain signal from the interferometer is recorded. The Fourier transform is then applied to obtain the resulting spectrum, which depicts the intensity of the absorbed light versus frequency.
This step is essential for generating clear and interpretable results, making it easier to identify characteristics of the sample. Essentially, this transformation allows researchers to visualize complex data cohesively. Achieving accurate Fourier transformations is critical in ensuring precise spectral readings.
Vibrational Modes of Molecules
Molecular vibrations occur when the atoms within a molecule move relative to each other. FTIR specifically analyzes these vibrational modes, which can be categorized mainly into two types: stretching and bending.
- Stretching Modes: These involve changes in the distance between atomic bonds, either through elongation or contraction.
- Bending Modes: These occur when angles between bonds change, which can include scissoring, rocking, wagging, and twisting.
The vibrational modes are influenced by factors such as mass of the atoms and bond strength. This information is crucial in determining how different compounds behave and react under various conditions. Understanding these vibrations allows scientists to predict the behavior of substances in practical applications, enhancing the overall utility of FTIR spectroscopy.
Understanding the fundamental principles of FTIR equips researchers with the knowledge to effectively harness the technique for various scientific inquiries.
Instrumentation and Setup for FTIR
Understanding the instrumentation and setup for FTIR is essential for effectively utilizing this powerful analytical technique. The quality of data obtained from FTIR can be greatly influenced by the design and configuration of the instrument, as well as the methods employed during sample preparation and calibration. A well-structured approach ensures reliable and reproducible results, which ultimately enhances the scientific rigor of any research project.
Components of FTIR Instruments
At the core of FTIR spectroscopy lies a variety of components that collectively contribute to the effectiveness of the analysis. The main elements include:
- Infrared Source: This component generates a broad spectrum of IR wavelengths, essential for exciting the molecular vibrations in the sample. Common IR sources include globars or ceramic heating elements.
- Beam Splitter: This optical element divides the incoming light into two pathways. Typically made of materials like potassium bromide or mylar, its properties influence the spectral resolution of the analyzer.
- Interferometer: This section facilitates the conversion of IR light into an interferogram. Michelson interferometers are commonly used and are pivotal in Fourier transformation, which translates the time-domain signal into a frequency-domain spectrum.
- Detector: The detector measures the intensity of the IR light after it interacts with the sample. Common detector types include photoconductive detectors and thermocouples, which respond to varying temperatures or light intensities.
- Sample Holder: The arrangement in which samples are placed is critical for obtaining accurate results. Different holders are available for solid, liquid, and gas samples, ensuring appropriate interaction with IR radiation.
Understanding these components allows researchers to make informed choices about their FTIR setup that can significantly affect the quality of their analytical results.
Sample Preparation Techniques
Sample preparation is crucial to achieve optimal results in FTIR analysis. Proper technique ensures that the sample provides a good representation of the material being studied. Techniques vary depending on the physical state of the sample:
- Solid Samples: These often require grinding and mixing with a non-absorbing matrix such as potassium bromide. This combination forms a pellet that allows for better transmission of IR light.
- Liquid Samples: Appropriate cuvettes are essential. Short path lengths ensure that sufficient data is captured while minimizing scattering and absorption that can obscure results.
- Gaseous Samples: These can be analyzed using gas cells, which maintain a specific path length and allow for accurate absorption measurements. The management of sample concentration is vital in this preparation stage.
Ultimately, the goal of sample preparation is to create a conducive environment for IR analysis that yields clear, interpretable spectra.
Calibration and Maintenance of FTIR Instruments
For FTIR instruments, calibration and maintenance are fundamental practices ensuring that results remain accurate and reliable over time. Regular calibration accounts for any discrepancies that may develop in the system due to equipment wear or environmental changes.
- Daily Calibration: It is advisable to perform standard calibrations with known reference materials before commencing analysis to check the instrument's performance.
- Routine Maintenance: Regular checks on components like the IR source and detectors can prevent potential instrument failure. Cleaning optical surfaces and checking the alignment of optical paths is essential to maintain performance.
- Software Updates: Keeping the data processing software updated enhances analytical capabilities and introduces new features that can streamline data analysis processes.
By integrating these routines into the FTIR workflow, researchers can assure the integrity of their findings and ensure consistency across experimental procedures.
Data Acquisition Processes
The process of data acquisition in FTIR is fundamental to obtaining meaningful spectral data. It involves the collection of information through various techniques, ensuring accuracy and consistency in results. This section delves into scanning techniques, spectrum interpretation, and the challenges often encountered during data collection.
Scanning Techniques in FTIR
Scanning techniques are crucial as they define how spectral information is gathered. The primary scanning method in FTIR is the single beam and double beam methods.
- Single Beam Scanning: This method records the sample signal directly against a background. It is simpler but does not account for fluctuations in the light source.
- Double Beam Scanning: This technique measures the sample signal and the reference beam simultaneously. This compensates for any variations in the light source or detector response and enhances the precision of the results.
Also, the FTIR spectrometer uses an IR beam split by a beamsplitter into two paths. One reflects off a fixed mirror while the other reflects off a movable mirror. The interference of these beams generates an interferogram, which is then transformed using the Fourier Transform into a spectrum.
A careful selection of scanning parameters greatly influence the data quality. The resolution and scan speed must be optimized depending on the sample characteristics and the specific application. High resolution captures more detail but requires longer scanning time. Conversely, faster scans can lead to loss of detail but are useful for dynamic measurements.
Interpreting FTIR Spectra
Interpreting FTIR spectra is where the data acquisition meets analytical chemistry. Each spectrum represents a unique molecular fingerprint, allowing for identification and characterization of materials. Spectra consist of peaks that correspond to vibrational modes of molecular bonds. The position and intensity of these peaks reveal valuable information about the sample's functional groups and molecular structure.
To interpret the spectrum:
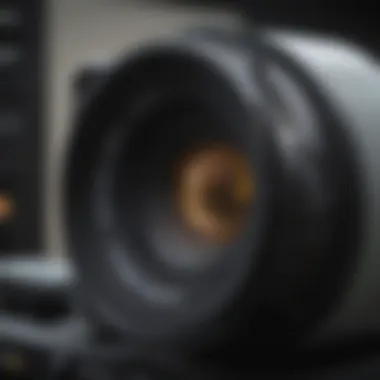
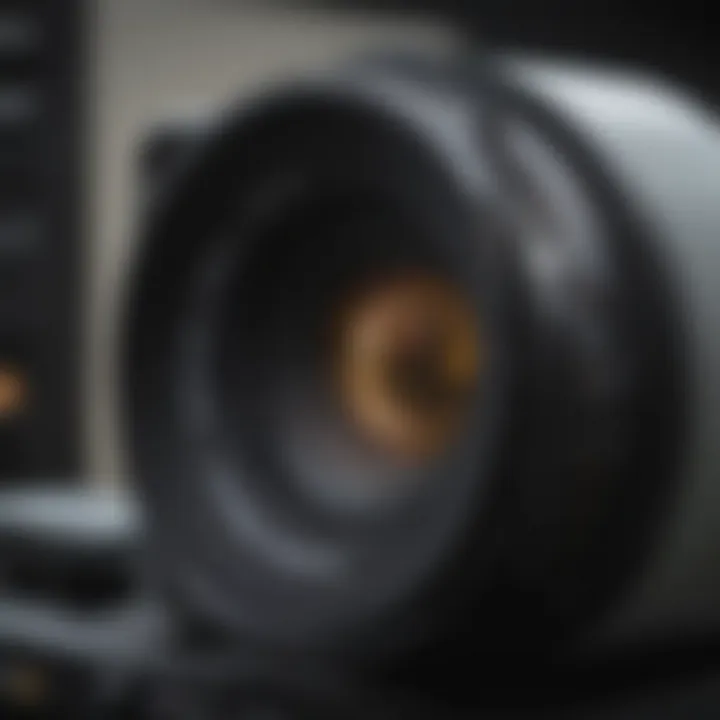
- Identify characteristic peaks that correlate with known functional groups. For instance, a strong peak around 1700 cm⁻¹ may indicate a carbonyl group.
- Observe peak intensities to obtain qualitative data on concentrations of components.
- Utilize software tools for advanced analysis, employing algorithms to assist in peak assignment and quantification.
It's essential to consider baseline correction and derivative spectroscopy techniques to enhance peak visibility and clarity. These methods help eliminate noise, which can obscure critical spectral features.
Common Challenges in Data Collection
Challenges in data collection can significantly affect the quality of FTIR data. Awareness of these challenges is vital for successful analysis.
Some common issues include:
- Sample Preparation: Poorly prepared samples can lead to anomalous spectral features. Ensuring samples are clean and correctly formatted is essential.
- Atmospheric Interference: Water vapor and CO₂ can create overlapping spectral features leading to misinterpretation. Conducting experiments in controlled environments can mitigate this problem.
- Instrument Calibration: Inadequate calibration can result in systematic errors. Regular maintenance of instruments is crucial.
Understanding these aspects of data acquisition helps to refine methods and improve reliability in FTIR analysis. By addressing these challenges, researchers can enhance spectral quality and the information extracted from FTIR studies.
"Precision in data acquisition lays the foundation for impactful scientific discovery."
Through careful consideration of scanning techniques, proficient spectrum interpretation, and awareness of common data collection challenges, researchers can significantly improve their FTIR outcomes. In the context of ongoing advances in FTIR technology and methodologies, the importance of meticulous data acquisition cannot be overstated.
Data Analysis Methods
In the realm of FTIR characterization, the analysis of data is crucial for deriving meaningful conclusions about materials. The reliability of the results hinges on the effectiveness of the data analysis methods employed. As FTIR generates complex spectral data, careful interpretation and analysis are necessary to distinguish between various molecular constituents and their interactions. The ability to accurately analyze this data has profound implications in material science, chemistry, and other fields, where nuanced differences in molecular structures can dictate different properties or functionalities.
Software Tools for FTIR Data Analysis
The advancement of technology has led to the development of sophisticated software tools for FTIR data analysis, making it easier for researchers to process and interpret spectral data. Some of the widely used software in this area includes:
- OriginLab: Known for its data analysis and graphing capabilities.
- OMNIC: Specifically designed to handle FTIR data, offering features for spectral editing and analysis.
- Grams/AI: A comprehensive software package that supports various analytical functions for FTIR data interpretation.
These tools often provide functionalities such as baseline correction, peak assignment, and data smoothing. They can significantly reduce the time required for analysis and increase the accuracy of results. Thus, selecting the right software for specific needs is an essential consideration for effective data analysis.
Quantitative vs. Qualitative Analysis
Understanding the distinction between quantitative and qualitative analysis in FTIR is fundamental.
- Quantitative Analysis: This method focuses on measuring the concentration or amount of specific components in a sample. Here, techniques such as calibration curves and standard addition methods are commonly used. For quantitative analysis, accurate sample preparation and instrument calibration are vital.
- Qualitative Analysis: Conversely, qualitative analysis aims to identify the presence of specific compounds within a sample. It provides insights into molecular structure and functional groups without quantifying their amounts. FTIR is particularly strong in qualitative analysis due to its ability to reveal distinct vibrational signatures associated with different molecular bonds.
In practice, both methods complement each other and can be used together to provide a comprehensive understanding of the sample being studied.
Statistical Methods in Spectral Interpretation
Statistical methods play a critical role in the interpretation of FTIR spectra, particularly when distinguishing subtle differences in spectral data or when dealing with complex mixtures. Common statistical techniques used include:
- Principal Component Analysis (PCA): This method helps reduce the dimensionality of data while retaining trends and patterns, making it easier to visualize complex datasets.
- Cluster Analysis: Useful for classifying spectra into groups based on similarities, aiding in the identification of patterns or anomalies.
- Regression Analysis: Employed to model relationships between variables, allowing researchers to predict outcomes based on similar spectra.
By incorporating these statistical methods into FTIR data analysis, researchers can increase confidence in their interpretations and reduce the chances of errors that arise from subjective judgment.
The integration of advanced statistical methods in FTIR analysis allows for enhanced reproducibility and reliability of results, which is essential in scientific research.
Applications of FTIR in Various Fields
Fourier-transform infrared spectroscopy (FTIR) has emerged as a crucial analytical method across diverse scientific disciplines. Its versatility allows scientists to understand complex molecular structures and their interactions with great accuracy. In this section, we will delve into several key areas of FTIR applications, shedding light on the specific benefits and considerations involved in each field.
FTIR in Material Science
In material science, FTIR plays a vital role in characterizing polymers, composites, and nanomaterials. It provides insights into the molecular bonds and functional groups present in materials, which are essential for applications ranging from the development of new materials to quality control. Using FTIR, researchers can identify changes in the chemical structure during processing or degradation, which can inform decisions on material selection and performance.
One benefit of FTIR in material science is its ability to analyze solid, liquid, or gaseous samples without extensive preparation. This flexibility accelerates research timelines and enhances data collection efficiency. Additionally, FTIR can detect minute quantities of contaminants, crucial for ensuring the purity and quality of materials.
FTIR in Pharmaceutical Analysis
The pharmaceutical industry heavily relies on FTIR for drug characterization, formulation analysis, and quality assurance. FTIR assists in identifying active pharmaceutical ingredients (APIs) and excipients, as well as monitoring changes in chemical structure. This technique can determine polymorphic forms, which significantly affect the drug's efficacy and stability.
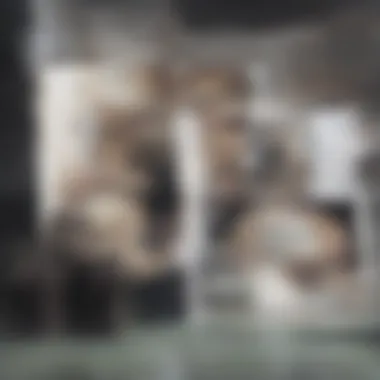
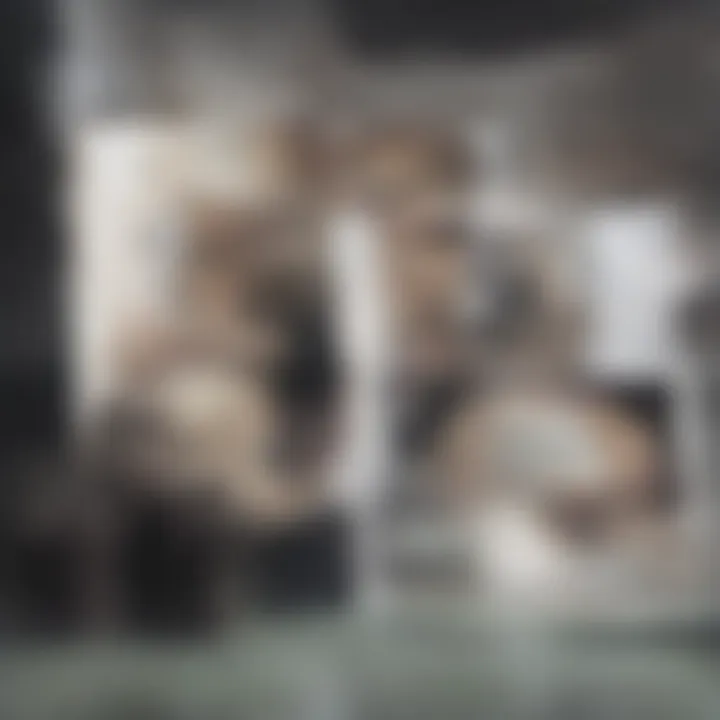
FTIR’s non-destructive nature allows the analysis of solid dosage forms, making it an attractive tool for ongoing research and development. Furthermore, it can aid in the validation of manufacturing processes, ensuring that products meet stringent regulatory standards. Accurate and reliable data from FTIR can thus streamline development cycles and reduce costs associated with pharmaceutical products.
FTIR in Environmental Studies
In environmental studies, FTIR provides essential information about atmospheric gases, pollutants, and various environmental samples. It can monitor air quality by detecting gases such as carbon dioxide, methane, and volatile organic compounds. This monitoring is critical for assessing environmental changes and aiding in compliance with regulatory standards.
FTIR also analyzes soil and water samples, allowing researchers to evaluate contamination levels and understand the presence of hazardous substances. By accurately characterizing organic and inorganic compounds, FTIR supports environmental remediation efforts and resource management, while helping to address widespread ecological concerns.
FTIR in Forensic Science
In forensic science, FTIR has become a fundamental tool for analyzing substances found at crime scenes. Its application involves the identification of unknown materials such as drugs, explosives, and fibers. The ability of FTIR to provide rapid and reliable analysis offers invaluable assistance to forensic investigators.
Each forensic examination begins with sample collection. After this, FTIR can reveal significant characteristics of the material under investigation. The data generated can assist in associating suspects with evidence, thereby supporting legal cases. Its non-destructive feature is particularly beneficial, as it preserves samples for potential further analysis.
FTIR is a versatile technique that has broad applications in various scientific domains. Its ability to analyze different types of samples and provide reliable data makes it indispensable in modern research and analysis.
Emerging Trends and Future Directions in FTIR
FTIR spectroscopy continues to evolve, unveiling new possibilities in scientific research and industrial applications. Understanding these emerging trends is critical. This section will outline advancements that push the boundaries of what FTIR technology can achieve, highlighting the benefits these introduce to the field.
Advancements in FTIR Technology
Recent years have seen significant advancements in FTIR technology. One of the most notable developments is the miniaturization of FTIR instruments. Compact devices are now available, allowing for in-situ analysis in field settings. This convenience opens possibilities in areas where traditional laboratory setups are impractical.
Additionally, the introduction of portable FTIR spectrometers is revolutionizing on-site testing. These devices offer rapid analysis, facilitating immediate decision-making in various fields, such as quality control in manufacturing.
Also, the integration of FTIR with advanced detection techniques, such as imaging and hyperspectral analysis, expands the analytical capabilities. The combination leads to enhanced spectral data collection and more detailed information regarding sample composition and behavior. This integration can also refine qualitative and quantitative analysis.
Integration with Other Analytical Techniques
The future of FTIR lies in its integration with other analytical techniques. Combining FTIR with techniques like Raman spectroscopy and mass spectrometry creates a multi-dimensional view of samples. Each technique brings unique advantages, enabling more comprehensive data interpretation.
For instance, while FTIR excels in providing information about molecular vibrations, Raman adds information about molecular symmetry and can be sensitive to different vibrational modes. This complementary nature significantly enhances analytical depth, allowing researchers to converge findings from different perspectives.
Collaboration between various methods can lead to breakthroughs in chemical analysis. It supports accurate substance identification, leading to better formulation in pharmaceuticals and deeper insights in material science.
Potential in Biotechnology and Nanotechnology
FTIR spectroscopy shows considerable promise in biotechnology and nanotechnology. As these sectors expand, FTIR applications are likely to become more prominent.
In biotechnology, FTIR can be utilized to study biomolecular interactions at the surface level. This can facilitate drug discovery processes through detailed analyses of drug-receptor interactions, potentially speeding up the development of new therapeutics.
In nanotechnology, FTIR is powerful for characterizing nanomaterials. Specific vibrational modes can provide insights into the structure and function of nanoparticles. This understanding is valuable, from improving drug delivery systems to developing new materials with unique properties.
As these fields evolve, FTIR remains an integral tool, adapting to and enhancing the future of research and application.
The End
Summary of FTIR Importance
FTIR is integral to various scientific fields, including chemistry, materials science, and biology. The technique's ability to provide precise molecular information enhances our comprehension of complex materials. Notably, FTIR offers:
- Non-destructive analysis, allowing for the preservation of samples while gathering vital data.
- Rapid data acquisition, enabling timely decision-making in research and development.
- Flexibility in sample types, accommodating solids, liquids, and gases.
These advantages underscore FTIR's position as an invaluable tool in laboratories globally. By facilitating a deeper understanding of molecular interactions, FTIR contributes significantly to advancements in both academic and industrial settings.
Lasting Impact on Scientific Research
The influence of FTIR extends far beyond immediate applications. Its ability to provide molecular insights has profound implications for future scientific research. Key impacts include:
- Facilitation of innovative discoveries: FTIR aids in identifying unknown substances, which can lead to breakthroughs in pharmaceuticals and materials discovery.
- Enhancement of interdisciplinary research: The integration of FTIR with other analytical techniques allows for comprehensive studies, fostering collaboration across fields.
- Contribution to sustainable practices: In environmental studies, FTIR helps in monitoring pollutants, thereby contributing to better environmental management.
Moreover, the ongoing advancements in FTIR technology, such as the development of portable FTIR spectrometers, suggests that its applications will continue to grow. This will likely lead to new methodologies and insights that can reshape how we approach scientific challenges in the years to come.
As a tool that bridges gaps within various scientific disciplines, FTIR will undoubtedly leave a lasting legacy in research, innovation, and application. Its ability to allow for in-depth understanding of materials continues to drive forward the boundaries of scientific knowledge. [Source](https://en.wikipedia.org)
"Fourier-transform infrared spectroscopy is not just a technique; it is a gateway to understanding the very fabric of matter."
The impact of FTIR on scientific research will continue to evolve, proving essential for tackling the complexities of modern scientific questions.