Examining mRNA: Its Role and Importance in Biology
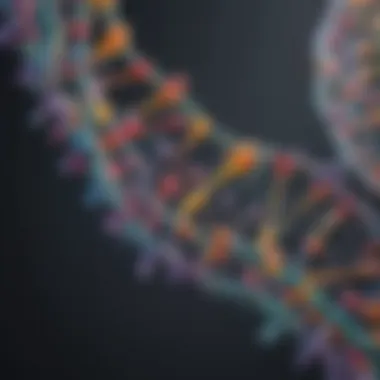
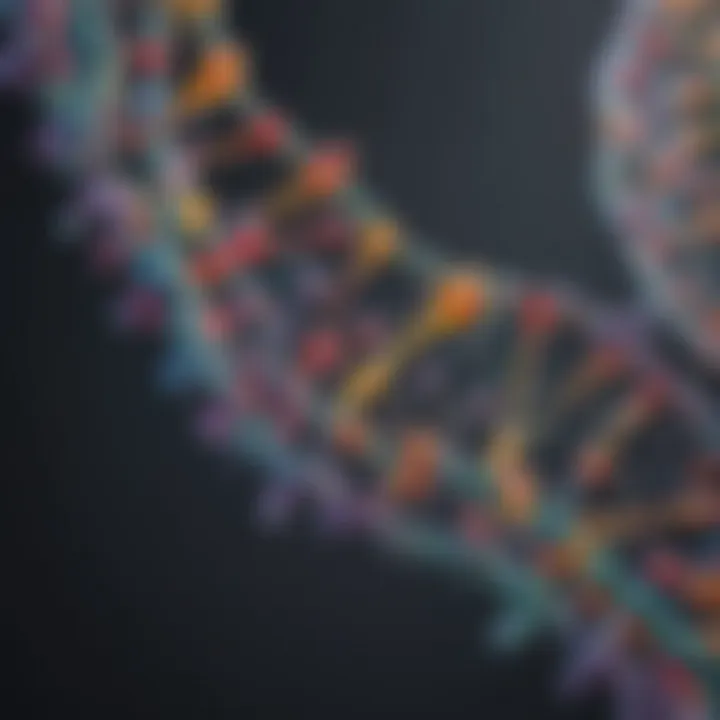
Intro
Messenger RNA (mRNA) plays a pivotal role in the realm of cellular biology. It serves as the intermediary that conveys genetic instructions from DNA to the ribosomes, where proteins are synthesized. This molecule is not just a simple messenger; its intricate structure and function are fundamental to how life operates at a cellular level. The recent advancements in biotechnology, especially in the development of vaccines, have underscored the importance of understanding mRNA further.
As we dig deeper into the subject, we will dissect the nuances of mRNA, exploring its structural anatomy, the processes that govern its functionality, and the broader implications it has in medicine and research. By gaining insight into mRNA's role, we can appreciate how this molecule is reshaping our understanding of biology and opening doors to innovative therapeutic strategies.
Understanding mRNA
Understanding mRNA is pivotal for grasping the intricate workings of cellular biology. Messenger RNA, or mRNA, plays a crucial role as a molecular intermediary, translating the genetic code from DNA into functional proteins. This step is fundamental in the development and functioning of all living organisms, making the study of mRNA essential in various fields, including genetics, molecular biology, and biomedicine.
A solid grasp of mRNA's roles and characteristics lays the groundwork for comprehending its implications in biotechnological advancements such as vaccine development and gene therapy. With the recent surge in mRNA applications, especially illustrated by the swift creation of vaccines during the pandemic, delving deep into its science has never been more pertinent.
Definition of mRNA
Messenger RNA, abbreviated as mRNA, serves as a critical component in the process of protein synthesis. It is a single-stranded molecule synthesized from DNA, carrying genetic instructions from the nucleus to the ribosomes, where proteins are assembled. The sequence of nucleotides in mRNA dictates the sequence of amino acids in the resulting protein, linking the genetic code to functional cellular machinery.
Historical Context
The journey of mRNA began in the early 1960s with the groundbreaking discoveries of researchers such as François Jacob and Jacques Monod, who explored gene regulation in bacteria. Their work laid the foundation for the understanding of how mRNA regulates protein synthesis. By the 1970s, advances in molecular biology allowed scientists to isolate and study mRNA in greater detail, revealing its structure, function, and significant role in gene expression.
Throughout the decades, research has unveiled the complexities of mRNA, highlighting its importance not only in fundamental biology but also in applied sciences, leading to developments in therapeutic strategies.
Chemical Composition
Understanding the chemical composition of mRNA provides insights into its structure and function.
RNA Structure
The structure of RNA differs from DNA primarily in that RNA is usually single-stranded, which allows for greater flexibility and a wider range of molecular interactions. Within the structure of mRNA, there are specific regions, including the 5' cap and the poly-A tail, that contribute to its stability and export from the nucleus. The key characteristic of mRNA's structure is its ability to fold into various shapes, which can influence its interaction with other molecules. This characteristic is beneficial for cellular processes such as translation and regulation of gene expression. An noteworthy feature of mRNA structure is the presence of non-canonical base pairing, which can introduce additional conformational diversity and functional implications in cellular mechanisms.
Nucleotide Building Blocks
Nucleotide building blocks are the foundation of mRNA, comprising four fundamental units: adenine (A), uracil (U), cytosine (C), and guanine (G). The arrangement of these nucleotides encodes the information necessary for protein synthesis. A pivotal characteristic of nucleotide building blocks is that they enable rapid synthesis and degradation of mRNA, thus allowing cells to quickly respond to changes in environmental conditions. The significant feature in this context is that the variability in sequences can lead to diverse functional outcomes, reflecting the adaptability and versatility of cellular processes.
Comparisons with DNA
When comparing mRNA with DNA, it becomes evident that while both molecules are vital for genetic processes, they follow distinct paths in cellular function. DNA serves as the long-term storage of genetic information in a double-helix form, whereas mRNA is derived from DNA and serves as the transient messenger that conveys this information to protein synthesis machinery. One key distinction lies in their stability; RNA is generally less stable than DNA, which allows for a finely tuned regulation of when and how much protein is made. This difference is significant, as mRNA's short lifespan is advantageous in allowing organisms to adapt quickly to changing demands, particularly during cellular responses to stress or growth conditions.
Understanding mRNA is key to unlocking the secrets behind cellular function and its applications in biotechnology and medicine.
This comprehensive approach to mRNA underscores its complexity and uniqueness, revealing insights that enrich our understanding of molecular biology and its applications in health and disease.
The Role of mRNA in Cells
The significance of messenger RNA (mRNA) within cells cannot be overstated. This molecule acts as a critical intermediary in the process of protein synthesis, bridging the genetic instructions encoded in DNA with the cellular machinery that translates these instructions into functional proteins. As the cornerstone of gene expression, mRNA carries the essential genetic information that guides numerous cellular activities. Understanding this role unveils the dynamic processes that drive life at the cellular level.
Transcription Process
Transcription is the initial step in gene expression, where mRNA is synthesized from a DNA template. This process unfolds in three key phases: initiation, elongation, and termination.
Initiation Phase
The initiation phase marks the starting point of transcription. RNA polymerase, the enzyme responsible for synthesizing RNA, binds to a specific region on the DNA known as the promoter. This binding is akin to finding a marker on a treasure map; without it, the entire transcription process would stall. One notable aspect of this phase is the involvement of transcription factors, which assist RNA polymerase in locating the promoter accurately. This collaboration is essential, as incorrect initiation can lead to dysfunctional mRNA, potentially resulting in faulty proteins. The efficiency of this phase sets the tone for the rest of the transcription process, laying a solid foundation for mRNA production.
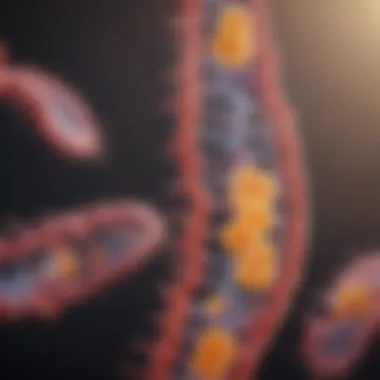
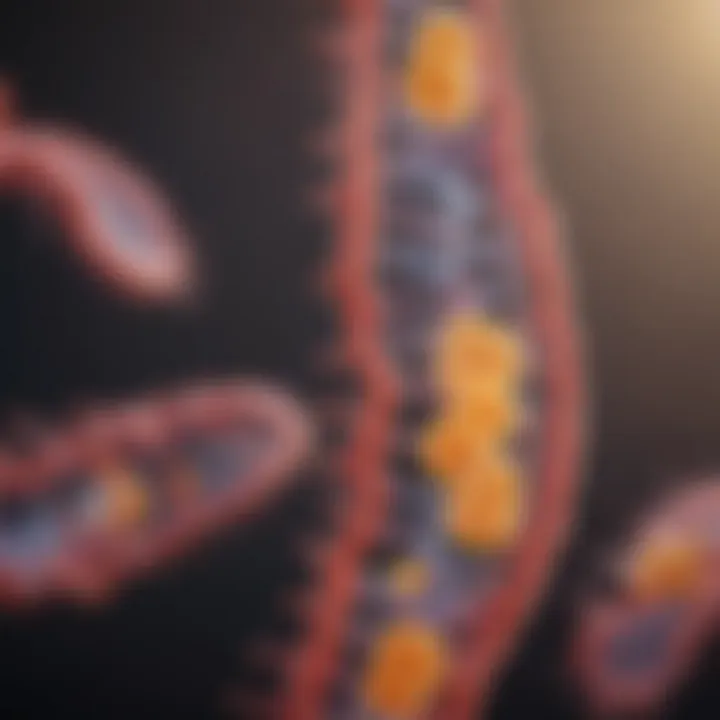
Elongation Phase
Following initiation, the elongation phase begins. Here, RNA polymerase moves along the DNA strand, unwinding the double helix as it synthesizes a complementary RNA strand. This phase is characterized by the rapid addition of RNA nucleotides, resulting in an mRNA transcript that elongates as the synthesis progresses. A key feature of this phase is that the newly formed mRNA strand undergoes co-transcriptional modifications, such as the addition of a 5' cap and poly-A tail. These modifications are beneficial, providing stability to the mRNA and facilitating its export from the nucleus to the cytoplasm. However, elongation must be tightly regulated; excessive or insufficient synthesis can have dire consequences for cell function.
Termination Phase
The termination phase concludes the transcription cycle. It occurs when RNA polymerase encounters specific sequences on the DNA that signal the end of the gene. Upon reaching these signals, RNA polymerase detaches from the DNA, releasing the newly synthesized mRNA strand. This process also involves the cleavage of the pre-mRNA to form the mature mRNA ready for translation. A unique aspect of termination is the regulation of what happens post-synthesis, including splicing events that can generate different protein variants from a single gene. Thus, while termination might seem like a straightforward end, it marks the beginning of diverse protein expressions crucial for cellular functions.
Translation Mechanism
Once mRNA is synthesized, it undergoes translation—a multi-step process wherein ribosomes convert mRNA sequences into proteins. The translation mechanism consists of several components and phases, each playing an integral role in ensuring proper protein assembly.
Ribosome Functionality
Ribosomes are the cellular machines that carry out the translation of mRNA into protein. Comprised of ribosomal RNA and proteins, these complexes facilitate the decoding of mRNA sequence into a chain of amino acids. A critical characteristic of ribosomes is their dual subunit composition—large and small subunits—that ensures effective interaction with mRNA and transfer RNA (tRNA) during translation. This feature makes ribosomes essential for maintaining accuracy and efficiency in protein synthesis. While ribosomes are incredibly versatile, they too face challenges; errors in translation can lead to nonfunctional or harmful proteins, highlighting the need for fidelity in this process.
tRNA Role
Transfer RNA (tRNA) serves as the translator between the mRNA sequence and the resulting amino acid chain. Each tRNA molecule carries a specific amino acid and possesses an anticodon that matches a corresponding codon on the mRNA. This ensures that the correct amino acids are added in the appropriate order. The unique capacity of tRNA to read mRNA codons and deliver matched amino acids makes it a pivotal player in translation. One of its distinctive characteristics is the ability to undergo charging, where it becomes covalently bonded to its respective amino acid, preparing it for incorporation into the growing polypeptide chain. However, mischarging can lead to protein defects, underscoring the importance of accuracy.
Amino Acid Chain Formation
Amino acid chain formation is the culmination of the translation process, producing the primary structure of proteins. As ribosomes facilitate the assembly, peptide bonds form between adjacent amino acids, resulting in a polypeptide chain. A key characteristic of this phase is its reliance on the sequence of mRNA codons, which directly dictates the resulting amino acid sequence. This relationship is foundational for the final protein's functionality. A unique aspect is the folding process that occurs post-translation, as the newly formed chain begins to fold into its three-dimensional structure. However, improper folding can lead to misfunctional proteins, revealing the delicate balance required in protein synthesis.
Post-translational Modifications
Post-translational modifications are crucial for dictating protein functionality and stability. These changes can occur after the polypeptide is synthesized and can include phosphorylation, glycosylation, and methylation, among others. Such modifications influence not just the protein's activity, but also its localization within the cell. A key point to note is the diversity of modifications possible; some proteins may undergo multiple modifications, resulting in distinct functional forms. The advantage of post-translational modifications lies in their ability to regulate protein activity in response to cellular signals and environmental changes, providing cells with a fine-tuned method to adjust their responses to ever-shifting conditions.
mRNA Variants and Their Functional Implications
Understanding the variability in mRNA is paramount to grasping its diverse roles within cellular mechanics. As researchers explore mRNA, they find that not all messenger RNA is created equal. The variants serve distinct purposes and offer varying degrees of functionality within the cell.
Types of mRNA
Polyadenylated mRNA
Polyadenylated mRNA, often referred to as poly(A) mRNA, features a long chain of adenine nucleotides at its tail end. This tail is not just for decoration; it plays a crucial role in stability and the export of RNA from the nucleus. One key characteristic of poly(A) tails is their influence on translation efficiency, as they help protect mRNA from degradation. This mRNA variant is essentially popular due to its significant presence in the coding regions of eukaryotic genes.
The unique feature of polyadenylated mRNA lies in its contribution to both the life span and translational control of mRNA. It serves as a protective mechanism, which in turn boosts its potential for protein synthesis. However, when considering disadvantages, highly stable poly(A) tails could sometimes lead to prolonged expression of certain genes beyond their necessity, potentially affecting cellular regulations.
Non-coding RNA
Non-coding RNA (ncRNA) may sound like an afterthought, given its name, but don’t be fooled. This variant does not code for proteins, yet it is far from useless. Instead, it participates in regulating gene expression. One key aspect of ncRNA is its involvement in gene silencing and chromatin remodeling, which highlights its significance in the overall functioning of the genome.
The charm of non-coding RNA lies in its regulatory capacities. Unlike other types of mRNA, ncRNA can control various cellular processes, tailoring the activity of coding genes. Its downside? Sometimes, it can complicate interpretations of how genes interact and regulate each other, making it a double-edged sword in research.
Small Interfering RNA
Small interfering RNA, often abbreviated as siRNA, represents another fascinating type of mRNA variant. SiRNA plays a pivotal role in the RNA interference (RNAi) pathway, where it helps in the silencing of specific genes by promoting their degradation. This characteristic makes siRNA immensely powerful in gene regulation, potentially serving as an effective tool in experimental and therapeutic settings.
A unique feature of siRNA is its ability to specifically target and inhibit the expression of genes, which grants researchers the ability to fine-tune biological responses. However, one consideration is the potential for off-target effects, where siRNA unintentionally silences other genes, complicating the outcomes of genetic experiments.
Alternative Splicing

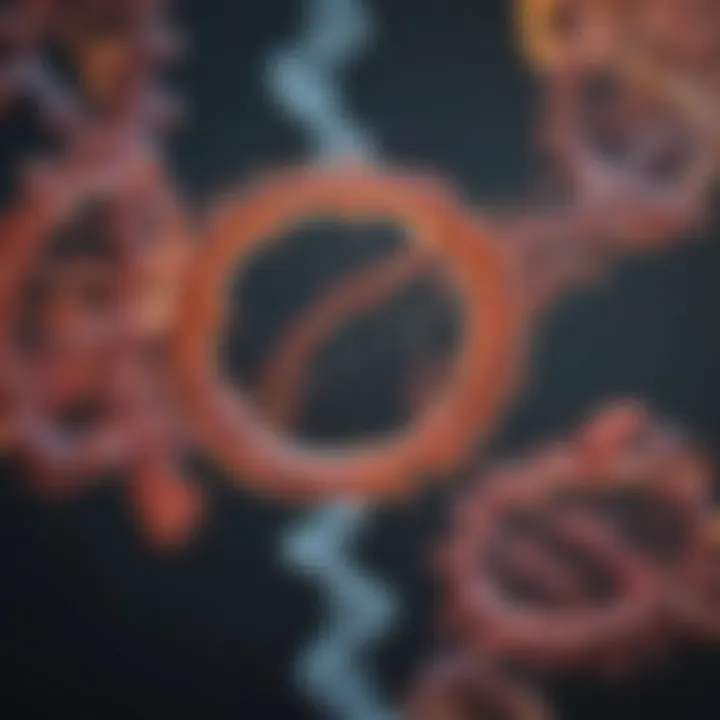
The phenomenon of alternative splicing is a game changer in how mRNA functions. This process allows a single gene to produce multiple mRNA variants by including or excluding certain segments. It is like a blueprint that can be modified to create different structures, leading to diverse protein products from a single genetic code. This flexibility significantly contributes to the complexity of proteomes in multicellular organisms, allowing for the customization of protein functions. It also supports cellular adaptation to varying environmental contexts.
mRNA Stability and Degradation
The stability of mRNA is crucial for determining how long the message stays available for translation into protein. Some variants are designed for longevity, while others are tagged for rapid degradation. This turnover rate can be influenced by various factors, including sequences in the mRNA itself and interactions with regulatory proteins. Understanding mRNA stability helps clarify not only how proteins are synthesized but also how their production is finely controlled, which is vital in numerous biological processes.
Biotechnological Applications of mRNA
Biotechnology is now a major driver of mRNA research, opening doors that were previously shut tight. With the rise of worlds like genetic engineering and vaccine development, mRNA has cemented itself as a crucial player. Understanding its applications can shine a light on how it can impact medicine and beyond. This discovery is not just a flash in the pan; its benefits stretch across various fields, addressing real-world problems.
mRNA Vaccines
Mechanism of Action
The mechanism of action for mRNA vaccines is a game changer in modern medicine. Rather than using live pathogens, mRNA vaccines harness the body's own machinery for a safer approach. When injected, the mRNA instructs human cells to produce a harmless piece of the viral protein. This ignites an immune response, enabling the body to recognize and combat the actual virus if ever encountered.
- A key characteristic of this method is its speed; mRNA can be synthesized quickly, facilitating rapid vaccine development in crises.
- A notable advantage to consider is that it can be adapted swiftly with the emergence of new variants. However, some may find the concept daunting, as it feels novel and different compared to traditional vaccines.
Success in Pandemic Response
The success of mRNA vaccines during the COVID-19 pandemic has set a new pinnacle in the history of vaccine technologies. They have demonstrated not only efficacy but also the ability to meet urgent health needs quickly. This allowed public health measures to be stepped up significantly.
- The key characteristic here is the impressive speed of development, with how fast these vaccines reached the public, showing a level of urgency unprecedented till now.
- A unique feature is the adaptability against mutations, exemplified in the case of Delta and Omicron variants, which posed new challenges. While the benefits are clear, there are disadvantages such as the need for cold storage which can complicate distribution.
Future Prospects
The future of mRNA technology is rife with potential. Researchers are continuously exploring not just the use in vaccines, but for cancer treatment, autoimmune diseases, and even metabolic disorders. Its applications could be vast and varied.
- A key characteristic is the ongoing research indicating that mRNA could pave the way for personalized medicine, targeting therapies to an individual's unique genetic makeup.
- The unique feature of mRNA being programmable allows for a broad spectrum of uses, but challenges remain. Questions about long-term safety and public acceptance must be addressed thoroughly.
Gene Therapy Applications
Gene therapy utilizing mRNA is an exciting frontier. By delivering specific RNA sequences directly to patients, mRNA holds the promise of correcting genetic defects and treating diseases at their source. This innovation echoes the traditional principles of medicine—target and treat—but with a modern twist that speaks to precision.
- mRNA gene therapy can potentially replace missing or dysfunctional proteins that lead to specific conditions.
- With low immunogenicity, it minimizes the risk of adverse reactions while maximizing therapeutic efficacy.
Synthetic mRNA Development
Manufacturing Processes
The manufacturing processes for synthetic mRNA have matured over the years, and the complexity has escalated. Using in vitro transcription, it’s possible to create mRNA that can potentially serve in a myriad of applications from vaccines to treatments.
- A key characteristic of these processes is scalability. Companies can ramp up production to meet global demand in a remarkably short time frame.
- Furthermore, achieving high purity and yield has become more feasible with advancements in technology, solidifying mRNA as a reliable candidate for therapeutic use.
Regulatory Challenges
Regulatory challenges pose a significant hurdle for synthetic mRNA development. Safety and efficacy standards are stringent, and navigating the maze of regulations can be daunting.
- The key characteristic here is that regulatory bodies must ensure that new mRNA treatments meet high standards before they reach the public. This inherently slows down the process.
- The unique feature of this challenge lies in balancing innovation with safety, which is a delicate dance that requires constant dialogue between developers and regulators.
Challenges and Ethical Considerations
As mRNA technologies continue to shape medicine and research, understanding the challenges and ethical implications becomes crucial. These factors not only influence scientific progress but also inform public dialogue and regulatory frameworks. The stakes are high; addressing these concerns fosters trust in scientific advancements and promotes responsible innovation.

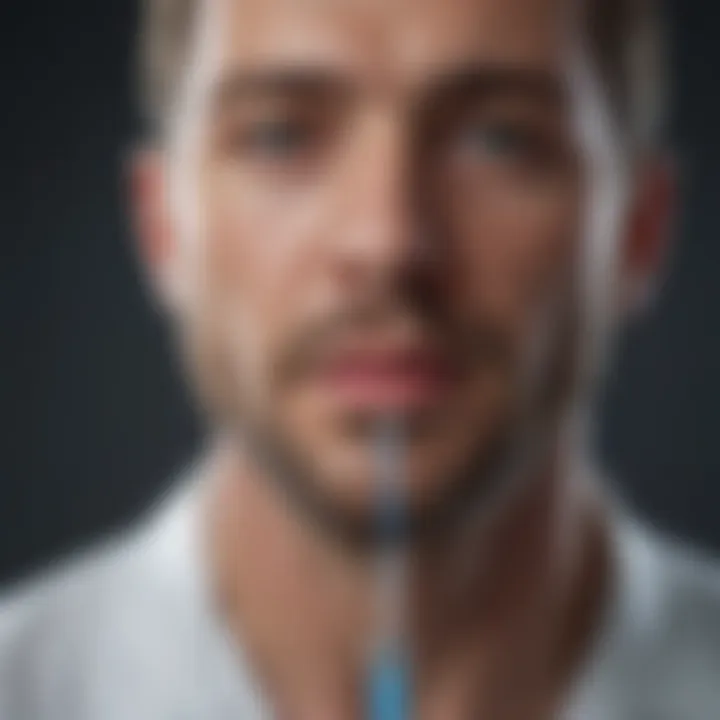
Safety and Efficacy Concerns
Safety and efficacy are at the forefront of discussions surrounding mRNA applications. It's vital to ensure that any new therapies or vaccines not only work effectively but do so without causing harm. For instance, mRNA vaccines like Pfizer-BioNTech and Moderna have undergone rigorous clinical trials to assess their impacts. Data from these trials guide health officials in decision-making, shaping public health policies worldwide. The speed at which mRNA platforms developed for COVID-19 raised questions for many—was safety compromised for the sake of urgency?
The balance between rapid development and thorough evaluation is a tightrope act. Patients and healthcare professionals need assurance that the benefits outweigh the inherent risks involved. In summation, ongoing monitoring of side effects and feedback loops between research teams and regulatory bodies are essential pathways toward ensuring that safety remains paramount in mRNA research.
Ethical Implications in Research
Consent and Regulation
In the realm of mRNA research, the aspect of consent and regulation cannot be understated. Potential participants in clinical trials must be fully informed about what they’re participating in. This includes understanding not just the potential benefits, but also the risks involved. A notable characteristic of this ethical framework is that it enables agency, allowing individuals to make informed choices regarding their own health.
However, the regulatory landscape can be a complicated maze. It evolves constantly, striving to keep pace with scientific advancements. The nimbleness of regulatory bodies is tested as new mRNA uses emerge. Striking a balance between protecting public interests and fostering innovation is a significant hurdle.
This duality highlights a unique feature of consent: it empowers individuals while also placing the onus on researchers and companies to operate transparently. Hence, it is vital for mRNA-related initiatives to prioritize ethical transparency, ensuring that informed consent is not just a formality but a core principle.
Long-term Effects
The long-term effects of mRNA technologies, especially in vaccine development, are another area of ethical concern. While short-term outcomes can be tracked and analyzed, the implications that stretch over months or even years post-administration merit focused scrutiny. A powerful characteristic of this aspect lies in its predictive nature. The scientific community is tasked with assessing how the body's immune response and other physiological reactions evolve over time.
Unique in this case is the aspect of cautious optimism; scientific advancements can bring monumental benefits, but there’s always a shadow of uncertainty. Potential unexpected long-term effects could alter public confidence in mRNA technologies.
Regular follow-up studies and validation of empirical data are essential to illuminate these unknowns. The navigational road ahead necessitates not just evidence-based insights but also a compassionate approach to public health communications, ensuring that the populace remains informed while maintaining trust in science.
Public Perception of mRNA Technologies
Public perception of mRNA technologies often mirrors societal sentiments toward medical advancement. Factors like misinformation, Ssocial media discourse, and historical distrust in medical research can heavily shape opinions. Understanding how the public views these innovations is paramount for scientists and communicators alike.
Many people are still unaware of what mRNA is capably doing. With the COVID-19 pandemic thrusting it into the limelight, there was both excitement and apprehension.
- Misinformation can greatly sway public safety beliefs. Clearing misconceptions through transparent communication is thus vital.
- Trust in health authorities often influences acceptance. When people feel informed and involved, they are more likely to embrace new therapies, including mRNA.
Future Directions in mRNA Research
The ever-evolving field of mRNA research carries immense promise, a beacon of hope for numerous applications in biotechnology and medicine. This segment aims to navigate the intricate landscape shaping future endeavors while shedding light on pivotal considerations that emerge as the technology matures. These directions not only underscore the invaluable role that mRNA plays today but also paint a clearer picture of its potential impact on individual health and global healthcare solutions.
Innovations in mRNA Technology
Recent advancements in mRNA technology have sparked a wave of enthusiasm across scientific circles, ushering in an era characterized by unprecedented innovations. One key area of development lies in the optimization of mRNA sequences, enhancing their stability and translation efficiency in the cell. This optimization can lead to more effective therapeutics, opening doors for treatments that were previously deemed unfeasible.
Moreover, next-generation mRNA delivery systems are being formulated, employing lipid nanoparticles and other delivery mechanisms. Such progress minimizes immune responses while ensuring that the mRNA reaches its target with pinpoint accuracy. By improving the specificity and efficacy of these systems, researchers aim to elevate mRNA therapies' therapeutic outcomes.
"The frontier of mRNA technology is laden with opportunities that extend well beyond vaccines, emphasizing its versatility in treating diseases like cancer and genetic disorders."
Another interesting trajectory is the development of mRNA-based therapies that extend into the realm of personalized medicine. Here, the emphasis is placed on tailoring therapies to individual genetic profiles, potentially revolutionizing treatment paradigms. The gradual incorporation of machine learning techniques to predict successful mRNA designs marks a significant milestone, promising a more efficient innovation process.
Personalized Medicine Approaches
The concept of personalized medicine has garnered significant traction, and mRNA technology embodies a critical component of its implementation. mRNA-based therapeutics enable customized responses to treatment based on individual patient characteristics, a shift from one-size-fits-all approaches.
In practice, oncologists might analyze a patient's tumor to identify specific mutations and subsequently design mRNA that codes for a protein capable of eliciting a targeted immune response. This method shifts the paradigm in cancer treatment, making therapies not only more effective but also with potentially fewer side effects due to the specificity of the treatment.
In addition to oncology, chronic diseases such as diabetes could benefit from personalized mRNA therapies. For example, researchers are exploring mRNA solutions to help patients regenerate insulin-producing cells, catering to the unique circumstances of each individual’s condition. This focus on personalized approaches could revolutionize how diseases are managed, enhancing patient outcomes across the board.
Utilizing bioinformatics, researchers can efficiently analyze vast datasets, tailoring mRNA vaccines and therapies to genetic predispositions or unique responses to treatments. Consequently, this continuous loop of data and iteration fosters a responsive scientific environment to meet patient needs not previously addressed.
Moving forward, continued interdisciplinary collaboration among scientists, clinicians, and bioethicists is essential to navigate the landscape of personalized mRNA approaches. Balancing innovation with ethical considerations will be vital as researchers push boundaries further into this promising domain.
The trajectory of mRNA research presents not just a glimpse into the future of medical science but also serves as a reminder of how far we have come in the understanding and application of biological systems. The ongoing investment in these areas signals a commitment to expanding our capabilities, ultimately aiming to improve human health on a global scale.