Understanding Cas9 mRNA Transfection in Gene Editing
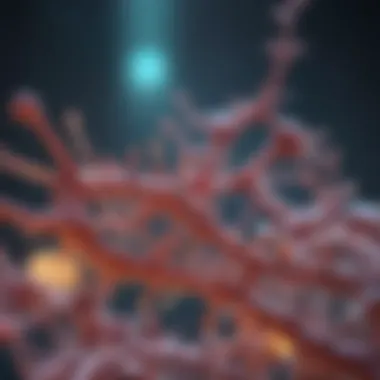
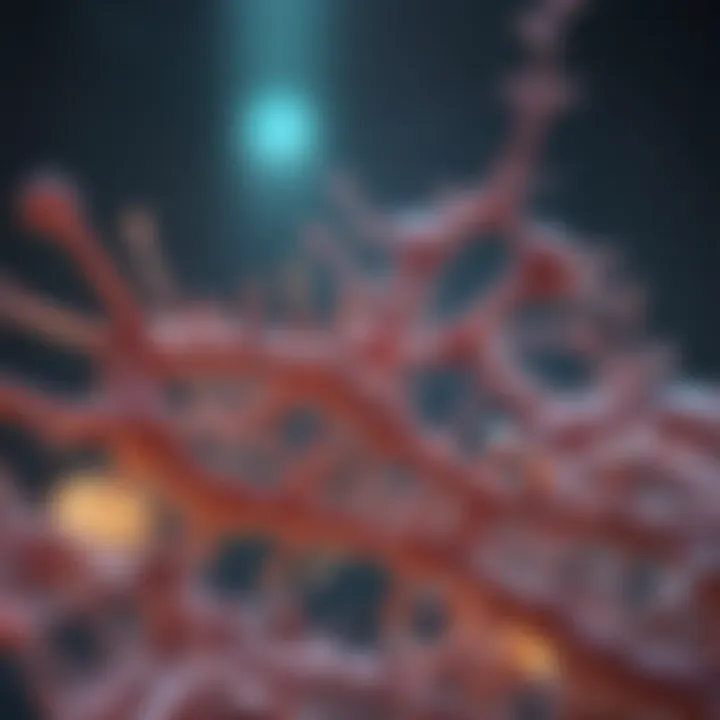
Intro
In the rapidly evolving landscape of genetic engineering, Cas9 mRNA transfection stands out as a cutting-edge methodology that has transformed our understanding and application of CRISPR technology. At its core, this process enables researchers to introduce the messenger RNA encoding the Cas9 nuclease into various cell types, facilitating precise genome editing. The significance of such precision cannot be overstated; with the potential to edit genes responsible for genetic disorders or enhance crop resilience, the ramifications echo across fields such as medicine, agriculture, and beyond.
Here, we will discuss the essential principles underlying Cas9 mRNA transfection, the techniques utilized for efficient delivery, and explore a variety of applications that stem from this powerful genetic tool. Throughout this discussion, we will highlight the importance of understanding these mechanisms, not only for those entrenched in genetic research but also for educators, students, and professionals aiming to stay abreast of contemporary advancements in biotechnology.
Prelims to Cas9 mRNA Transfection
The advent of CRISPR technology has revolutionized the field of genetic engineering, with Cas9 mRNA transfection standing at the forefront of this transformation. Understanding the mechanisms by which Cas9 operates, along with the role of mRNA, is crucial for grasping the full picture of gene editing capabilities today. This section aims to lay the groundwork for comprehending this significant topic by exploring the evolving methodologies and their respective benefits.
The utilization of Cas9 mRNA for transfecting target cells has several advantages. One primary benefit is the reduction in off-target effects. When using mRNA instead of DNA, there's a decrease in the likelihood of integration into the host genome, thus tempering potential risks associated with genomic alterations. Moreover, mRNA can be translated rapidly into protein, providing a quicker means of delivering the Cas9 enzyme directly to the cells. This swift action is vital in various applications, from therapeutic interventions to basic research in genomics.
Another consideration is the flexibility and adaptability that mRNA transfection offers researchers. Various delivery methods—be it chemical, physical, or viral—can be optimized to fit the specific requirements of the cells being studied or modified. Such versatility allows scientists to explore uncharted territories in gene therapy, cancer treatments, and agricultural biotechnology, thereby broadening the horizons of Cas9 applications.
With all these advantages, however, there still exist important challenges. For instance, the efficiency of mRNA delivery into cells can be variable, influenced by factors such as cell type, method of transfection, and the stability of the mRNA itself. Bearing all this in mind, it becomes evident that a comprehensive understanding of Cas9 mRNA transfection is essential not only for researchers but also for the broader implications it holds for biomedicine and agriculture.
Overview of Cas9 Technology
Cas9 technology, commonly associated with CRISPR-Cas9 systems, employs an RNA-guided endonuclease to modify genes at specific sites within an organism's genome. This system's origins trace back to bacterial immune mechanisms that protect against viral infections, showcasing how nature has paved the way for cutting-edge genetic engineering methodologies. The Cas9 protein can be guided to a target DNA sequence by a single guide RNA (sgRNA), which ensures precision in editing endeavors.
The ability to program Cas9 through the use of sgRNAs has unlocked possibilities that were once considered science fiction. Geneticists now possess the tools to add, modify, or delete DNA sequences with an unprecedented degree of accuracy which makes gene editing much simpler and more efficient.
Whether it’s conducting fundamental research or developing new therapies, the Core Cas9 technology serves as the backbone for a multitude of groundbreaking applications, one of which is mRNA transfection.
Significance of mRNA in Gene Editing
The role of mRNA in the context of gene editing cannot be overstated. Firstly, unlike DNA, mRNA does not integrate into the recipient genome, hence reducing risks of unwanted mutations and potential long-term effects that could arise from permanent modifications. This distinction is particularly significant in therapeutic applications where safety is paramount.
Using mRNA allows for a more transient expression of the Cas9 protein; it becomes critical when time-sensitive processes or tissue-specific modifications are desired. The transience means researchers can exert control over the levels and duration of protein expression, which is invaluable in studies that require tight regulation of gene activity.
Moreover, the use of mRNA circumvents the complexities associated with traditional plasmid DNA delivery. With increasing concerns regarding immunogenicity and toxicity associated with DNA vectors, mRNA serves as a more favorable alternative that has shown potential in multiple clinical trials.
In summary, as we delve deeper into the intricacies of Cas9 mRNA transfection, it’s clear that the integration of mRNA not only ameliorates safety concerns but also enhances versatility and efficacy in gene editing approaches. The intersection of these factors heralds significant advancements not just in research but in practical applications spanning therapeutic and agricultural innovations.
The Mechanism of Cas9 Action
The Cas9 protein is at the heart of CRISPR technology, acting like a pair of molecular scissors that can cut DNA at specific sites. Understanding the intricate mechanisms behind how Cas9 operates is essential for both researchers and practitioners aiming to harness this powerful tool for gene editing. The process relies on a well-orchestrated interaction between Cas9, guide RNA, and the target DNA, resulting in precise genetic modifications. As we venture into this section, we'll break down the structural intricacies, the pivotal role of guide RNA, how Cas9 recognizes and binds to its target, and the actual cleavage mechanism that brings gene editing to life.
Structure of Cas9 Protein
The Cas9 protein itself is a complex molecule, showcasing a two-lobed structure that is essential for its DNA-cutting abilities. Each lobe serves a distinct function, facilitating the recognition and binding of the target DNA sequence. The N-terminal lobe primarily houses the nuclease domains responsible for cleaving the DNA strands, while the C-terminal lobe mainly interacts with the guide RNA. The overall configuration allows Cas9 to effectively navigate within cells, aligning itself with the desired genetic targets.
Key characteristics of Cas9 structure include:
- Two distinct domains: Each lobe plays a critical part in the functionality, ensuring both specificity and efficiency.
- RNA-binding capability: This attachment area for guide RNA is crucial for the target identification process.
- Nuclease activity: The active sites within the protein execute the cutting of double-stranded DNA.
The structure of Cas9 not only underpins its function but also shapes the subsequent applications in gene editing.
Role of Guide RNA
At the crux of Cas9's functionality lies the guide RNA, a pivotal player in the CRISPR system. Comprising a short RNA sequence, the guide RNA is engineered to complement a specific DNA target. What makes this remarkable is its versatility—scientists can design guide RNA for almost any gene of interest, paving the way for targeted editing.
- Specific targeting: By matching the RNA sequence to a DNA locus, the guide RNA directs Cas9 precisely to the site of interest, ensuring high specificity in gene editing.
- Cation stability: The presence of guide RNA stabilizes the Cas9 protein in the cell, improving its longevity and performance.
- Flexible design: Researchers can exploit this capacity to tailor the guide RNA according to the needs of their specific applications, whether it’s in animals, plants, or microorganisms.
In sum, without the guide RNA, Cas9 would be a lost vessel, unable to find its way to the genomic sites it must alter.
Target DNA Recognition and Binding
Once the guide RNA is in place, the next stage involves target DNA recognition. Cas9 locates its specific DNA sequence via complementary base pairing between the guide RNA and the DNA. The recognition process is characterized by several key interactions:
- Seed sequence alignment: The initial matching occurs at a region known as the 'seed', where a few base pairs of the guide RNA bind to the target DNA, initiating the recognition process.
- Subsequent pairing: Following seed binding, Cas9 assesses additional base pairings along the length of the DNA sequence, ensuring the complete match required for effective binding.
- PAM sequence: A protospacer adjacent motif (PAM) is essential for Cas9 activity, providing the necessary docking site. Without it, no binding will occur. This requirement adds an additional layer of specificity to the process.
These intricate steps ensure that Cas9 precisely targets the correct region of DNA without significant off-target activity, a consideration that is crucial in achieving effective gene editing outcomes.
Cleavage Mechanism of Cas9
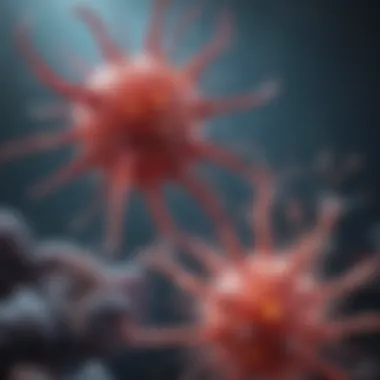
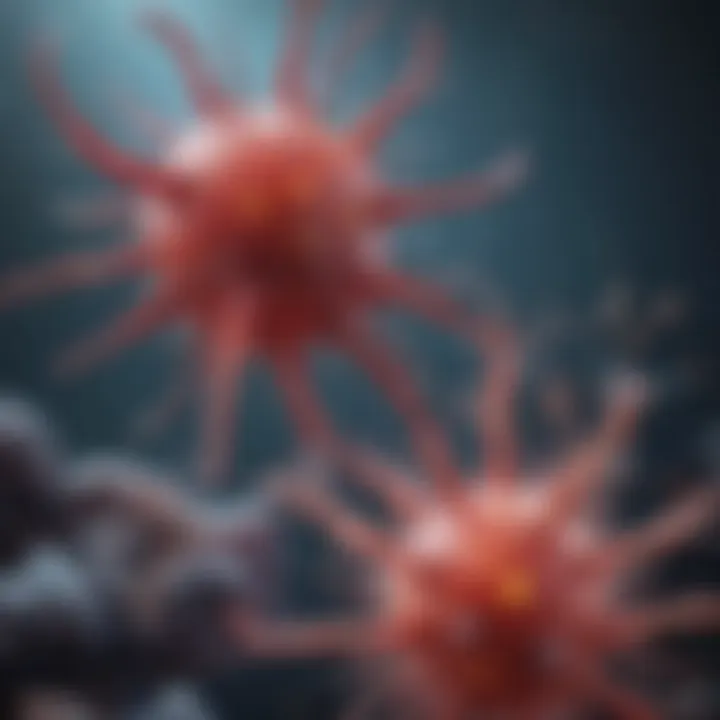
Finally, the actual cleavage of the DNA strand is executed through an elegant mechanism once the target is appropriately recognized and bound. The cutting action unfolds as follows:
- Nuclease activity: The binding of the active Cas9 protein induces a conformational change, activating its nuclease domains.
- Strand separation: Cas9 unwinds the double helix at the targeted location, providing access for the nuclease domains to act.
- Cleavage: The protein then cuts both strands of the DNA, creating a double-strand break. This break can be repaired by the cell through either non-homologous end joining or homology-directed repair, allowing for the insertion or deletion of genetic material.
"The elegance of the Cas9 mechanism lies in its ability to induce precise modifications that can lead to significant biological insights and potential therapeutic avenues."
This elucidation of the Cas9 action mechanism underscores not just its intricacies but also the broader implications it holds for advancements in genetic engineering and biotechnology.
Methods of mRNA Transfection
Transfecting mRNA into cells is a pivotal step in harnessing the power of CRISPR technology. The methods used to facilitate this transfection can significantly affect both the efficiency of gene editing and the ultimate success of any experimental or therapeutic application. This section will dissect the various methods available for mRNA transfection, focusing on their unique characteristics, benefits, and considerations that researchers must weigh carefully.
Physical Methods of Transfection
Electroporation
Electroporation stands out for its ability to introduce mRNA into a wide range of cell types effectively. This technique involves applying an electrical field to temporarily permeabilize cell membranes, allowing molecules like mRNA to enter. One of its key characteristics is that it can achieve high transfection efficiencies without requiring any helper substances.
The uniqueness of electroporation lies in its ability to transiently disrupt the cell membrane with precision. Its advantages include versatility with various cell types and the capacity for a large volume of mRNA to be delivered at once. However, one must also consider potential drawbacks, such as cellular damage or stress—issues that necessitate optimization parameters to minimize impact on cell viability.
Microinjection
Microinjection, on the other hand, is like the old-school artisan approach to transfection. It involves using glass micropipettes to inject mRNA directly into the cytoplasm of target cells. This method is especially beneficial when working with sensitive cells, as it allows for direct delivery without inducing generalized membrane permeability.
A distinct advantage of microinjection is its precision. Scientists can target individual cells and introduce precise quantities of mRNA. Yet, it’s not without its challenges; the technique can be labor-intensive, requiring considerable skill and fine motor control. Additionally, it is generally only practical for a small number of cells at a time, which limits throughput.
Chemical Methods of Transfection
Calcium Phosphate
One of the classical methods in the transfection toolkit, calcium phosphate transfection utilizes a simple yet clever mechanism. Researchers mix calcium ions with phosphate, forming precipitate that can encapsulate mRNA, which is then taken up by cells through endocytosis. Its ease and low cost make it a staple in many labs.
The appeal of calcium phosphate transfection lies in its simplicity and effectiveness for many cell lines. It can lead to high levels of expression but requires careful handling; the conditions must be just right. Factors like pH and calcium concentration are crucial, and a less-than-ideal setup can lead to poor results.
Lipid-Based Reagents
Then we have lipid-based reagents, which have grown in popularity due to their effectiveness and relative ease of use. These reagents form lipid nanoparticles that encapsulate and protect the mRNA. This process facilitates cellular uptake through fusion with the lipid bilayer of the cell membrane.
The key characteristic of lipid-based transfection is its ability to efficiently package mRNA while also enhancing cellular uptake. They are particularly favored for their versatility in different cell types and their ability to generate consistent results. Nonetheless, researchers must be cautious, as some reagents can introduce toxicity or result in variable expression levels depending on the cell type.
Viral Methods for Delivery
Using Lentiviral Vectors
Lentiviral vectors present a unique approach to mRNA transfection by utilizing viruses to carry genetic material into cells. These vectors can integrate the mRNA into the host genome, allowing for stable expression over time. One significant characteristic of lentiviral vectors is their ability to infect both dividing and non-dividing cells, making them particularly valuable for therapeutic applications.
The most compelling feature of employing lentiviral vectors is their long-term expression capability, ideal for applications requiring sustained gene expression, such as gene therapy. However, these vectors can be complex to produce and require careful handling due to the potential for insertional mutagenesis.
Adenoviral Systems
Adenoviral systems offer another high-efficiency option for mRNA delivery. Similar to lentiviral systems, adenoviral vectors can deliver genetic material effectively, though they typically do not integrate into the host genome. Their advantage lies in their ability to induce robust and transient expression, making them a popular choice for research applications.
The unique feature of adenoviral systems is their large capacity for genetic material, which allows for the delivery of multiple genes simultaneously. They are less likely to cause long-term effects compared to lentiviral vectors. On the downside, adenoviral transductions often incite immune responses, which can affect experimental results and limit their therapeutic use.
Understanding these various methods of mRNA transfection—their mechanics, advantages, and limitations—sets the foundation for effective application in genetic engineering. As you dive deeper into the workings of Cas9 mRNA, these methods will allow you to harness the technology to its fullest potential.
Performance Evaluation of mRNA Transfection
The core of successful gene editing using Cas9 mRNA hinges on the effectiveness of mRNA transfection. This phase is critical, as it determines how well the mRNA can be delivered into target cells for proper expression and subsequent action of the Cas9 protein. Evaluating performance is not just a box to check; it shapes the very framework of experimental viability and reproducibility in genetic engineering.
From assessing transfection efficiency to examining the stability of the transfected mRNA, these evaluations allow researchers to understand not only whether the mRNA is being taken up by the cells but also how well it endures the biological environment once inside.
Assessment of Transfection Efficiency
Evaluating transfection efficiency is the linchpin for understanding the success of mRNA delivery methods used in experiments. It highlights how effectively the mRNA gets into the intended cells and translates into the Cas9 protein that initiates gene editing. A more efficient transfection means better outcomes in experiments, facilitating advances in research applications and therapeutic strategies. There are various techniques available to quantify this efficacy, and every single method has its own nuances.
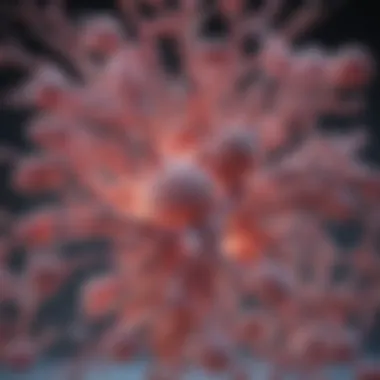
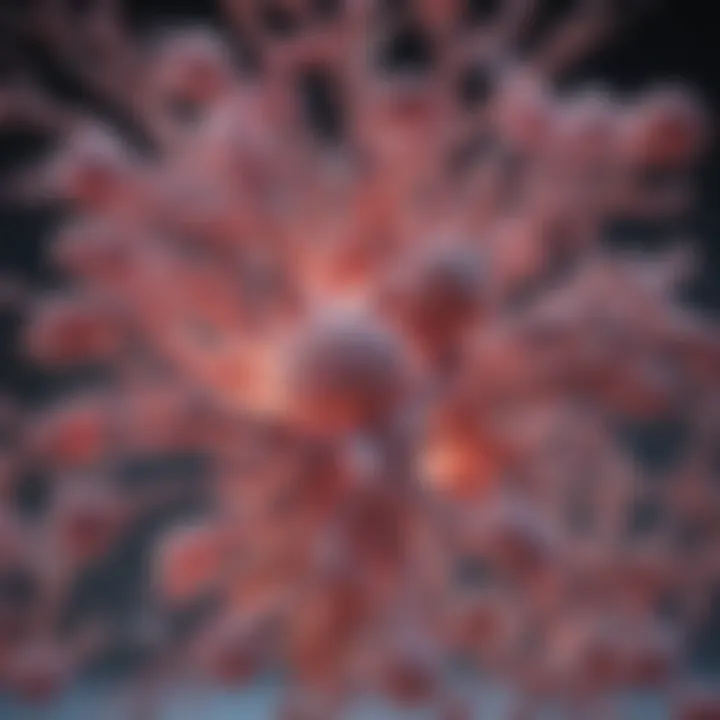
Quantification Techniques
Quantification techniques serve as the backbone when measuring transfection efficiency. Their major advantage lies in the precision they offer researchers to gauge how much of the delivered mRNA is functional after transfection. Techniques such as qPCR (quantitative Polymerase Chain Reaction) and Western blotting provide specific metrics and give insightful details about the mRNA uptake.
For example, qPCR allows for quantitative measurement of mRNA levels in cells, providing a clear view on how much mRNA has made its way post-delivery. On the downside, it can be somewhat complex and require calibration to get accurate readings.
- Pros of Quantification Techniques:
- Cons of Quantification Techniques:
- High precision in measurement.
- Ability to track specific mRNA species.
- Requires sophisticated equipment and techniques.
- Potential for operator error due to technicality.
Fluorescent Markers
Fluorescent markers represent another innovative means to assess transfection efficiency. By tagging mRNA with fluorescent dyes, researchers can visualize and track where the mRNA goes within the cell. This method makes it easier to determine both localization and quantity within cells.
The key attractive feature of using fluorescent markers is their ability to provide immediate visual confirmation of mRNA uptake, as well as qualitative assessments regarding cell distribution. However, these fluorescent tags can sometimes alter the behavior of the mRNA itself, leading to issues in experimental results.
- Pros of Fluorescent Markers:
- Cons of Fluorescent Markers:
- Real-time visualization of mRNA delivery.
- Easy to apply across various cellular contexts.
- Potential alteration to mRNA stability.
- Limited to cells that can be visualized, i.e., not all tissues are amenable to imaging.
Stability of Transfected mRNA
The mRNA's stability post-transfection is another pivotal area of focus. After all, how long the mRNA remains functional within the cellular environment affects the duration and success of gene expression. A stable mRNA can lead to prolonged protein production, which is essential for achieving desired cellular outcomes. Factors like enzymatic degradation and cellular storage mechanisms ultimately determine this stability.
Overall, a thorough evaluation of mRNA transfection performance brings to light the different techniques and their implications. With the right balance of efficiency and stability assessments, researchers can push the limits of Cas9 mRNA technology, adding layers of complexity and utility to genetic manipulation.
Applications of Cas9 mRNA Transfection
The applications of Cas9 mRNA transfection carve out a significant niche in the expansive landscape of genetic engineering. This segment highlights key facets of its utility that underscore its importance in contemporary research and practical applications. Cas9 mRNA transfection leverages the precision of the CRISPR technology while enhancing the efficiency of gene modifications across various areas. These applications not only demonstrate the versatility of the technology but also pave the way for innovations that might have seemed far-fetched in the past.
Research Applications
Gene Function Studies
Gene function studies are a cornerstone of molecular biology, allowing scientists to dissect the roles of specific genes within biological pathways. By harnessing Cas9 mRNA transfection, researchers gain a powerful tool for precisely editing genes within an organism. This precision is one of the standout characteristics of this method. Through targeted gene disruption or modification, understanding gene functionality becomes much clearer. One beneficial aspect is the ability to conduct these studies across various organisms, ranging from tiny yeast to larger animal models.
A unique feature of gene function studies utilizing Cas9 is the rapidity with which researchers can obtain results, thanks to the efficient nature of mRNA transfection. However, a downside might be the off-target effects that can arise, necessitating careful design and validation of the guide RNA to minimize unintended genetic modifications.
Animal Model Development
Animal model development plays a critical role in biomedical research, particularly for studying diseases and testing therapeutics. The ability to modify genes in model organisms such as mice or zebrafish makes Cas9 mRNA transfection a popular choice for developing these models. Researchers can tailor these animals to exhibit specific phenotypes, thus mimicking human diseases and paving the way for potential treatments.
The standout characteristic here is the adaptability of this method. With Cas9, various disease models can be generated with relative ease. An example includes developing models for genetic disorders, where researchers can knock out or insert genes effectively. Nevertheless, researchers also face challenges, such as ethical concerns surrounding animal experimentation and the complexity of creating viable models that accurately represent human conditions.
Therapeutic Applications
Gene Therapy
Gene therapy represents a transformative approach to treating genetic disorders. The incorporation of Cas9 mRNA transfection into gene therapy routines has propelled this field to new heights. One of the defining characteristics of gene therapy is its ability to correct defective genes responsible for disease onset. By targeting these altered genes, scientists can employ Cas9 to either knock out the faulty allele or replace it with a healthy one.
The impressive versatility of gene therapy enabled by Cas9 mRNA transfection marks it as a beneficial strategy. Importantly, the transient expression of Cas9 from mRNA reduces the risk of permanent alterations to the genome, hence minimizing long-term side effects. However, it’s crucial to acknowledge potential challenges, including delivery mechanisms and ensuring a precise editing process without off-target effects.
Oncological Interventions
Oncology is another critical area where Cas9 mRNA transfection has made strides. In oncological interventions, this technology can target and silence genes that are overactive in cancer cells. One of the key attributes of oncological applications is the selectivity it offers. By narrowing down specific cancer driver genes, Cas9 can play an integral role in developing targeted therapies.
Moreover, its unique feature lies in the ability to induce gene edits that can make cancer cells more susceptible to existing treatments, thus aiding in combinational therapy strategies. However, this approach is not without its pitfalls; challenges such as targeting accuracy and ensuring effective delivery to tumors require ongoing research and fine-tuning.
Plant Biotechnology
The significance of Cas9 mRNA transfection extends into plant biotechnology, a domain witnessing rapid advancements due to the precision of gene editing. The ability to modify genes within crops implies greater attributes such as enhanced resistance to diseases or improved nutritional profiles. Utilizing Cas9 in crops can result in quicker generation of desired traits compared to traditional methods of breeding.
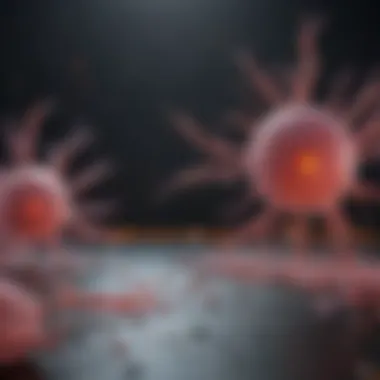
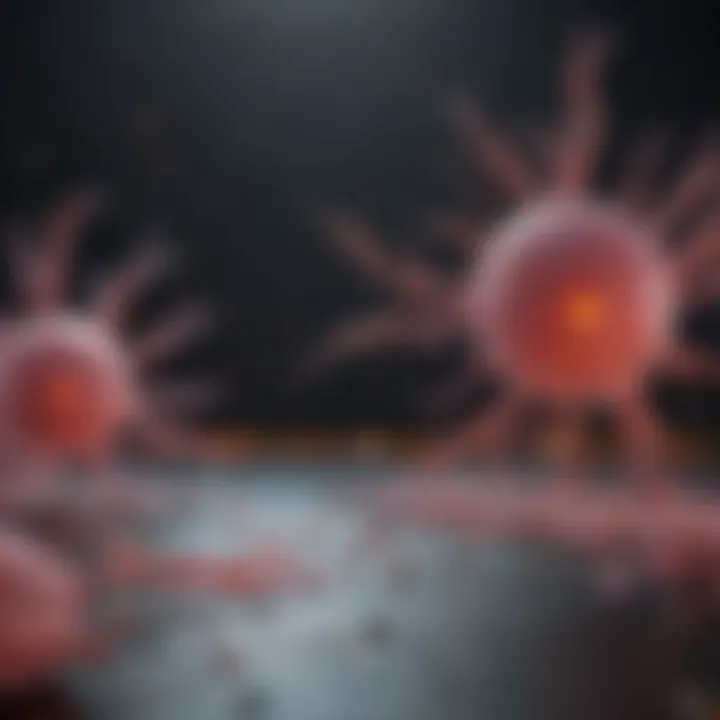
A significant advantage of this approach in plants is the specificity of target gene modification, which can enhance both yield and resilience of various crop species. Nevertheless, dependency on regulatory frameworks varies, as public acceptance of genetically modified organisms can occasionally hinder widespread application. The potential for tailored crops, offering more sustainable food sources, is a promising facet moving forward.
Challenges in Cas9 mRNA Transfection
The use of Cas9 mRNA transfection has brought a transformation in the world of genetic engineering, but it isn't all smooth sailing. There are key hurdles that researchers face which can influence the success rate of these techniques. Recognizing these challenges is paramount, as it shapes the future of genetic research and application in real-world settings. This section delves into the notable aspects such as the cellular immune response, off-target effects, and limitations of delivery efficiency, underscoring their significance in driving innovative solutions.
Cellular Immune Response
When introducing foreign mRNA into cells, the body’s immune system can go on high alert. This cellular immune response can lead to a variety of complications, including inflammation and even apoptosis. The recognition of mRNA as a foreign entity by the immune system is problematic. Studies have pointed out that certain sequences within the mRNA can stimulate toll-like receptors (TLRs), triggering an immune response that could undermine the effectiveness of Cas9 mRNA transfection.
Thus, navigating around this immune response is important. Researchers are exploring various strategies to mitigate these reactions. This includes modifying the mRNA structure to reduce immunogenicity, using chemical modifications in mRNA, or employing delivery methods that bypass immune detection.
Off-Target Effects
One of the critical issues associated with Cas9 technology is the risk of off-target effects. In simple terms, this means the Cas9 protein may not only cut the intended DNA sequence but could also strike other locations within the genome.
These unintended edits can lead to unpredictable results, potentially causing harmful mutations. For instance, if a gene responsible for cell growth is inadvertently targeted, it might lead to unchecked cell proliferation, raising concerns for therapeutic applications.
To counteract this challenge, many researchers are engaging in the development of more precise guide RNAs, which are designed to minimize these off-target interactions. Tools like high-throughput sequencing can also be utilized to identify and validate potential off-target sites, ensuring greater accuracy in gene editing initiatives.
Delivery Efficiency Limitations
Despite advances in transfection techniques, delivery efficiency remains a major stumbling block in the application of Cas9 mRNA. The effectiveness of this gene editing tool hinges on the ability of the mRNA to reach the target cells in an intact form.
Factors influencing delivery efficiency include the physical properties of the mRNA, the method of delivery, and the physiological conditions of the target tissue. For instance, certain lipid-based transfection methods may have difficulty penetrating dense cellular membranes or may degrade mRNA before it achieves its intended destination.
Moreover, achieving uniform delivery across diverse cell types can pose additional challenges. Thus, ongoing research is heavily focused on optimizing delivery systems to enhance transfection efficiency. This might involve using novel nanoparticles or developing hybrid delivery systems that combine the strengths of various methods.
"Understanding these challenges isn't just about finding obstacles; it's about paving pathways to better solutions."
Future Perspectives in mRNA Transfection
The potential of mRNA transfection technology, particularly in the context of Cas9, opens numerous avenues for exploration and innovation in genetic engineering. As researchers dig deeper into the intricacies of mRNA applications, it is clear that the future holds promises not only for scientific advancement but also for practical implementations in the medical and biotechnology fields. Understanding and harnessing these insights is crucial, as the evolution of mRNA transfection seems to be at a tipping point, potentially revolutionizing how we approach gene editing and therapeutic interventions.
Innovations in Delivery Technologies
Emerging technologies in delivery systems are paramount to enhancing the effectiveness of mRNA transfections. One fascinating area of development is the evolution of nanoparticles, which can encapsulate mRNA and deliver it directly to target cells in a more efficient manner. Innovations such as lipid nanoparticles have already started to take center stage, with their ability to facilitate cellular uptake while providing protection to the mRNA from degradation. As new materials are explored, researchers are hopeful to develop methods that both increase the specificity of delivery and reduce cytotoxicity, which is essential for therapeutic applications.
Furthermore, mesoporous silica nanoparticles and polymeric carriers are gaining attraction due to their tunable properties. These novel platforms offer the potential to control release kinetics and improve tissue targeting. With advancements in bioengineering, it’s likely we will see the creation of multifunctional nanoparticles, combining imaging and therapeutic capabilities, giving rise to more precise interventions.
Advancements in mRNA Stability
Stability of mRNA is another significant consideration that will influence the future trajectory of this technology. Naturally, mRNA is prone to degradation by cellular enzymes, so efforts are targeted towards enhancing its stability. Chemical modifications, such as the incorporation of 5’ cap structures and nucleoside analogs, are some strategies that have shown promise. These modifications not only protect mRNA from enzymatic decay but also boost translation efficiency.
In addition to chemical modifications, researchers are examining the role that storage conditions play in mRNA stability. Developing storage systems that preserve the integrity of mRNA during transport and storage remains a challenge. Reliable formulations could drastically influence the practicality of mRNA therapeutics, particularly outside of laboratory settings. This is where the intersection of scientific inquiry and real-world application becomes vital.
Potential Regulatory Challenges
As with any cutting-edge technology, regulatory challenges present another hurdle that could affect the adoption of Cas9 mRNA transfection techniques. Agencies like the FDA and EMA are constantly updating their guidelines to ensure safety and efficacy, yet the fast pace of innovations often leads to moving targets for compliance. For instance, the approval pathways for new delivery systems or modified mRNA constructs remain somewhat murky.
These regulatory complexities require ongoing dialogue between researchers, companies, and regulators to ensure that advancements in mRNA transfection can meet the necessary regulatory frameworks. Engaging in discussions about ethical implications, safety assessments, and long-term monitoring is also crucial in helping shape policies that can support sustainable innovation in the field.
"As we glance at the future of mRNA research, the convergence of stability enhancements, innovative delivery mechanisms, and balanced regulation will shape the landscape of genetic engineering and therapy."
In summary, the future of Cas9 mRNA transfection holds tremendous promise. With ongoing advancements in delivery technologies, stability of mRNA constructs, and a and regulatory conversations, this field stands at the brink of remarkable transformations. The implications for research, therapeutics, and biotechnological applications could be profound, making it essential for students, researchers, and professionals alike to stay abreast of these developments.
Culmination
The exploration of Cas9 mRNA transfection encapsulates a significant frontier in genetic engineering and molecular biology. This conclusion serves to tie together the numerous strands of discussion that has unfolded throughout the article. By understanding the mechanisms of Cas9 and its applications in various fields, a holistic view of its potential emerges.
Summary of Key Findings
Throughout the article, it became evident that Cas9 mRNA transfection is not merely a technological advance; it's a transformative approach with far-reaching effects. The key findings can be summarized as follows:
- Mechanistic Insight: We delved into the intricate workings of the Cas9 protein, highlighting its structure and interaction with guide RNA, as well as its mechanism for cleaving target DNA.
- Transfection Methods: Different methodologies used for mRNA transfection were examined, ranging from physical and chemical techniques to viral delivery systems. Each method offers distinct advantages and limitations that researchers must navigate.
- Diverse Applications: Applications cut across various domains, from research in genetic functions to direct interventions in therapy and innovations in plant biotechnology. Each application carries implications for how we can leverage genetic edits for societal benefits.
- Challenges: Potential obstacles related to cellular immune responses, off-target effects, and delivery efficiency were also discussed. Addressing these challenges is vital to refining the application of mRNA transfection.
Implications for Future Research
Looking ahead, the implications of Cas9 mRNA transfection for future research are broad and promising. Here are a few noteworthy considerations:
- Innovative Delivery Systems: The quest for more efficient and targeted delivery methods will continue to be at the forefront. Improved systems can enhance the precision of gene editing applications.
- Understanding Side Effects: As research advances, it's crucial to better understand the biology behind off-target effects and immune responses, ensuring that future applications have their safety thoroughly vetted.
- Expanding Applications: New areas of inquiry may emerge, particularly in areas such as regenerative medicine or advanced agricultural practices. The flexibility of Cas9 technology hints at untapped avenues.
- Policy and Ethics: As we push boundaries in genetic engineering, a careful balance between innovation and ethical considerations must be maintained. Regulatory frameworks need to adapt to these rapid advancements to safeguard public interest.
In summation, the journey through Cas9 mRNA transfection offers critical insights that are shaping the landscape of genetic manipulation. By recognizing the benefits, challenges, and future directions, we prepare not only to embrace the technology but to do so responsibly.