Understanding Basic Limits in Scientific Research
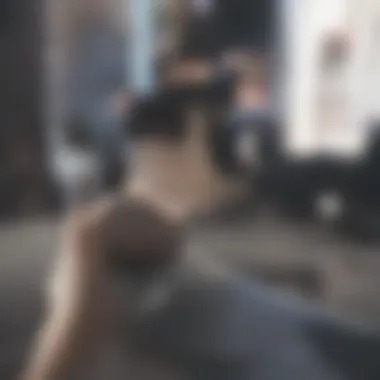
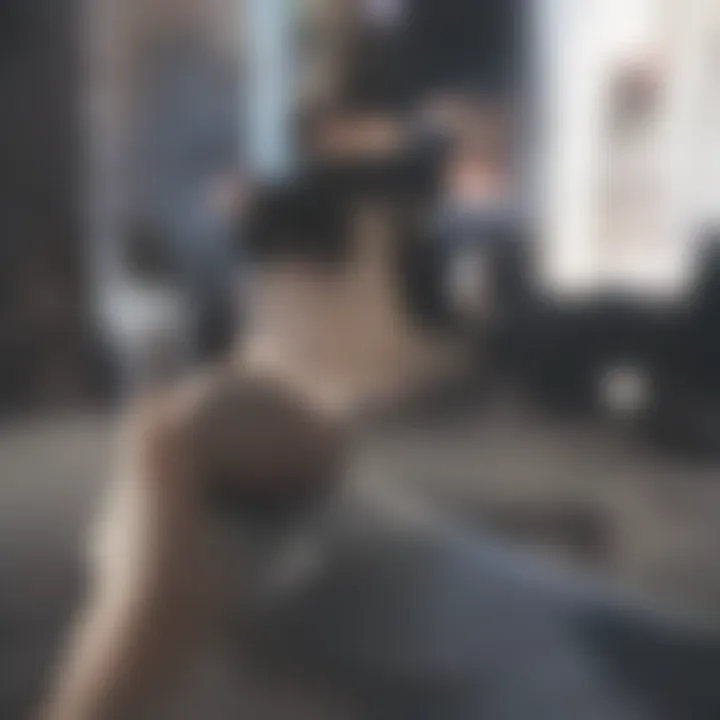
Intro
This article aims to illuminate these fundamental boundaries, helping readers to recognize both their practical and ethical implications. By dissecting the various factors that constitute these basic limits, we hope to enhance the critical thinking skills necessary for researchers and students in the scientific community.
Defining Basic Limits
Understanding the concept of basic limits is essential in scientific research. These limits encompass various aspects that define the boundaries within which researchers operate. By grasping these restrictions, one can better appreciate the implications for methodologies, ethical considerations, and even the formulation of research questions. Defining these limits helps to clarify the scope of inquiry and establishes parameters that guide scientists in their work.
Conceptual Framework
The conceptual framework of basic limits serves as a foundation for understanding how scientific research is conducted. These limits may stem from various factors, including theoretical constraints, methodological boundaries, and practical aspects of research execution. Each discipline has its unique set of limits, which shapes everything from hypothesis development to data collection and analysis.
For instance, in biology, genetic constraints may define the possible variations that can arise within a species. In contrast, in chemistry, thermodynamic principles dictate how reactions occur under certain conditions. By establishing clear definitions of these basic limits, researchers can develop more targeted and effective approaches to their investigations.
Historical Context
Historically, the understanding of limits in scientific research has evolved. Early scientists often worked without a clear sense of established boundaries, which sometimes led to misleading conclusions. As disciplines advanced, researchers began to recognize the significance of understanding their limits.
For example, the formulation of the laws of thermodynamics in the 19th century provided chemists with a framework to comprehend energy transformations. This historical development illustrates how recognizing and defining limits leads to more informed and reliable scientific practices. In summary, both the conceptual framework and historical context of basic limits are vital in guiding future research endeavors.
Importance of Understanding Limits
Understanding the basic limits in scientific research is crucial for several reasons. These limits set boundaries for what can be achieved within various fields, including biology, chemistry, physics, and earth sciences. Recognizing these constraints allows researchers to align their efforts with realistic expectations. This means less time spent on unproductive avenues of inquiry and more focus on exploration that can yield substantial contributions to knowledge.
Guiding Research Directions
Basic limits influence the direction scientists take in their investigations. Without a grasp of what is feasible or possible, research can veer into areas that may lead to inconclusive or meaningless results. For instance, in biology, genetic constraints may determine which organisms are suitable for certain experiments. Researchers must consider these factors to avoid misdirected efforts.
Additionally, understanding limits helps in prioritizing research questions. When limits are accepted, it becomes easier to identify areas that require more attention or that have yet to be explored. This prioritization can streamline research initiatives and promote progress in key areas. In that way, researchers can be tactical when selecting projects, thus improving their chances of success.
Formulating Hypotheses
Creating hypotheses is a fundamental aspect of scientific research. However, formulating effective hypotheses requires a sound understanding of the limits that govern the field of study. Basic limits can shape the parameters within which scientists can work. This understanding ensures hypotheses are not only creative but also grounded in reality.
When researchers develop hypotheses, they need to consider the practical constraints that may affect their experiments. For example, in chemistry, thermodynamic principles can limit the reactions that can realistically occur. By acknowledging these principles during hypothesis formulation, researchers refine their focus and improve the quality of their predictions. This method leads to stronger, more testable hypotheses.
In summary, understanding the importance of limits in scientific research enhances both the direction and the quality of investigatory work. It fosters a clearer vision of what is achievable and encourages more strategic and well-informed approaches to inquiry.
Basic Limits in Biology
Biology, as a study of living organisms, inherently involves various basic limits that shape our comprehension of life itself. These limits stem from genetic, ecological, and evolutionary constraints. Understanding these limits is essential for students, researchers, educators, and professionals, as they can directly impact research outcomes and methodologies. Thoughtful consideration of these constraints not only informs study design but also fosters a more nuanced interpretation of biological data.
Genetic Constraints
Genetic constraints refer to the limitations imposed by the genetic makeup of organisms. These constraints can influence evolution, development, and ultimately, the diversity of life forms. For example, certain traits are inherited and cannot evolve or change significantly due to the stability of genetic information. This stability can hinder rapid adaptation to environmental changes.
Key points to consider about genetic constraints include:
- Inheritance Patterns: The manner in which traits are passed down affects evolution. Traits that offer survival benefits might not be expressed due to recessive alleles.
- Mutation Rates: The frequency of genetic mutations can limit biological innovation. A high mutation rate might lead to instability, whereas a low rate can restrict potential adaptations.
Understanding these aspects allows researchers to better frame their biological inquiries and anticipate the outcomes of genetic interventions.
Ecological Boundaries
Ecological boundaries represent the various constraints imposed by an organism's environment. Each species operates within a specific ecological niche, which sets limits on its growth, reproduction, and survival.
Factors contributing to ecological boundaries include:
- Resource Availability: The amount of food, water, and shelter can limit population sizes and species distributions. For instance, habitats with scarce resources cannot support large populations.
- Inter-species Interactions: Competitions, predation, and symbiosis shape community dynamics. These interactions can create clear boundaries for species, determining where and how they thrive.
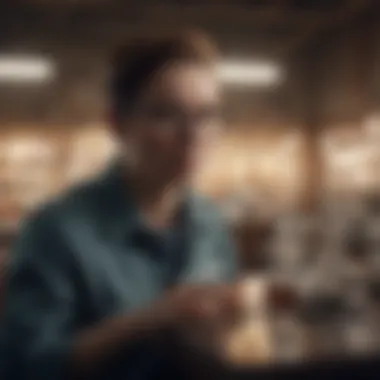
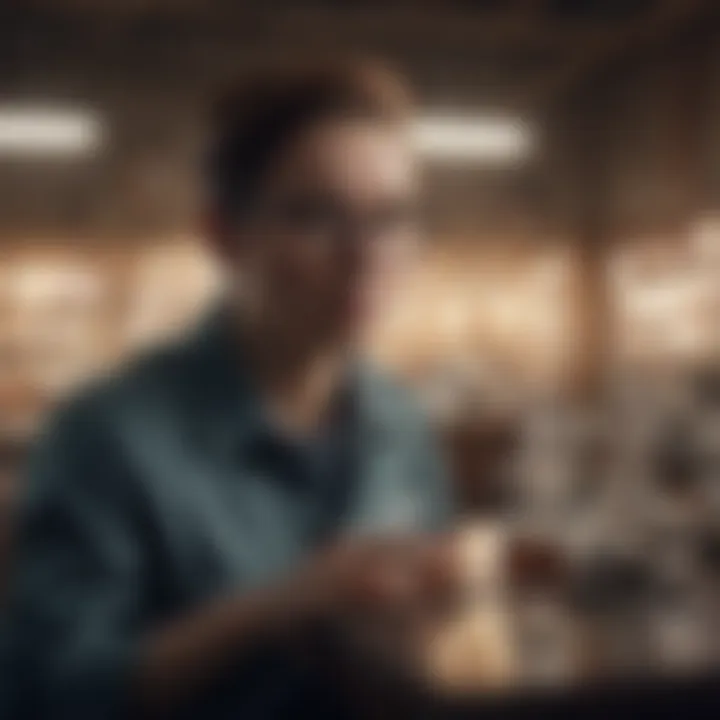
Addressing ecological boundaries is critical for conservation efforts and understanding species' responses to environmental changes. Researchers can analyze how these limits inform biodiversity and ecosystem health.
Understanding basic limits in biology is critical because it lays the groundwork for making informed decisions in research and conservation practices.
Basic Limits in Chemistry
In the realm of chemistry, understanding basic limits is paramount for several reasons. These limits not only define the scope of research but also guide the development of new theories and applications. Basic limits in chemistry often reside in the fundamental principles governing chemical reactions and interactions. By appreciating these limits, chemists can streamline methodologies, improve experimental designs, and ultimately contribute to the advancement of scientific knowledge.
Thermodynamic Principles
Thermodynamics offers a crucial framework for understanding chemical processes. It focuses on energy transfers and the spontaneity of reactions. The laws of thermodynamics set essential boundaries that chemists must recognize when designing experiments. For instance, the first law, which deals with the conservation of energy, implies that energy cannot be created or destroyed, only transformed. Insights from thermodynamic principles help researchers optimize conditions for reactions, making them more efficient.
Considerations such as enthalpy and entropy play a vital role in predicting whether a reaction is feasible under specified conditions. Analyzing these variables allows chemists to assess reaction pathways effectively. Failure to grasp thermodynamic limits could lead to experimental designs that waste resources or yield suboptimal results. Therefore, understanding these principles leads to better informed, more effective experimental strategies.
Reaction Kinetics
Reaction kinetics addresses the rate at which chemical reactions occur. This field provides insights into how various factors, like concentration and temperature, influence reaction speed. The basic limits inherent in reaction kinetics help researchers understand what drives a reaction towards completion.
Key elements of reaction kinetics include the concepts of activation energy and rate constants. For instance, the collision theory posits that for a reaction to occur, reactants must collide with sufficient energy. This insight delineates practical boundaries within which kinetic studies must operate. Failing to negotiate these limits might lead to misconceptions about reaction behavior.
Moreover, researchers can benefit immensely from modeling kinetic scenarios. By employing mathematical expressions such as the Arrhenius equation, they can derive critical information regarding temperature dependence and activation energies. Understanding these rate laws not only aids in the optimization of chemical processes but also enhances predictive capabilities regarding reaction outcomes.
"In chemistry, basic limits are more than constraints; they are the guiding principles leading to innovation and efficiency."
Basic Limits in Physics
Understanding basic limits in physics is crucial. This field governs our understanding of the universe. These limits define the boundaries of what can be observed, measured, and understood. Without recognizing these constraints, research may lead to oversights or erroneous conclusions. The principles of physics often dictate the framework within which all scientific investigations operate. They set foundational rules that inform methodologies across various research disciplines.
Relativity and Its Constraints
Albert Einstein's theory of relativity reshaped physics. It introduced concepts that seemed counterintuitive but are crucial for understanding cosmic and quantum phenomena. One central notion is that the laws of physics must be consistent for all observers, regardless of their relative motion. The implications of this are profound. For instance, time can pass at different rates for observers in different gravitational fields or moving at different velocities. This challenges our conventional notions of time and space.
The constraints of relativity also highlight limits in experimental physics. Observations made at extreme velocities or in strong gravitational fields may yield results that deviate from traditional physics. These findings might lead researchers to rethink existing theories. Therefore, a strong grasp of relativity is essential for scientists. It influences how experiments are designed and how results are interpreted, especially in fields like astrophysics and cosmology.
"Relativity is a fundamental concept, shaping the inquiry into the nature of reality. Understanding its constraints enables deeper insights into physical laws."
Quantum Mechanical Limits
Quantum mechanics introduces another level of complexity in understanding basic limits in physics. The behavior of particles at the quantum level often defies classical intuitions. One of the essential principles here is the uncertainty principle, proposed by Werner Heisenberg. It states that there are fundamental limits to how precisely we can know the position and momentum of a particle simultaneously. This has significant implications for experimental physics.
At the quantum level, particles can exist in multiple states until observed. This phenomenon challenges conventional experimental designs. It enjoys a significant role in fields such as quantum computing and particle physics, where researchers must account for the probabilistic nature of matter. Understanding these quantum limits helps shape theories about matter and energy.
As we explore the frontiers of physics, these basic limits become crucial in our pursuit of knowledge. They remind us that while we may strive for absolute understanding, nature often possesses inherent constraints.
Basic Limits in Earth Sciences
In the realm of scientific research, the study of Earth sciences provides a rich field for understanding basic limits. These limits are significant as they shape our comprehension of the Earth's systems and their interactions. The essence of Earth sciences lies in its broad scope, which includes geology, meteorology, oceanography, and environmental science. Thus, acknowledging the constraints found within these disciplines is crucial for advancing our knowledge and ensuring sustainable practices.
Geological Time Scales
Geological time scales represent a cornerstone in understanding Earth's history. They define the chronological framework used by scientists to map events across millions and billions of years. By delineating various Eons, Eras, Periods, epochs, and ages, researchers can contextualize geological developments with respect to time. These scales highlight the limits of our temporal perception in relation to the Earth's processes, emphasizing the vastness that can often surpass human comprehension.
Studying geological time scales holds several implications:
- Temporal Limitations: Recognizing the rates of geological and biological change constrains expectations of what can be observed directly.
- Predictive Challenges: Appreciating the limits of geological time scales can lead to limitations in predicting future geological events or phenomena.
- Impact on Fossil Records: Understanding the time scales helps contextualize fossil distribution, which is essential for studies in paleontology and evolution.
The geological time scale serves not only as a scientific tool but also as a reminder of the limits inherent in our ability to observe and understand the processes shaping our planet over eons.
Environmental Constraints
Environmental constraints are key factors influencing Earth sciences research. These constraints cover a broad array of elements including climate, landforms, ecosystems, and resource availability. Understanding environmental limits is essential, as they dictate both the feasibility and the sustainability of human interaction with the planet.
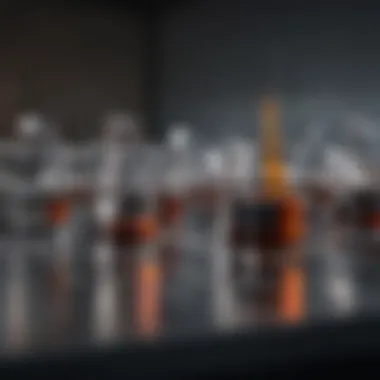
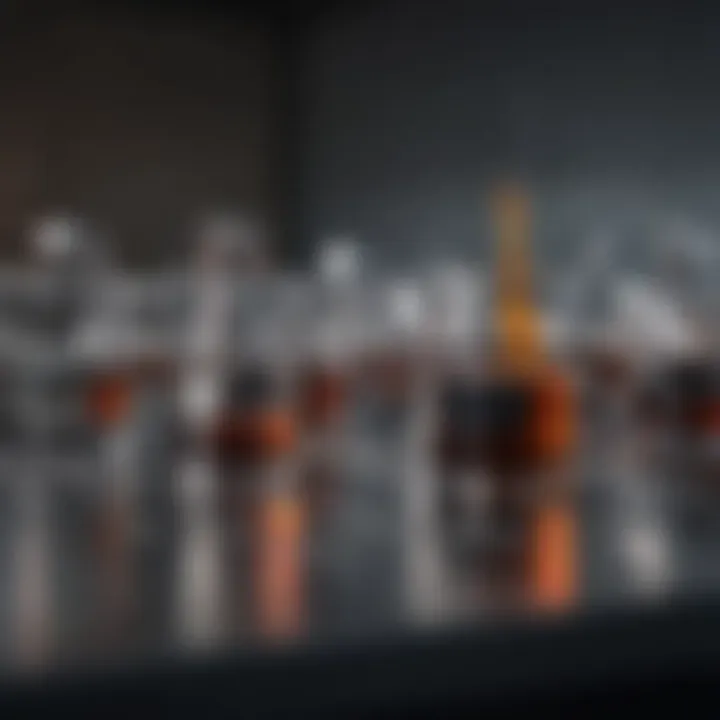
Several aspects highlight the significance of these constraints:
- Resource Allocation: Limited natural resources necessitate careful study and management. This leads researchers to confront boundaries regarding what can be sustainably harvested or utilized.
- Ecosystem Dynamics: Environmental limits shape biodiversity. Studies on species adaptation reveal how organisms respond to limiting factors such as temperature, water availability, and nutrient supply.
- Climate Variability: The interaction of human activities and natural systems imposes further constraints. Climate change exemplifies how alterations in Earth systems can redefine limits, creating challenges for adaptation and mitigation.
These constraints urge researchers and policymakers to consider the impacts of their actions on Earth's systems and to think critically about sustainable practices.
"A comprehensive grasp of the environmental constraints can aid in navigating the complexities of Earth's systems and support effective strategies for sustainable development."
In sum, recognizing and understanding basic limits in Earth sciences contributes to a more meaningful engagement with our planet. It pushes the boundaries of traditional research, inviting scholars to navigate the intricate balance between Earthโs natural processes and human endeavors.
Ethical Considerations Related to Limits
In scientific research, ethical considerations often intersect with the concept of basic limits. Understanding these limits is crucial for ensuring that research is conducted not only rigorously but also responsibly. Ethical dimensions can shape the choices researchers make and can promote societal trust in scientific findings.
Research Integrity
Maintaining research integrity involves adhering to ethical standards while recognizing the inherent limits of scientific inquiry. This includes a commitment to transparency, accuracy, and accountability in research practices. Researchers must be aware of their study's limitations and report them honestly. Failing to do so can lead to the propagation of false information and may ultimately impede scientific progress.
The importance of integrity extends to the treatment of data. Misrepresenting results to meet funding expectations or personal ambitions poses risks to the entire research community. These actions can damage credibility and lead to public mistrust in scientific institutions.
To foster integrity, research institutions can implement rigorous training programs that emphasize the ethical implications of potential limits. Educators should encourage students and early-career researchers to critically assess their findings and understand how methodological constraints shape the knowledge produced.
Impact on Society
The consequences of ignoring ethical considerations related to limits in research can be profound. When researchers fail to recognize and communicate the limits of their studies, the findings disseminated to the public can mislead stakeholders, policymakers, and practitioners. This is especially critical in fields that directly affect public health or environmental sustainability, where misinterpretation can lead to harmful practices or policies.
Moreover, ethical considerations should not only encompass the immediate outcomes of research. They also entail recognizing the broader societal implications of scientific findings. This is particularly relevant when addressing contentious or sensitive topics, where the potential for misuse of research results is high.
Given these impacts, fostering an ethical mindset among researchers is vital. This can be achieved through:
- Encouraging collaborative discussions about ethical dilemmas.
- Establishing clear guidelines for ethical research practices.
- Promoting community engagement to keep research aligned with public interests.
In summary, understanding and respecting the ethical considerations related to limits greatly enhance the quality of scientific research and its acceptance in society.
Experimental Design and Basic Limits
Experimental design forms the backbone of any scientific inquiry. It includes the plan or strategy used to investigate hypotheses and gather data. Understanding the basic limits in experimental design ensures that researchers can assess reliability and validity of their findings. These limits help outline what is possible and what constraints may impede the investigation. Furthermore, recognizing these boundaries can guide researchers in refining their methods and drawing sound conclusions.
Limitations in Methodology
Methodological limitations pose challenges to researchers in all fields of study. The effectiveness of a given method is crucial to the quality of data we obtain. Several factors contribute to these limitations, including sample size, measurement tools, and procedural consistency. For instance, using an inadequate sample size can lead to misleading conclusions, as the results may not truly represent the population.
- Sample Size: A small number of participants can introduce significant variability, skewing results.
- Measurement Tools: Instruments must be precise. Poorly calibrated tools can produce data that lacks accuracy.
- Replication: The ability to replicate experiments is vital. If results cannot be reproduced, questions arise about the original findings.
Despite limitations, innovative techniques continue to emerge that enhance experimental design. For example, the use of randomization and blinding can reduce biases, leading to more credible outcomes. Researchers must be diligent in acknowledging these boundaries in their published work.
Data Interpretation Challenges
Once data is collected, interpretation becomes the next critical step. Misinterpretation of data can occur due to biases or preconceived notions. This is where limitations in methodology can also affect conclusions. Proper statistical analysis is fundamental, yet it can often lead to confusion among practitioners.
Common data interpretation challenges include:
- Bias: Personal biases can distort interpretation. Awareness of oneโs biases is important.
- Statistical Overreach: Overinterpreting data can lead to drawing unwarranted conclusions. Researchers should avoid this by adhering closely to their hypotheses.
- Contextual Factors: Not considering external variables may mislead interpretations.
Overall, clear communication about methods and results is essential. This transparency helps the scientific community critically evaluate findings and their implications.
"Understanding basic limits provides a framework for evaluating the quality and credibility of research outcomes."
Acknowledging experimental design and its basic limits is critical in shaping robust scientific knowledge. It invites a systematic approach that ultimately leads to more sound and trustworthy research.
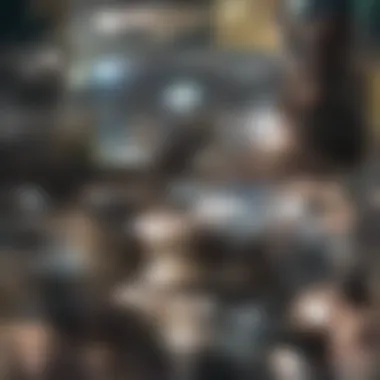
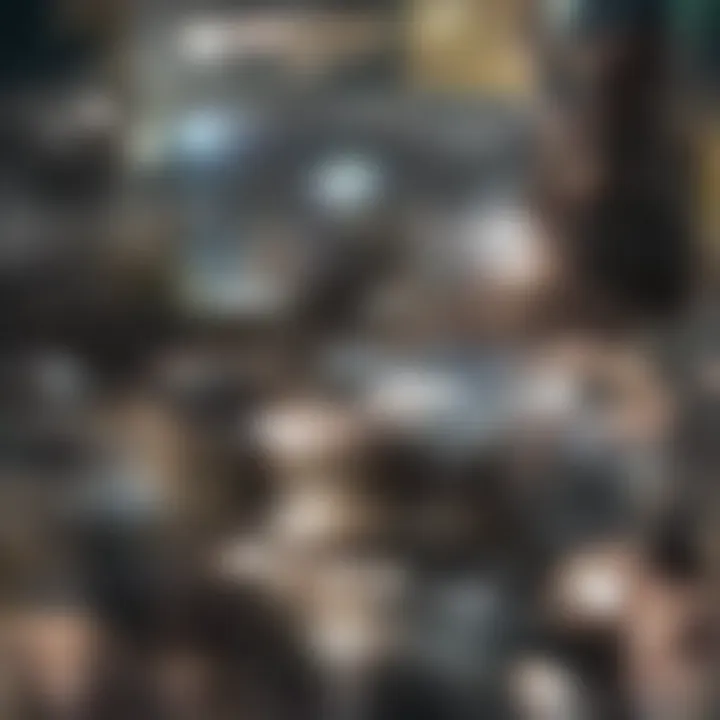
The Role of Basic Limits in Innovation
Understanding the role of basic limits in innovation is essential for numerous fields of scientific inquiry. Innovation is not simply about creativity and new ideas; it also involves navigating the boundaries and limitations that exist. The recognition of these limits can guide researchers toward more effective methodologies and push the boundaries of what is possible.
Basic limits can provide a foundation for technological advancements. When researchers understand what is constraining their work, they can design experiments and projects that either work within these boundaries or seek to alter them. For instance, in synthetic biology, genetic constraints push scientists to innovate in gene editing technologies like CRISPR. This understanding fosters valuable refinement in the tools at researchersโ disposal, leading to breakthroughs in healthcare and agriculture.
These limits are not merely hurdles; they can stimulate innovation. Early computer technology faced enormous barriers regarding processing power and storage. Yet, these limitations drove the development of more efficient algorithms and hardware designs. Thus, constraints allowed for the evolution of technology that we take for granted today.
"Innovative solutions often emerge from deep understanding of existing limitations and constraints."
Technological Advancements
Technological advancement relies heavily on the understanding of basic limits. As researchers encounter constraints, they often seek new solutions. This may involve the development of novel technologies or the alteration of existing ones. By recognizing the boundaries, scientists can identify opportunities for innovation.
For example, in energy research, the limits of current battery technology inform the pursuit of new materials and designs. Lithium-ion batteries, while prevalent, have limitations in energy density and recharge cycles. Understanding these factors, researchers explore alternatives like solid-state batteries or lithium-sulfur batteries. This search for alternatives not only enhances performance but could also lead to breakthroughs that transform entire industries.
Moreover, the enhancement of existing technology is often tied to overcoming limits. For instance, with the rise of machine learning, researchers are focused on overcoming limits pertaining to algorithmic efficiency and data availability. The ongoing development of techniques like transfer learning demonstrates how insights about limitations lead to substantial technological advancements.
Interdisciplinary Approaches
Interdisciplinary approaches play an increasingly important role in addressing basic limits in innovation. The boundaries of various scientific fields often impose restrictions that an interdisciplinary strategy can successfully navigate. By combining knowledge from different disciplines, researchers can create a more holistic understanding of a problem, ultimately leading to innovative solutions.
For instance, environmental science requires expertise in biology, chemistry, and geology. Challenges such as climate change cannot be tackled effectively by isolating any single discipline. Instead, by merging insights from various fields, new approaches emerge, such as carbon capture technologies. This significant fusion allows scientists to innovate in ways that single-discipline approaches cannot achieve.
Furthermore, engaging with diverse perspectives fosters creativity. Engineers collaborating with biologists might explore bio-inspired designs in robotics, leading to innovations that mimic nature to solve complex human problems. Thus, recognizing and overcoming basic limits through interdisciplinary collaboration yields substantial benefits.
Future Perspectives on Basic Limits
The study of basic limits in scientific research carries significant implications for the direction and efficacy of future methodologies. Understanding these limits allows researchers to focus their efforts on more realistic goals, promoting a culture of innovation while respecting the foundational constraints of various disciplines. As we progress, it becomes crucial to recognize both the potential benefits and the limitations these perspectives can introduce. This section will delve into specific emerging research areas and the global challenges associated with established boundaries.
Emerging Research Areas
Emerging research areas often lie at the intersection of established disciplines, challenging existing basic limits and proposing new theories and frameworks. Some such areas include:
- Synthetic Biology: This field combines biology and engineering, creating synthetic organisms and systems. As techniques in genetic manipulation progress, researchers face limits in biological systems understanding, necessitating further exploration to overcome these constraints effectively.
- Quantum Computing: The blend of computer science and quantum physics presents significant challenges inherited from current computational paradigms. The basic limits in processing capacity motivate researchers to explore new algorithms tailored for quantum systems, thus pushing the boundaries of technological capabilities.
- Artificial Intelligence: The integration of AI into various fields begs the question of ethical and practical limits. Researching how AI systems can operate within these constraints opens new possibilities in understanding human cognition and decision-making processes while respecting boundaries in ethics.
These emerging areas not only broaden our understanding of scientific limits but also encourage interdisciplinary collaboration, allowing for a richer exploration of ideas.
Global Challenges and Boundaries
Global challenges such as climate change, pandemics, and resource scarcity highlight the necessity of redefining basic limits in science. Coping with these issues often requires:
- Multidisciplinary Approaches: Dealing with complex global problems necessitates insight from diverse fields. Understanding the basic limits that apply to each discipline allows scientists to create comprehensive strategies that consider the intricate interplay between biological, chemical, and physical processes.
- Sustainability: The limits of our environmental resources pose critical questions about future research directions. Scientists must navigate the constraints of ecological sustainability while seeking innovative methods to preserve biodiversity and foster renewable resources.
- Global Health: The recent pandemic has exposed significant limits in our public health responses. Moving forward, researchers must comprehend these limitations to develop resilient health systems that adapt to future threats.
"The acknowledgment of basic limits is not simply a constraint; it is a guiding principle that enhances the significance of scientific inquiry."
Understanding the future perspectives in basic limits enables researchers to craft realistic goals, embrace innovation, and confront the intricate challenges that lie ahead.
Epilogue: The Significance of Basic Limits
Understanding basic limits is crucial for the advancement of scientific research. These limits shape the trajectory of inquiry, affecting how scientists formulate hypotheses, design experiments, and interpret data. Recognizing these boundaries helps researchers avoid pitfalls that can arise from a misunderstanding of the system they study.
Summary of Insights
Throughout this article, we delved into the essential nature of basic limits across various scientific fields. We examined genetic constraints in biology, thermodynamic principles in chemistry, and quantum mechanics in physics, among others. Each discipline has its unique set of parameters that influence outcomes and research directions.
- Conceptual Framework: Each limit serves as a lens through which the world is interpreted.
- Methodological Constraints: Limitations in experimental design guide what can be effectively studied.
- Ethical Principles: Understanding these limits ensures integrity in research practices.
The interplay of these factors leads to a more nuanced understanding of scientific inquiry and promotes responsible practices.
Implications for Future Research
The implications of understanding basic limits are profound. Recognizing constraints encourages researchers to think critically about their work. In many cases, acknowledging limitations directly influences the framing of research questions. This can lead to more robust and ethical inquiries.
- Emerging Research Areas: New technologies may challenge existing limits and unveil previously unexamined phenomena.
- Interdisciplinary Collaboration: Engaging with other fields can offer alternative perspectives on fundamental limits.
- Global Challenges: Addressing issues like climate change will require a deep understanding of the limits in environmental science.
"The limits of science may be the very edges of human understanding, pushing researchers to redefine boundaries continuously."