Exploring the Versatility of Porous Polymers
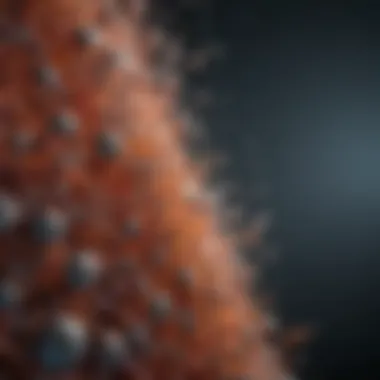
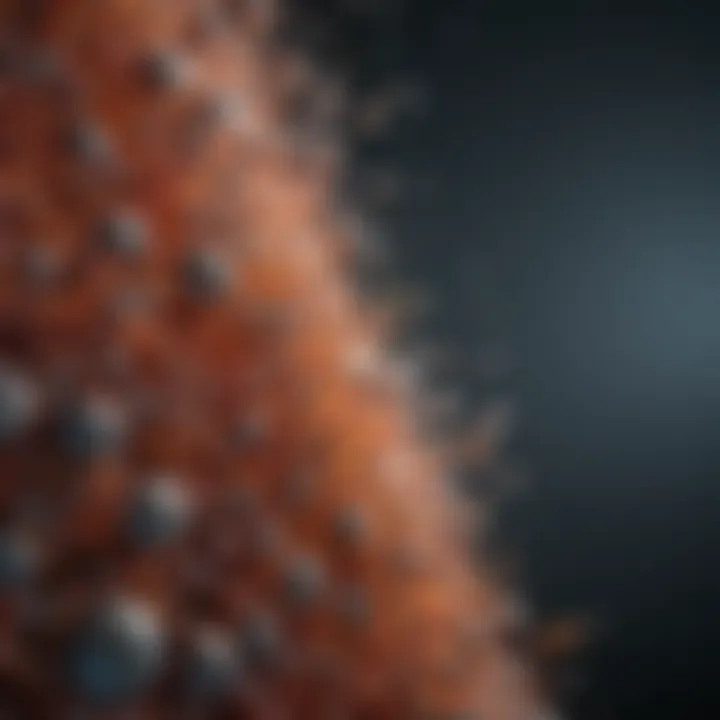
Intro
Porous polymers have emerged as a pivotal material in modern scientific research and industrial applications. Their unique structural characteristics —with a network of interconnected pores— grant them remarkable properties that span a variety of fields. Understanding the intricate nature of these materials is not just an academic pursuit; it holds the key to advancements in sustainability, healthcare, and environmental protection.
These polymers are synthesized through various techniques, yielding materials that can be tailored to meet specific needs from fine filtration in water treatment systems to targeted drug release in medical applications. Their versatility is largely due to the porous architecture, which allows for high surface areas, lightweight properties, and tunable mechanical attributes.
Imagine a sponge —but made of synthetic materials—that can trap or release substances on demand. This analogy serves to illustrate the multifaceted applications of porous polymers, making them invaluable in settings ranging from environmental remediation, where they aid in capturing pollutants, to the biomedical fields, where they facilitate effective drug delivery systems. Each application taps into these materials' unique traits, prompting a deeper exploration of their role in ongoing scientific innovation.
In this article, we will dissect the structural characteristics, synthesis methods, as well as the technological applications of porous polymers. Insights into their future potential, especially in biocompatibility, will delineate the significance these materials hold for both researchers and industry professionals alike.
Prologue to Porous Polymers
Porous polymers have captured the scientific community’s attention in recent years, given their remarkable versatility and multitude of applications. From environmental cleanup to advanced medical procedures, their unique properties allow them to tackle some pressing challenges across a variety of fields. By exploring the intricate world of porous polymers, we open doors to innovative solutions that are not only effective but also sustainable.
Understanding the significance of porous polymers involves delving into their defining characteristics and potential benefits. These materials, with their defined void spaces, can absorb, filter, and even release substances, presenting them as ideal candidates for numerous tasks.
Definition and Characteristics
At their core, porous polymers are organic materials with a networked structure that incorporates numerous voids or pores. These pores vary in size—from nanometers to millimeters—thus creating a rich interplay between surface area and porosity. This means they can be tailored to achieve specific properties suitable for various applications.
For instance, consider the BET surface area analysis, which plays a crucial role in assessing the surface area of these materials. This method is instrumental in determining their adsorption capabilities, acting as a measure of how effective a polymer can be in trapping or filtering substances. High surface area can significantly enhance the performance in applications like pollutant removal or drug delivery systems, where precise control is as important as functionality.
Additionally, pore size distribution directly affects the behavior of these materials in applications such as water treatment systems, where larger pores might facilitate quicker filtering but smaller ones may ensure finer filtration.
Historical Perspective
The roots of porous polymer science can be traced back to the mid-20th century. Initially, these polymers were synthetized for industrial purposes, such as packaging and insulation. However, the late 20th and early 21st centuries witnessed a surge in research focusing on their unique properties. Scientists began to investigate not only how to make these polymers but how to manipulate their structures to fit highly specialized roles.
One pivotal moment in this realm was the introduction of template-based synthesis methods in the 1990s. This technique allowed for greater control over pore sizes and distribution, pushing the boundaries of what was feasible with porous polymers. It laid the groundwork for pivotal advancements in various fields, particularly in healthcare and environmental remediation. As research expanded, so did the avenues for innovation, leading to a present day where the applications of porous polymers are as varied as they are critical to solving today’s challenges.
With this foundational understanding, the article now embarks on a detailed exploration of the structural composition, synthesis techniques, and, most importantly, the myriad applications of porous polymers that continue to reshape various industries and scientific advancements.
Structural Composition of Porous Polymers
Understanding the structural composition of porous polymers is crucial to grasp their functionalities and potential applications. These materials boast a unique microstructure, characterized by interconnected voids or pores that differentiate them from traditional polymers. The significance of this topic lies in how the microscopic architecture influences not only the physical properties of the polymers but also their efficacies in various applications, such as filtration, adsorption, and drug delivery. Their design caters to specific needs across multiple industries, making it vital to analyze how their structural elements contribute to their overall versatility and performance.
Chemical Structure Overview
The chemical structure of porous polymers is a fascinating interplay between polymer chains and void spaces. At its core, these polymers are often formed through the reaction of monomers that create a crosslinked network. This combination allows the creation of cavities, which can be engineered to achieve desired properties. The flexibility in this chemical design supports the customization of pore size and distribution, enabling the polymer to be tailored for unique applications.
One of the defining features in the chemical structure is the degree of crosslinking. Higher degrees can lead to more stable materials that resist deformation under mechanical stress, while lower degrees might produce softer, more pliable polymers. Furthermore, the choice of monomer plays a vital role in determining chemical stability and reactivity. Therefore, engineers and scientists must consider these factors carefully when designing porous polymers intended for specific uses.
Types of Porous Polymers
Porous polymers can be classified into various types, depending on their pore sizes: micro-, mesoporous, and macroporous. Each category presents unique characteristics and functionalities that make them suited for different applications.
Micro-, Mesoporous, and Macroporous
Micro-, mesoporous, and macroporous polymers serve distinct roles in various uses. Their classification primarily hinges on pore size:
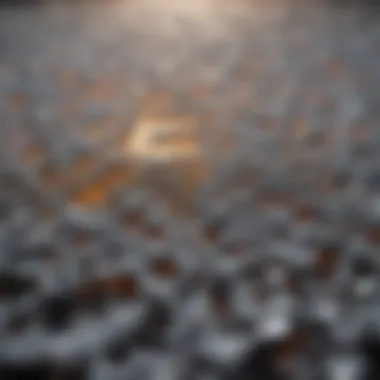
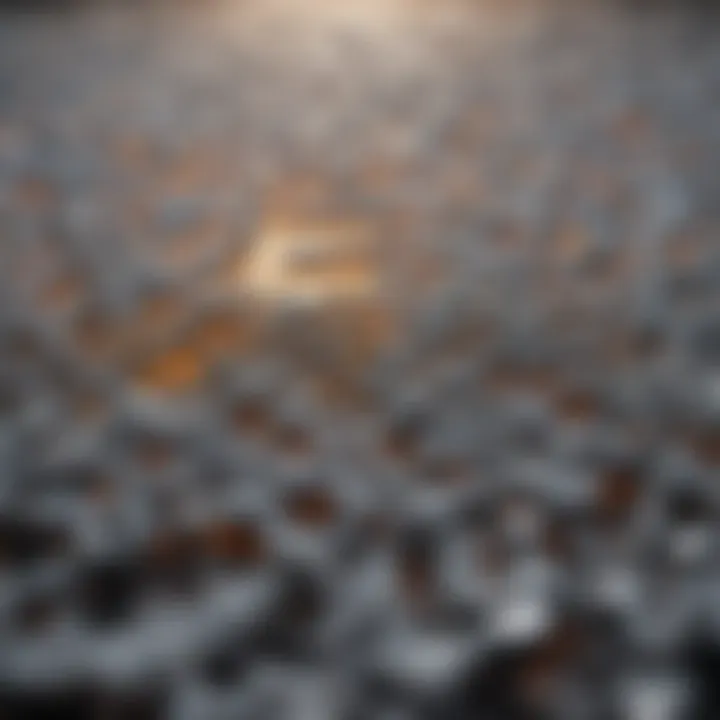
- Microporous polymers (pore size 2 nm) are hailed for their high surface area, making them excellent for gas adsorption and molecular sieving. They can trap tiny molecules, which is ideal for applications like filtration systems and gas storage. These polymers are often utilized in the creation of reusable catalysts due to their extensive surface interactions.
- Mesoporous polymers (pore size 2–50 nm) strike a balance between storage and permeability. Their structured architecture allows for better diffusion properties while still retaining a high surface area. This makes mesoporous materials particularly valuable in drug delivery systems where the controlled release of pharmaceuticals is essential. Furthermore, these structures are also popular in catalysis, providing a playground for reactions with larger reactant molecules.
- Macroporous polymers (pore size > 50 nm) are known for their ability to accommodate larger particles. They often find their place in applications that require fluid flow, such as in filtration devices or as scaffolding in tissue engineering. The larger pore sizes encourage better permeability and quicker responsiveness in applications like environmental remediation.
In summary, understanding these three types of porous polymers not only illustrates their key attributes but also showcases their significant roles in advancing technologies across different sectors. The tailored designs of these materials enable them to excel in diverse applications, addressing industry challenges while paving the way for innovative solutions.
Synthesis Techniques
The development and application of porous polymers cannot be discussed without closely examining the various synthesis techniques utilized to create these versatile materials. The method of synthesis not only influences the physical and chemical characteristics of the polymers but also their functionalities across different fields. Understanding these techniques is paramount for researchers and practitioners alike, as each method carries specific benefits, limitations, and application potential.
Solvent-Induced Techniques
Solvent-induced techniques stand out due to their relative simplicity and the advantageous conditions they offer for creating porous structures. This technique typically involves dissolving the polymer in a solvent, then inducing phase separation to form pores during the solidification process. The key here is the careful selection of both the polymer and solvent, as they play pivotal roles in determining the resulting porosity and mechanical strength.
- Advantages: The procedure offers a control over pore size and distribution by adjusting various parameters like the solvent type and temperature. It allows for the creation of materials that are not only porous but also highly functionalized.
- Considerations: However, one must also consider the environmental impact of solvents used, which can sometimes lead to toxic byproducts. Hence, a careful consideration of eco-friendliness is crucial, especially in today's push toward sustainable practices.
Template-based Approaches
Template-based approaches are another fascinating route for synthesizing porous polymers. In this method, a template—usually porous in itself—is created first, and then the polymer is deposited onto it. After curing, the template is removed, leaving behind a highly structured porous polymer. This technique offers a unique possibility of crafting specific pore structures, facilitating tailored microenvironments within the material.
- Benefits: The precision involved in this method allows for the creation of tunable pore architectures. As a result, template-derived polymers can cater to specific needs in fields such as drug delivery, where the release rates can be finely controlled.
- Drawbacks: On the flip side, the removal process can be complex and sometimes diminishes the structural integrity of the final product. Moreover, the choice of template significantly influences the outcome, presenting additional challenges.
Retro-Synthesis Methods
Retro-synthesis methods offer a more advanced route to creating porous polymers by focusing on building a structure from the molecular level upwards. This conceptual approach often utilizes self-assembly or other advanced techniques to create a precursor that can later be processed into a porous polymer.
- Importance: These methods allow for a high degree of customization, as they can incorporate various monomers and additives tailored to impart specific properties such as conductivity or biocompatibility.
- Challenges: However, the complexity of this process may lead to higher production costs and require extensive knowledge of polymer chemistry. Furthermore, monitoring the process closely is essential to ensure the desired outcomes.
"The choice of synthesis technique greatly influences the final properties of porous polymers, making it a critical decision for researchers engaged in material innovation."
Porosity and its Measurement
Porosity is a crucial feature when evaluating porous polymers, impacting their functionality in numerous applications. Understanding the measurement of porosity aids in revealing not just how much void space exists in a material, but also its effective surface area, which in turn plays a vital role in processes such as adsorption, filtration, and even drug delivery. In this section, we’ll explore various techniques to measure porosity, alongside how porosity affects the material properties of these polymers.
Porous Characterization Techniques
BET Surface Area Analysis
The Brunauer-Emmett-Teller (BET) method stands out as a robust technique for determining the surface area of porous materials. What makes this analysis essential is its ability to provide specific surface area measurements, which can indicate how much surface is available for interactions with surrounding substances. A key characteristic of BET analysis is its reliance on gas adsorption, typically using nitrogen at liquid nitrogen temperature. This characteristic allows for a detailed account of how much gas a sample can adsorb, giving insight into the material’s porosity.
This method is particularly beneficial because it is widely accepted and understood in both academic and industrial settings. The unique feature that sets it apart is its capacity to discern between micro-, meso-, and macroporous structures. However, while the BET method delivers valuable information about surface area, it does have its downsides, including potential inaccuracies for materials with very high porosity or those that might not adsorb gases in a manner conducive to accurate measurement.
Pore Size Distribution
Pore size distribution is another vital measurement in understanding porous polymers. This measurement outlines the range and frequency of pore sizes within a given material, thus offering an insight into how that material might perform under various conditions. The primary characteristic of this distribution is that it informs us about the accessibility of the pores. Accessibility is critical for applications such as catalytic processes or drug delivery systems, where pore size can dictate how effectively substances diffuse through the material.
The importance of this technique cannot be overstated, as it becomes a pivotal point for assessing practical applications of porous polymers. Its unique feature lies in its ability to reveal not just an average pore size but the overall distribution pattern, shedding light on whether pores are uniformly sized or if there is a wide variation. However, measuring pore size distribution can sometimes be challenging and may require using multiple analytical methods to attain accurate and comprehensive results.
Impact of Porosity on Material Properties
The impact of porosity on the material properties of porous polymers is profound. Increased porosity often results in lower density but may also lead to compromised mechanical strength. In contrast, controlled porosity can enhance specific mechanical properties while maintaining lightweight characteristics. The interplay of porosity with other material attributes—like permeability and diffusion rates—can demonstrate how well a material functions for its intended application. In essence, the balance of porosity can define a polymer’s efficiency in tasks such as gas separation, water filtration, or even its efficacy in drug delivery systems.
As porous polymers continue to evolve through innovations in measurement techniques and characterization methods, their functionalities in diverse fields will only grow, offering promising futures in environmental, biomedical, and energy storage solutions.
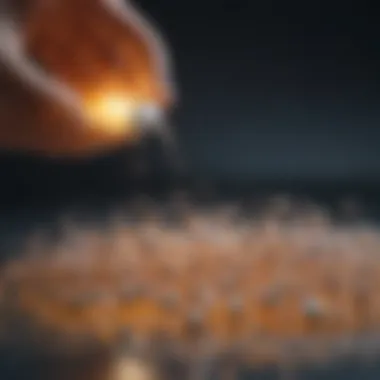
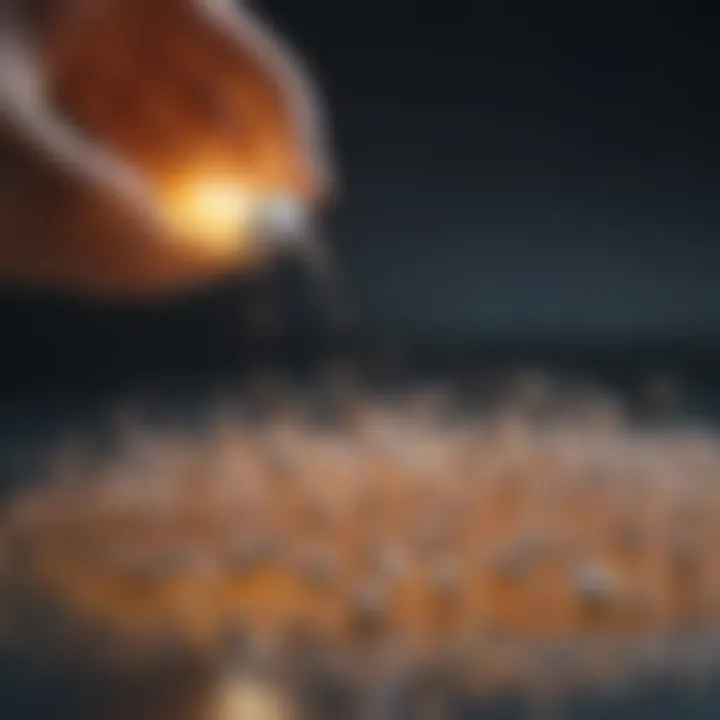
Applications in Diverse Industries
The versatility of porous polymers extends beyond a simple array of chemical compositions; it finds applications that push the boundaries of innovation across numerous sectors. These materials boast a unique ability to interact with various substances, enhancing their performance and opening avenues for new technologies. Understanding how porous polymers fit into diverse industries is crucial, not only for researchers and developers but also for anyone keen on the future of materials science.
Environmental Remediation
Pollutant Adsorption
Pollutant adsorption caters to the growing need for environmental protection. The standout characteristic of this process lies in its capacity to capture harmful toxins and chemicals from various environments, be it water or air. The porous structure of these materials allows them to trap pollutants like a sponge soaking up oil, facilitating a cleaner ecosystem. In discussions surrounding remediation, this method is often highlighted due to its efficiency and effectiveness.
One particularly interesting feature of pollutant adsorption is its adaptability; porous polymers can be tailored to target specific contaminants. However, one must recognize their limitations. The efficiency can vary based on the nature of the pollutant and environmental conditions, which makes them a good option, but not a one-size-fits-all solution. Ultimately, the role of pollutant adsorption in environmental remediation underscores the increasing importance of porous polymers in achieving sustainable solutions.
Water Treatment Systems
Water treatment systems incorporate porous polymers to enhance filtration techniques, making them invaluable in supplying clean drinking water. A key aspect of these systems is their ability to remove microscopic impurities and pathogens. The high surface area and tunable porosity of the polymers enable this efficiency. In a world grappling with water scarcity, their ability to purify water has positioned them as essential to modern treatment processes.
What makes these systems especially compelling is their low energy requirement compared to traditional methods. This is a significant advantage in regions where resources are limited. Nevertheless, there's a catch: maintaining the integrity and function of these polymer materials over prolonged use remains a challenge, which can lead to the need for replacement or regeneration. In summary, water treatment systems utilizing porous polymers enhance our capacity to deliver safe drinking water while presenting certain challenges that need addressing.
Biomedical Applications
Drug Delivery Systems
Drug delivery systems harness the unique attributes of porous polymers to create vehicles for controlled medicine release. This aspect is vital as it enables the precise administration of therapeutics, minimizing side effects while maximizing efficacy. The key characteristic lies in their customizable pore sizes, allowing them to encapsulate various drug molecules effectively.
The unique feature of drug delivery systems is their ability to trigger release under specific conditions—like pH or temperature changes. This targeted approach means that medications can be released more efficiently at their intended sites within the body. However, it’s vital to consider that the material's biocompatibility must be thoroughly assessed, or unintended reactions could occur, making this a double-edged sword. All in all, these systems exemplify the potential of porous polymers in revolutionizing how drugs are administered and absorbed in the body.
Tissue Engineering
Tissue engineering explores the idea of scaffolding, with porous polymers serving as a foundation for cell growth and tissue repair. This area is particularly exciting as it integrates biology and materials science, an emerging field that stands to redefine medical practices. The most notable characteristic is the ability of these polymers to mimic natural tissue properties, which is crucial for successful cell adhesion and proliferation.
The standout feature here is functionality; porous polymers can support the growth of various cell types and be engineered for specific biomedical applications. However, the challenge remains in the compatibility with human tissues over time, and scalability in production. Despite these hurdles, the role of porous polymers in tissue engineering offers a glimpse into a future where damaged tissues can be repaired or replaced more effectively.
Energy Storage Solutions
Battery Technology
The development of battery technology increasingly relies on the application of porous polymers, particularly in improving energy storage efficiency. A key characteristic is their contribution to ion conductivity, which is pivotal in enhancing battery performance. With a unique microstructure that facilitates ion movement, these polymers stand out as a promising option in the quest for more efficient batteries.
One appealing feature is their lightweight nature, which is crucial for applications in electric vehicles and portable devices. Nonetheless, challenges regarding stability under prolonged charge-discharge cycles exist, presenting a hurdle to widespread adoption. Nevertheless, they play a significant role in the race towards more sustainable energy solutions.
Photovoltaic Cells
Photovoltaic cells made with porous polymers are pushing the envelope in sustainable energy production. Their key characteristic is the capacity to maximize light absorption while minimizing material usage. This makes them highly efficient, especially when synthesized correctly, as the porous structure enhances electron mobility.
Furthermore, the unique aspect of these polymers lies in their adaptability to various substrates, enabling their use in flexible solar panels that can be integrated into everyday objects. However, the longevity of these cells remains under scrutiny, especially under harsh environmental conditions. In essence, photovoltaic cells utilizing porous polymers present exciting opportunities for a more energy-efficient future, despite needing solutions to durability concerns.
Challenges in Porous Polymer Development
The exploration of porous polymers provides a wealth of opportunities across various industries, but it is far from a walk in the park. Underlying stability and scaling issues present significant hurdles in the development of these materials. Understanding these challenges is imperative for researchers and practitioners aiming to harness the full potential of porous polymers. The intricate balance between performance, cost, and scalability is a maze that must be navigated carefully to achieve practical applications.
Stability and Durability Concerns
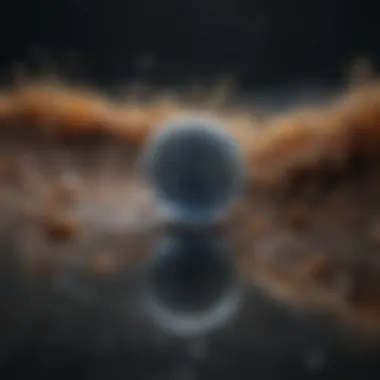
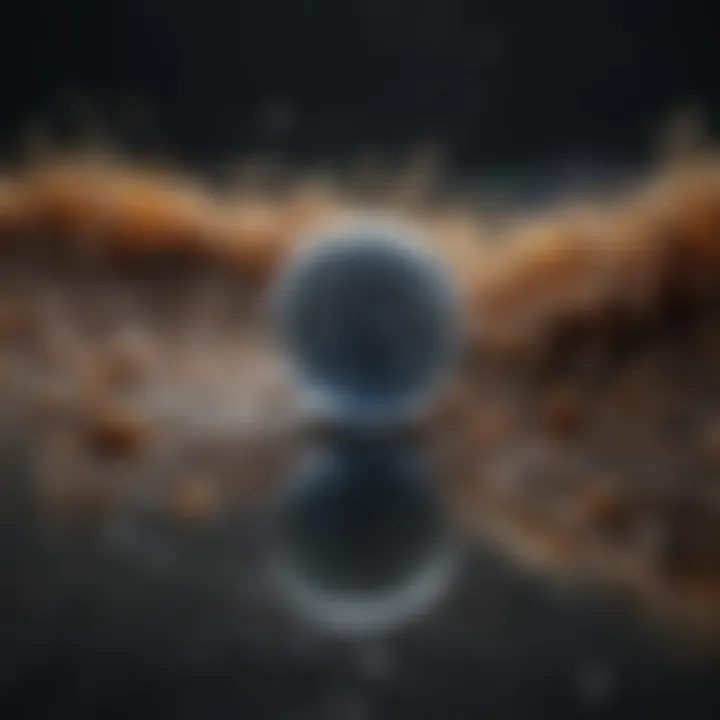
Stability in porous polymers is a vital concern that can make or break their functionality. When subjected to environmental stressors or physical wear, these materials must retain their structural integrity. For instance, in water treatment systems, a porous polymer may become compromised by organic pollutants or high-pressure conditions. If it can't withstand these challenges, the effectiveness in filtering contaminants diminishes quickly.
Moreover, the durability of porous polymers is crucial in biomedical applications like drug delivery systems. A material that degrades too rapidly can lead to premature medication release, which diminishes therapeutic efficacy. Researchers often grapple with the trade-off between material flexibility and rigidity, as both aspects play a role in the longevity and performance of the polymers.
"A polymer that doesn’t last is like a clock without hands; it doesn’t serve its purpose."
It's also important to consider that stability is closely tied to the intrinsic properties of the raw materials used in porous polymer synthesis. For example, the choice between natural and synthetic polymer bases can drastically influence the end product's resilience. By narrowing down the material options and conducting rigorous testing under varying conditions, developers can better identify suitable candidates for robust porous polymers.
Production Scalability Issues
Scaling production of porous polymers is akin to trying to fit a square peg in a round hole; it sounds simpler than it actually is. While the initial synthesis of these materials may be promising, transitioning to large-scale manufacturing introduces a slew of technical challenges. The methods effective at a smaller scale can often become unfeasible when trying to increase output, inevitably causing costly bottlenecks.
For instance, template-based approaches might yield high-quality porous structures in lab settings, but replicating that quality on a commercial scale can be problematic. Factors like uniformity and consistency in pore size often take a hit as production volumes increase.
Additionally, regulatory compliance adds another layer of complexity. Each industry has its own set of standards and requirements that manufacturers must navigate, further complicating the scalability of porous polymer production. Companies might invest heavily in research and development only to find out they are hitting walls when trying to mass produce a polymer that meets regulatory benchmarks.
Future Trends and Innovations
The landscape of porous polymers is evolving rapidly, and the exploration of future trends within this field is fundamental to understanding how these materials will shape our world. As researchers push the boundaries of material science, innovations emerge that promise to enhance functionality and broaden applications. Key aspects of this forward-looking perspective include advancements in synthesis techniques, tailored material properties for specific applications, and the integration of porous polymers into novel technologies.
Advancements in Material Science
In the realm of material science, porous polymers are at the forefront of cutting-edge research. Innovations are driven by a need to create materials that are not just effective but also highly customizable to fit various needs. New synthesis methods are being developed that allow for the construction of polymers with precise control over pore size and distribution. For instance, using electrospinning to create fibrous structures can lead to enhanced surface area, which is beneficial for applications in filtration and adsorption.
Moreover, "green" chemistry principles are being increasingly adopted in polymer production, thus minimizing environmental impact. This shift not only improves sustainability but also enhances the safety profile of porous polymers used in sensitive environments, such as biomedical applications.
Importantly, machine learning is stepping into the picture, aiding researchers in predicting the behavior of complex materials. By analyzing large datasets, scientists are finding trends that allow for the optimization of polymer properties, leading to stronger and more resilient materials that can endure varying conditions without compromising their functions.
Biocompatible Porous Polymers
The development of biocompatible porous polymers holds transformative potential, especially in the medical field. As the demand for advanced drug delivery systems and tissue engineering scaffolds grows, the creation of polymers that can seamlessly integrate into biological systems becomes paramount. These materials must not only be compatible with human tissues but also provide optimal structures for cell growth and nutrient exchange.
Recent advances have paved the way for polymers made from natural materials, such as chitosan or collagen, which boast excellent biocompatibility. This makes them ideal candidates for various biomedical applications, from sutures to sophisticated drug delivery mechanisms.
A key advantage of biocompatible porous polymers is their ability to facilitate controlled release of therapeutic agents. This attribute makes them highly effective in minimizing side effects while maximizing treatment efficacy. With ongoing research, the refinement of these polymers is likely to open new avenues in regenerative medicine and personalized therapies.
"The future of porous polymers is not just about functionality but also about improving lives through innovation and sustainability."
As we look ahead, the implications of both advancements in material science and the development of biocompatible polymers cannot be overstated. The potential applications stretch across myriad fields, promising to address some of humanity's most pressing challenges.
End
In wrapping up our exploration into the realm of porous polymers, it’s essential to reflect on their multi-faceted significance across various fields. Porous polymers stand out not just for their unique properties but also for the fundamental benefits they offer in practical applications. These materials have carved a niche in industries such as environmental science, healthcare, and energy solutions.
Summarizing the Importance of Porous Polymers
Porous polymers are more than just a scientific curiosity; they embody the intersection of innovation and necessity. These materials possess remarkably high surface area and customizable pore structures, which makes them valuable in filtration and adsorption processes. For instance, in environmental remediation, their capability to trap pollutants underscores their role in sustaining our ecosystems. They act like a sponge, absorbing unwanted substances and ensuring cleaner environments.
Furthermore, in the biomedical field, the ability of porous polymers to facilitate controlled drug release is changing the way medical professionals approach treatment plans. The adaptability of these materials makes them suitable for various drug delivery systems, offering targeted therapies and enhanced patient outcomes. This has implications for everything from cancer treatment to administering vaccines efficiently.
Additionally, the energy sector greatly benefits from porous polymers. Their use in battery technology plays a pivotal role in improving energy storage capabilities, which is crucial as we transition toward renewable energy sources. They also show promise in enhancing the efficiency of photovoltaic cells, pushing the boundaries of how we harness solar energy.
But it's not all smooth sailing. While porous polymers bring numerous advantages, challenges remain. Concerns regarding their stability and scalability during production must be addressed. It’s crucial for researchers and manufacturers to ensure that the advantages provided by these materials do not come at the cost of reliability or environmental sustainability.
"Through conscientious development and application, the true power of porous polymers can be fully realized, impacting the world for the better."
The journey of understanding porous polymers is just starting. Keeping an eye on future breakthroughs will be vital for anyone engaged in related fields. Students, researchers, and industry professionals alike will benefit from this ongoing conversation about the versatility of these fascinating materials.