Understanding UV-Visible Spectrometry in Science
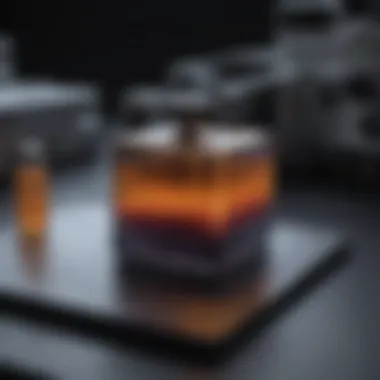
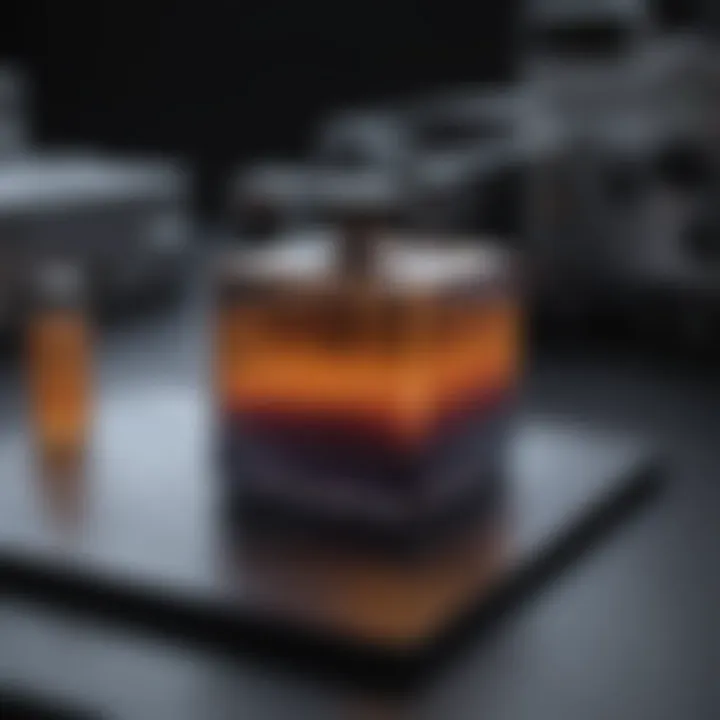
Intro
UV-visible spectrometry, a technique rooted in the principles of light absorption, represents a cornerstone in analytical chemistry and various scientific domains. By understanding the spectrum of ultraviolet and visible light, researchers can derive valuable insights into the composition and behavior of substances ranging from complex biological molecules to simple synthetic compounds. This method functions by measuring the absorbance of light as it passes through a sample, allowing scientists to determine the concentration of analytes, identify compounds, and examine reaction kinetics.
The operational mechanics of UV-visible spectrometry involve a combination of light sources, monochromators, sample cuvettes, detectors, and data analysis systems. Each component plays a vital role in ensuring the accuracy and reliability of the measurements. Throughout this article, we will uncover the intricate mechanisms at play, discuss the significant applications across diverse fields such as chemistry, biology, and material science, and explore the advancements that have emerged in recent years.
As we delve deeper, the goal is to equip readers with a thorough knowledge that not only highlights the practical aspects of UV-visible spectrometry but also sheds light on its theoretical foundations. The implications of this technique are far-reaching, influencing both academic research and industrial applications. With innovations continuously reshaping its use, understanding UV-visible spectrometry is essential for anyone involved in scientific inquiry.
As we move forward into the article, the subsequent sections will elaborate on the key findings, implications of the research, and future directions within this fascinating field.
Prolusion to UV-Visible Spectrometry
UV-visible spectrometry serves as a keystone in the analytical sciences, bridging essential concepts in physics, chemistry, and biology. This technique allows for the examination of light absorption by matter, particularly in the ultraviolet and visible regions of the electromagnetic spectrum. Through this understanding, researchers and students gain insights into molecular interactions and concentrations, benefitting areas like drug development, environmental monitoring, and biochemical analysis.
Definition and Importance
UV-visible spectrometry, often abbreviated as UV-Vis spectrometry, refers to the quantitative measurement of light absorption in the ultraviolet and visible spectral regions, spanning wavelengths from approximately 200 nm to 800 nm. The technique is crucial for determining the concentration of solutes in solution, as it relies on the principle that different compounds absorb light at specific wavelengths.
Benefits associated with UV-Vis spectrometry include:
- Simplicity: The method has straightforward operational principles, making it accessible for various laboratories.
- Rapid Analysis: It allows for quick results, which is essential for high-throughput analysis in research scenarios.
- Wide Applicability: The technique finds use in numerous fields, including pharmaceuticals, environmental science, and food safety.
The importance of UV-Vis spectrometry cannot be overstated. It serves not only for identification purposes but also for quantification in various studies. Many industries employ this technique for routine quality control and compliance verification. Its dependable results help in making informed decisions based on accurate data.
Historical Development
The journey of UV-visible spectrometry is rooted in the early explorations of light and its interactions with matter. Although spectroscopic techniques appear to be a modern development, their origins date back centuries. The initial forays into determining wavelength and its implications set the stage for adopting UV-Vis techniques.
In the early 19th century, notable scientists like William Herschel discovered infrared radiation, which laid groundwork for later developments in spectroscopy. Innovations continued through the years, leading to the production of the first commercial spectrophotometers in the early 20th century. As technology advanced, spectrometers became more sophisticated, integrating better light sources and detectors.
The latter part of the 20th century saw exponential growth in instrumentation capabilities, allowing researchers to conduct more complex analyses with enhanced sensitivity and accuracy. Today, UV-visible spectrometry is integral to educational programs and research presentations, reflecting its vital role in the broader context of scientific inquiry. The continued advancements in this field resonate through modern applications, marking its evolution as a foundational tool for contemporary research.
Fundamental Principles
The fundamental principles of UV-visible spectrometry form the foundation that underpins this analytical technique. These principles are vital for understanding how spectrometry works and why it is essential in various scientific fields. A solid grasp of these concepts can significantly enhance the effectiveness of analyses conducted in research and industry. This section will explore the basic concepts of light absorption, the Beer-Lambert Law, as well as the spectral range and wavelength relevant to this method.
Basic Concepts of Light Absorption
Light absorption is a key aspect of UV-visible spectrometry. When light interacts with a sample, specific wavelengths may be absorbed, while others are transmitted. The ability of a sample to absorb light is primarily dependent on its electronic structure and the chemical bonds present. Molecules can absorb UV or visible light to promote electrons from a lower energy state to a higher one. This electronic transition is what provides valuable information about the sample.
Absorption can be expressed in various forms. For example, chromophores are specific parts of molecules that absorb light. The presence of chromophores determines how a substance interacts with light. When evaluating the absorption spectrum, one can identify the wavelengths at which a sample absorbs, thus allowing for qualitative analysis of the substances in the sample.
Beer-Lambert Law
The Beer-Lambert Law is a fundamental equation used in UV-visible spectrometry. It relates the absorption of light to the properties of the material through which the light is traveling. The law can be expressed mathematically as:
Where:
- A is the absorbance (no units)
- ε is the molar absorptivity (L/(mol*cm))
- c is the concentration of the solution (mol/L)
- l is the path length of the sample (cm)
This relationship allows researchers to determine the concentration of an absorbing species in a mixture by measuring the absorbance. It is essential for conducting quantitative analyses. However, certain assumptions apply, such as that the system must be under ideal conditions and that absorbance is linear with respect to concentration.
Spectral Range and Wavelength
The spectral range refers to the spectrum of electromagnetic radiation that can be measured using UV-visible spectrometry. Typically, this spans the ultraviolet (approximately 200 to 400 nm) and visible (approximately 400 to 700 nm) ranges. The selection of wavelengths is critical since different substances have distinct absorption profiles over this range. By understanding the different wavelengths, one can tailor their experiments to achieve optimal results.
Moreover, different applications may require focusing on specific wavelengths to identify or quantify substances accurately. For example, nucleic acids have strong absorption at 260 nm, while proteins generally absorb around 280 nm. Knowing these specifics enables researchers and analysts to design better experiments and obtain reliable results.
Understanding the fundamental principles of light absorption and the Beer-Lambert Law is crucial for accurate interpretation of UV-visible spectrometry data.
Instrumentation
Instrumentation is a crucial aspect when it comes to UV-visible spectrometry. It provides the necessary components that make the measurement and analysis of light absorption possible. Understanding the individual parts that compose a spectrometer will help in grasping how they contribute to the overall effectiveness of UV-visible spectrometry.
Core Components of UV-Visible Spectrometers
Light Source
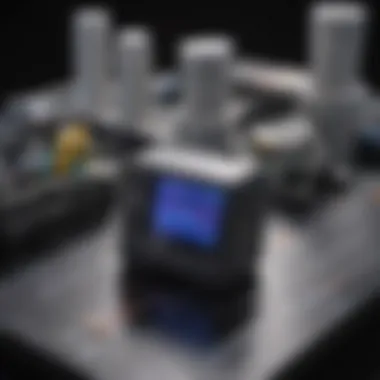
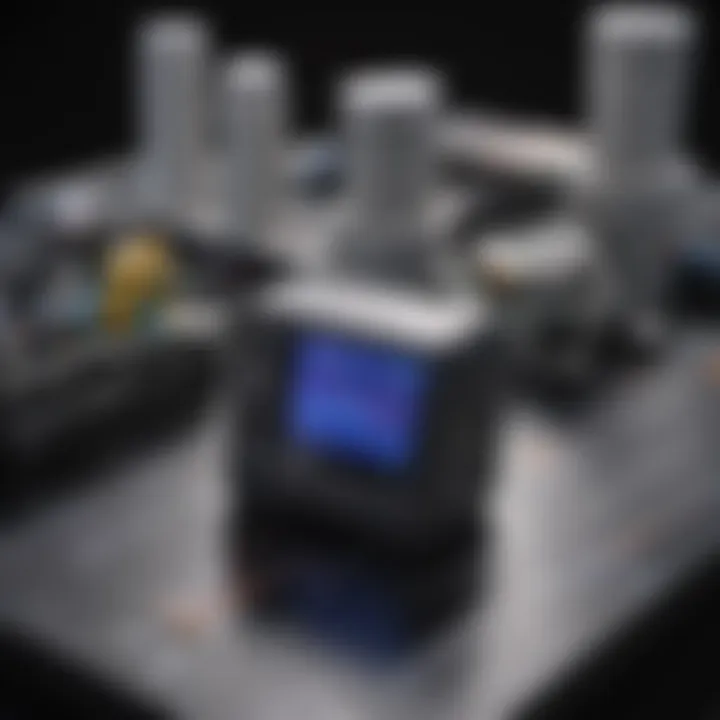
The light source in UV-visible spectrometers is responsible for generating the light that illuminates the sample. Commonly used light sources include deuterium lamps for the UV range and tungsten halogen lamps for the visible range. The key characteristic of these light sources is their ability to provide a continuous spectrum of light, which is essential for obtaining accurate absorbance readings. This makes them a beneficial choice for precise measurements across different wavelengths.
One unique feature of a deuterium lamp is its high intensity in the UV region, which allows for sensitive detection of samples that absorb in that range. However, these lamps have a limited operational lifespan and can require frequent replacements, which could be a disadvantage in a high-throughput environment.
Monochromator
The monochromator is a vital component that selects specific wavelengths of light to be transmitted to the detector after passing through the sample. This selection process is critical for analyzing how much light is absorbed at defined wavelengths. The major advantage of a monochromator is its flexibility in analyzing various compounds, making it quite popular in research laboratories.
A notable feature of modern monochromators is their use of diffraction gratings, which provide higher resolution and thus better discrimination between closely spaced wavelengths. However, they tend to be more complex and may increase the overall cost of the spectrometer.
Detector
The detector's role is to measure the intensity of light after it has interacted with the sample. Common detector types include photomultiplier tubes and photodiodes, each excelling in different spectral regions. The key characteristic for a successful detector is sensitivity, as it directly impacts the instrument's ability to detect low concentrations of analytes.
Photomultiplier tubes are known for their extreme sensitivity and fast response time, making them highly effective in many applications. On the other hand, photodiodes are more rugged and easier to integrate into compact devices but may have lower sensitivity in certain regions. Choosing the right detector often depends on the kind of analysis being performed.
Types of Spectrometers
Single Beam Spectrometers
Single beam spectrometers measure the intensity of light before and after it passes through the sample using a single light path. This simplicity makes them relatively lightweight and easier to operate. However, they can be less precise in environments where the baseline may change, as they have no reference beam for noise correction.
Their key characteristic is straightforwardness in design, which translates into lower costs and ease of use. Nonetheless, they are not as favored when high precision and accuracy are required.
Double Beam Spectrometers
Double beam spectrometers utilize two light paths, one for the sample and another for a reference. This design enables real-time comparison of the sample and reference light intensities, significantly reducing the influence of fluctuations in the light source. This is a major benefit in ensuring accurate results.
They are well-known for their excellent stability and high precision, which makes them the preferred choice for quantitative analysis in many labs. However, the complexity of their structure can lead to increased maintenance costs.
Scanning Spectrometers
Scanning spectrometers move the monochromator across a range of wavelengths, allowing comprehensive scanning of the spectrum. This capability is particularly valuable when analyzing complex samples with unknown absorption characteristics. They are especially beneficial in research and development settings.
A unique feature of scanning spectrometers is their ability to gather a wide array of data in one run, which enhances efficiency. However, the scanning process may lead to longer analysis times, which is a consideration when rapid analysis is required.
In summary, instrumentation in UV-visible spectrometry plays a pivotal role in determining accuracy, reliability, and overall performance of the analysis. Understanding each component's strength and limitations aids in selecting the appropriate spectrometer for specific applications.
Analytical Techniques
Analytical techniques play a vital role in UV-visible spectrometry, enabling researchers and professionals to draw meaningful conclusions from data obtained during experiments. These techniques allow for both qualitative and quantitative assessments, providing insights that are crucial in various fields. Understanding these methods is essential for effective application in real-world scenarios, facilitating precise measurements and identification of compounds.
Quantitative Analysis
Quantitative analysis involves determining the amount of a substance in a sample. This part of analytical techniques is fundamental because it provides quantifiable data that can be applied in numerous scientific and practical applications.
Calibration Curves
Calibration curves are graphs used to correlate the concentration of a substance to its absorbance. This method is critical in quantitative analysis as it offers a visual representation of how absorbance changes with concentration. The key characteristic of calibration curves is their ability to provide a linear correlation, which enhances the reliability of quantification.
The uniqueness of calibration curves lies in their effectiveness in various applications, especially when interpreting results in research settings. They are widely adopted because they enable researchers to predict the concentration of unknown samples based on the established relationship. One advantage is that they can result in high accuracy, but they also require precise preparation of standards to avoid systematic errors.
Standard Addition Method
The standard addition method involves adding known quantities of a substance to a sample to account for matrix effects. This technique is particularly effective when analyzing samples from complex matrices, as it ensures accuracy in the results obtained. The essential characteristic of this method is its adaptability, allowing researchers to analyze a wide range of samples without extensive sample preparation.
The unique feature of the standard addition method is its ability to improve measurement reliability in complicated scenarios. A major advantage is that it mitigates issues related to interference from other components present in the sample. However, careful calibration and quantitative calculations are still required to achieve accurate results.
Qualitative Analysis
Qualitative analysis focuses on identifying the components of a sample rather than quantifying them. Understanding qualitative analysis is paramount as it allows scientists to establish what substances are present in a mixture, aiding in scientific discovery and application.
Identifying Compounds
Identifying compounds using UV-visible spectrometry involves analyzing the absorption spectra of samples to determine their composition. This method is effective for its straightforward approach in recognizing compounds based on specific absorption patterns. The key characteristic is the ability to identify a wide range of organic and inorganic substances, making it a versatile method within analytical chemistry.
The uniqueness of identifying compounds lies in its effectiveness at differentiating closely related substances based on their UV-Vis fingerprints. This capability is particularly beneficial in research and quality control laboratories where precise identification is critical. Nevertheless, it does have limitations, particularly when samples exhibit overlapping spectra, complicating the identification process.
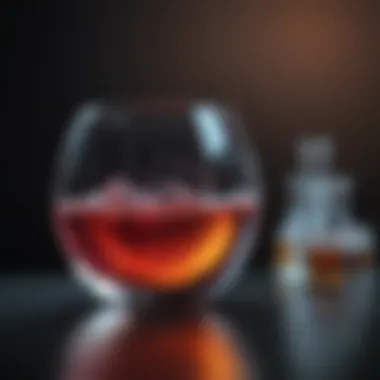
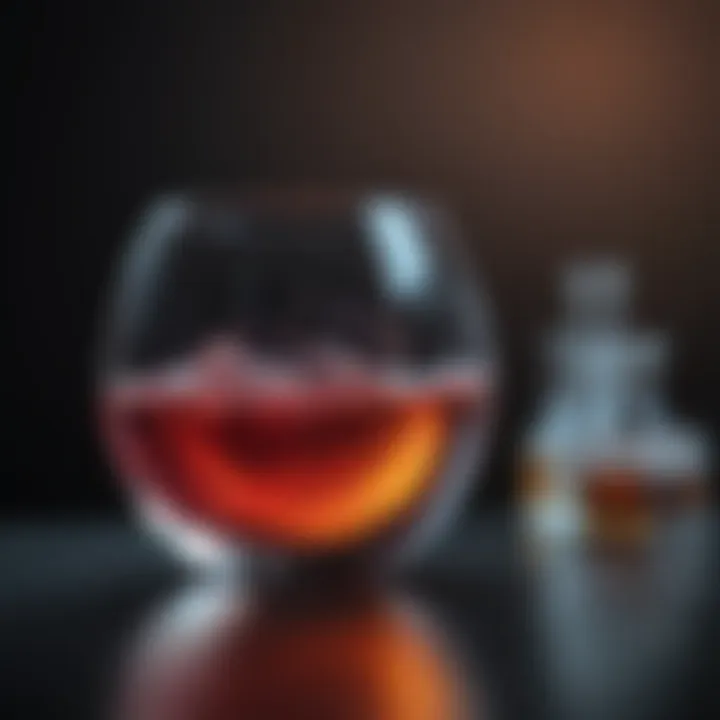
Analyzing Mixtures
Analyzing mixtures involves determining the individual components within a complex sample. This is a crucial aspect of qualitative analysis, especially in environmental and biological sciences where samples often contain multiple substances. The key characteristic of this analysis is its ability to provide insights into the composition of mixtures, which is essential for understanding chemical behavior in different environments.
The distinctive feature of analyzing mixtures is its applicability in real-world scenarios, such as pharmaceutical development or environmental monitoring. Its advantages include the potential to provide comprehensive data about sample composition, revealing vital information about interactions between components. However, this method may face challenges regarding instrument sensitivity and the presence of interfering substances, necessitating careful consideration during analysis.
Applications of UV-Visible Spectrometry
UV-visible spectrometry has a profound impact across various fields. It serves as a cornerstone in analytical chemistry, biological research, and environmental studies. This technique enables precise measurement of light absorbance and helps in identifying various substances based on their optical characteristics. Its application is vital for conducting research and quality control in multiple domains. Thus, understanding these applications can significantly benefit scientists and researchers.
In Chemistry
Analysis of Solutions
The analysis of solutions using UV-visible spectrometry is essential for determining the concentration of specific chemical species. This method leverages the Beer-Lambert law, which states that absorbance is directly proportional to concentration. The key characteristic of this analysis is its sensitivity; even low concentrations of a substance can be detected. This makes it a popular choice in both academia and industry.
In this context, the unique feature is the ability to quickly obtain data without extensive sample preparation. Although it is generally a fast and effective choice, one drawback lies in the potential for matrix effects, which can skew results if not carefully managed.
Reaction Kinetics
Examining reaction kinetics through UV-visible spectrometry offers insights into the rate and mechanism of chemical reactions. By measuring absorbance changes over time, researchers can derive important information about reaction rates. A key characteristic of this approach is its real-time data collection, allowing for the understanding of dynamic processes.
This method is beneficial because it provides continuous monitoring, an advantage over traditional batch analysis. However, determining the concentration of intermediate species can be challenging, which may complicate kinetic interpretations.
In Biology
Nucleic Acid Quantification
Nucleic acid quantification is another significant application of UV-visible spectrometry. Researchers frequently use it to measure DNA and RNA concentration. The key aspect of this analysis is its ability to provide quick quantification using minimal sample volume. It’s a favored technique in molecular biology labs for quantifying nucleic acids before further analysis, such as sequencing.
A unique feature of this technique is its specificity; distinct absorbance peaks correspond to nucleic acids, thus ensuring accurate results. However, it is crucial to consider the presence of other contaminants that may absorb at similar wavelengths, potentially leading to inaccuracies.
Protein Analysis
Protein analysis utilizing UV-visible spectrometry allows for the quantification and characterization of protein samples. This approach typically exploits the absorbance of aromatic amino acids at specific wavelengths. The key characteristic here lies in its non-destructive nature, making it a valuable choice for various applications.
This technique can efficiently provide information on protein concentration and purity. Nonetheless, it is essential to note that proteins lacking aromatic residues may not be accurately measured, limiting its application for certain samples.
In Environmental Science
Water Quality Assessment
Water quality assessment is a critical application of UV-visible spectrometry. By measuring the absorbance of various contaminants, researchers can gauge the presence of pollutants in water bodies. A significant characteristic of this method is its ability to analyze multiple parameters simultaneously, enhancing efficiency.
Moreover, it can detect a wide range of substances, including organic and inorganic compounds. However, variability in water composition can affect the accuracy of readings, requiring careful calibration and method validation.
Pollution Monitoring
Pollution monitoring through UV-visible spectrometry is crucial for environmental studies and regulatory compliance. By tracking absorbance changes, scientists can evaluate the levels of pollutants in different ecosystems. The key aspect of this application is its real-time monitoring capabilities, which facilitate timely decision-making.
This method's unique feature is its adaptability to various environmental conditions. However, factors such as light scattering and matrix effects may compromise the accuracy of data, making it essential to use appropriate sampling techniques and controls.
Limitations and Challenges
Understanding the limitations and challenges of UV-visible spectrometry is essential for a comprehensive grasp of its reliability and application across various scientific fields. While this analytical technique serves many purposes, its effectiveness can be hindered by several factors. Acknowledging these limitations ensures that researchers use this method appropriately and develop strategies to mitigate challenges that may arise.
Interference and Background Noise
Interference and background noise are significant challenges in UV-visible spectrometry. Factors such as stray light, temperature fluctuations, and optical scatter can distort measurements. These external variables can introduce errors in absorbance readings, leading to inaccurate quantitative and qualitative analyses. For example, the presence of impurities in a sample can absorb light at similar wavelengths as the analyte, resulting in misleading results.
Moreover, background noise arises from multiple sources, including electronic noise from the detector and environmental factors. Researchers often use blank samples to determine baseline noise levels. By subtracting this background information, they can improve measurement accuracy. However, relying solely on this correction method has its limits.
"Understanding background noise and how to manage it is vital for accurate UV-visible spectrophotometric analysis."
To minimize interference, it is crucial to maintain instrument calibration and implement proper sampling techniques. Additionally, selecting wavelengths where the analyte has a distinct absorption peak can help differentiate it from potential interferents.
Sample Preparation Issues
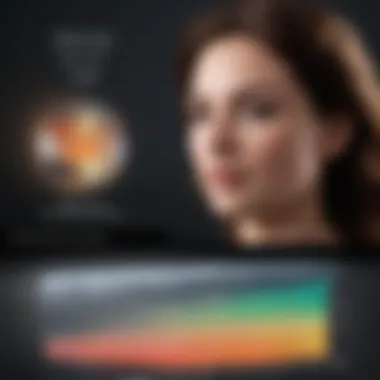
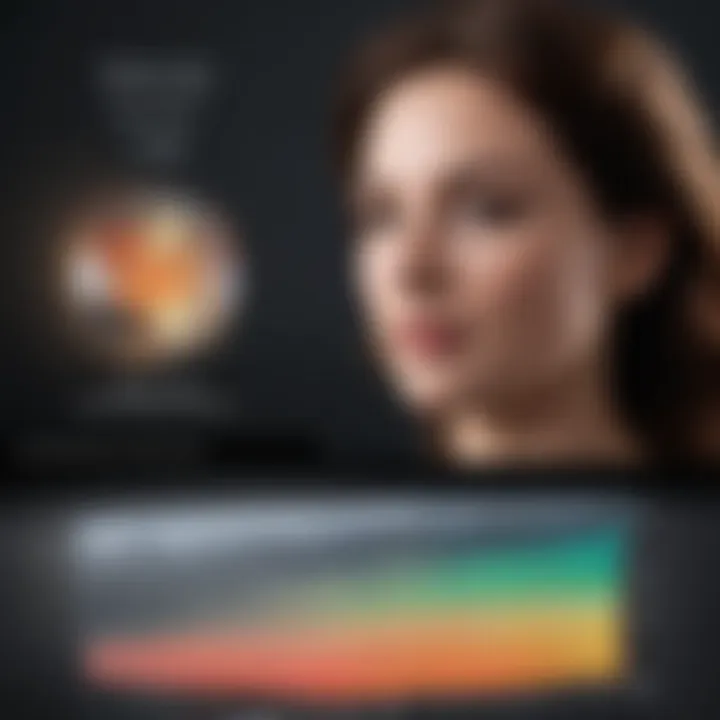
Sample preparation is another crucial aspect that directly influences the outcomes of UV-visible spectrometry. If samples are not prepared correctly, the validity of results can be compromised. Factors such as sample concentration, solvent compatibility, and dust contamination can impact absorbance readings.
For accurate analysis, using the right solvent is essential. Some solvents might exhibit absorbance in the UV-visible range, which can obscure the signals from the analytes of interest. In such cases, alternative solvents need to be considered. Further, concentrations must be appropriately managed, as excessively high concentrations can lead to deviations from the Beer-Lambert Law—resulting in non-linear responses.
Another common sample preparation issue is ensuring that the samples are free from particulate matter. Dust or bubbles can scatter light and introduce errors in measured absorbance. Adequate filtration or centrifugation can help achieve sample clarity.
In summary, recognizing limitations in interference, background noise, and sample preparation is critical for optimizing the use of UV-visible spectrometry. Addressing these challenges enables researchers to derive more accurate and reliable results, ultimately enhancing the technique’s application across diverse scientific disciplines.
Recent Advancements
Recent advancements in UV-Visible spectrometry signify a transformative phase in analytical chemistry, responding to the rising demands of precision and versatility in research. The integration of new technologies and methodologies has enhanced the effectiveness of this technique. Understanding these advancements is critical for professionals and students engaged in scientific exploration. They emphasize the continuous evolution of UV-Visible spectrometry, addressing both traditional limitations and emerging applications.
Technological Innovations
The field of UV-Visible spectrometry has witnessed significant technological innovations that enhance data quality and instrument performance. One notable development is the introduction of photodiode array (PDA) detectors. These detectors allow for simultaneous acquisition of an entire spectrum, greatly increasing throughput and allowing for rapid analysis. This ability reduces data collection times and minimizes errors associated with sample handling.
Moreover, advances in light sources have improved the stability and intensity of light emitted, which directly impacts the sensitivity and accuracy of measurements. LEDs have emerged as popular choices due to their long lifespan and limited heat generation. This innovation is crucial for maintaining the integrity of temperature-sensitive samples during analysis.
Another promising area is miniaturization. Handheld UV-Visible spectrometers are now capable of providing high-quality data while being portable, facilitating field analyses that were once impossible or impractical. This portability extends the reach of spectrometry into various environmental and field-based applications.
Integration with Software
The integration of software with spectrometry systems has significantly enhanced data analysis capabilities. Advanced software programs aid in processing complex data sets, automating routine analyses, and improving the overall user experience. Applications now allow researchers to generate customizable reports, visualize spectra, and apply sophisticated algorithms to extract meaningful results.
Several platforms leverage machine learning algorithms to enhance predictive models. These advancements enable better identification of compounds and improve quantitative results, particularly when dealing with complex mixtures in biological and chemical analyses.
Furthermore, cloud-based data storage solutions allow for secure sharing and analyzing large datasets. Researchers can collaborate more efficiently, even across long distances, facilitating a global approach to problem-solving. The easy access to data promotes transparency and reproducibility in scientific investigations, which are essential in today’s research environment.
The integration and innovation in UV-Visible spectrometry not only enhance the existing capabilities but also pave the way for entirely new applications, further expanding its utility in varied fields.
Future Trends
The field of UV-visible spectrometry is continuously evolving. Future trends in this area are crucial as they reflect not only advancements in technology but also a shifting landscape in research and application. Understanding these trends is essential for researchers, educators, and professionals who rely on spectrometric techniques to inform their work. Adaptation to emerging trends ensures efficient and accurate analysis, fostering innovation across various scientific domains.
Emerging Research Applications
Recent developments have opened new avenues for using UV-visible spectrometry. One prominent area is in the analysis of complex biological systems. Researchers are devising novel applications that harness spectrometric techniques to study cellular environments, drug interactions, and metabolic pathways. For example, UV-visible spectrometry is being employed to monitor the dynamics of enzyme-catalyzed reactions in real-time, providing insights into reaction mechanisms.
Another promising application is the use of UV-visible spectrometry in nanotechnology. Characterizing materials at the nanoscale presents challenges, but this technique allows for precise analysis of nanomaterials' optical properties. This includes studying surface plasmon resonances in metallic nanoparticles, which have implications for sensors and imaging technologies.
"Emerging applications in research continue to expand the utility of UV-visible spectrometry, offering tools for deeper analysis of complex phenomena."
Moreover, integration with other analytical methods, such as mass spectrometry or chromatography, is shaping the future landscape of UV-visible techniques. This multidisciplinary approach enhances the ability to resolve intricate mixtures and provides comprehensive data for better decision-making in fields like environmental science, pharmaceuticals, and materials science.
Sustainability and Environmental Considerations
In today's context, sustainability is a critical factor guiding future trends in UV-visible spectrometry. Environmental considerations are influencing the design of spectrometers, including the use of eco-friendly materials and energy-efficient light sources. Researchers are increasingly focusing on minimizing the environmental impact of their analytical processes.
One significant trend is the push towards using less toxic solvents in sample preparation. Traditional solvents can pose hazards; thus, alternatives that are less harmful are gaining traction. This aligns with broader environmental goals and regulations aimed at reducing hazardous waste in laboratories.
Furthermore, UV-visible spectrometry is being positioned as an essential tool for monitoring environmental pollutants. Researchers harness its capabilities for assessing water and air quality, enabling real-time monitoring of contaminants. This usage not only aids in research but also supports compliance with environmental regulations and helps industries manage their ecological impact.
Overall, as UV-visible spectrometry adapts to emerging research demands and addresses sustainability challenges, its role in science and industry solidifies. Researchers not only look to improve existing methods but also consider the broader implications of their work, aligning with global efforts toward sustainability.
Finale
The conclusion serves as a vital component of the article, synthesizing the various aspects of UV-visible spectrometry discussed. Its significance lies in its ability to encapsulate the essence of the preceding discussions, offering the reader a cohesive understanding of this analytical technique. By summarizing the fundamental principles, instrumentation specifics, and a broad spectrum of applications, the conclusion ensures that key points are not just highlighted but are also clearly connected to their implications in scientific research.
In reviewing the content, several specific elements come to light. First, the importance of understanding both quantitative and qualitative analytical techniques is critical. This understanding allows researchers to effectively employ UV-visible spectrometry across disciplines, ensuring accurate results and insights. Furthermore, discussing limitations and challenges reinforces the necessity for meticulous sample preparation and consideration of potential interferences. Such awareness empowers professionals to address these hurdles proactively, enhancing the reliability of their analyses.
Summary of Key Points
Several key points emerge from the discussion on UV-visible spectrometry:
- Foundational Knowledge: A clear grasp of how light absorption works is paramount for utilizing the method.
- Instrumentation Insight: Knowledge of core components like the light source, monochromator, and detector ensures proper equipment selection and maintenance.
- Analytical Versatility: The technique's application spans across chemistry, biology, and environmental science, proving its versatility.
- Emerging Trends: Acknowledgment of recent technological advancements positions researchers to leverage modern developments in their inquiries.
These points underscore the significance of UV-visible spectrometry in various fields. A strong foundation in these aspects enables effective utilization of the technique, fostering further exploration and discovery.
Emphasis on Continued Research
Looking ahead, the emphasis on continued research in UV-visible spectrometry is crucial. As scientific inquiry evolves, so too does the need for refined methodologies and innovative applications. Researchers should focus on addressing current limitations, such as interference and noise, through rigorous studies and technological improvement.
Moreover, exploring emerging research applications could open new avenues for UV-visible spectrometry. For instance, integrating real-time environmental monitoring and advancing chemical analysis can reveal insights into pressing global challenges. This not only enhances the relevance of UV-visible spectrometry but also solidifies its place in future scientific discussions.