Understanding Semiconductor Temperature Sensors
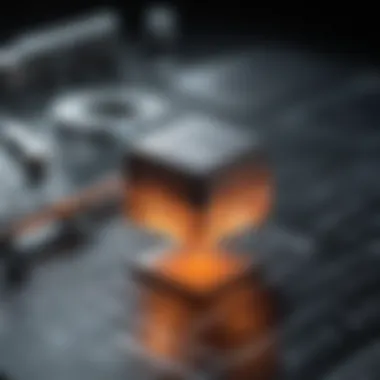
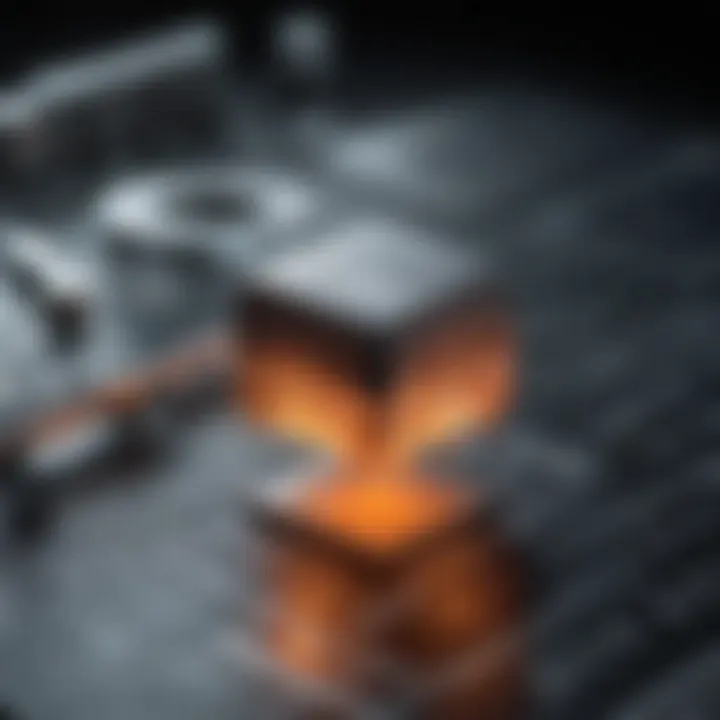
Intro
In today's fast-paced technological landscape, the importance of precise temperature measurement cannot be overstated. Semiconductor temperature sensors, in particular, have emerged as critical components across various industries. These sensors leverage the unique properties of semiconductor materials to provide accurate temperature readings.
Semiconductor temperature sensors are not just another gadget; they are the backbone of numerous applications, ranging from everyday electronics to automotive systems and environmental monitoring. Their ability to function effectively in diverse environments makes them indispensable.
As we embark on a deeper exploration of semiconductor temperature sensors, it’s essential to grasp the fundamental aspects of how they operate, the technology behind them, and what the future might hold for these devices.
Key Findings
Summary of the main results
Through extensive research and analysis of semiconductor temperature sensors, several critical insights have emerged:
- Operational Efficiency: Semiconductor sensors demonstrate high sensitivity and rapid response times, allowing for immediate temperature fluctuations detection.
- Material Innovation: Advancements in materials—such as silicon, germanium, and compound semiconductors—contribute significantly to performance enhancement.
- Energy Efficiency: Many semiconductor sensors are designed to operate with minimal energy consumption, making them suitable for battery-powered applications.
Significance of findings within the scientific community
The importance of these findings extends beyond just academic analysis. In the scientific community, understanding the mechanisms behind semiconductor temperature sensors can lead to advancements in other fields. For instance, their reliability and stability can significantly enhance the development of smart devices, enabling a new level of interconnectedness in the Internet of Things (IoT).
"Incorporating accurate temperature measurements in IoT devices can optimize performance and extend the lifespan of countless applications."
Implications of the Research
Applications of findings in real-world scenarios
The implications of semiconductor temperature sensors’ functionality reach far and wide:
- Electronics: From consumer gadgets to industrial equipment, these sensors ensure temperature-controlled environments to protect sensitive components.
- Automotive Sector: Smart cars rely on these sensors for monitoring engine temperatures to optimize performance and fuel efficiency.
- Environmental Monitoring: Understanding climate changes is easier with precise temperature data, crucial for everything from agriculture to urban planning.
Potential impact on future research directions
The future of semiconductor temperature sensors looks promising. With ongoing research on material science and signal processing technologies, we can expect even higher performance and new applications. More breakthroughs could lead these sensors to play a pivotal role in developing autonomous systems that rely on real-time environmental data.
Prelude to Semiconductor Temperature Sensors
When it comes to devices that ensure stability and efficiency in various applications, semiconductor temperature sensors take center stage. These sensors are not just critical components but the backbone of modern technology, playing roles in everything from home appliances to sophisticated industrial systems. The significance of understanding these sensors lies in their ability to provide precise temperature measurements, which can drastically influence performance and reliability.
Defining Semiconductor Temperature Sensors
Such sensors rely on the proper properties of semiconductors, ensuring they respond accurately to temperature changes. By monitoring these variations, systems can adapt and optimize their functionality, leading to better energy efficiency, safety, and overall effectiveness. The ability to monitor and analyze temperature in real time highlights their importance across numerous fields, particularly when precision is key.
Definition and Overview
A semiconductor temperature sensor is essentially a device that detects temperature by utilizing the characteristics of semiconductor materials. Unlike traditional thermometers that might use mercury or alcohol, these sensors operate based on the principles of electrical resistance. As temperature fluctuates, so does the resistance of the material. This variability can be effectively harnessed into an electrical signal that can be measured and interpreted, which brings forth notable efficiencies in processing temperature data.
The principle revolves around the relationship between voltage, current, and the temperature coefficient of the semiconductor. This dynamic mechanism makes these sensors highly adaptable in various settings, ranging from consumer electronics to precise medical devices.
Historical Context
To fully appreciate the modern semiconductor temperature sensors, it's beneficial to glance back through history. The path leading to the development of these devices runs through the evolution of thermistors in the 1950s. Initially, the reliance was more on thermocouples and resistance temperature detectors. Those devices, while functional, had their own limitations in terms of accuracy and response times.
With growing demand for miniaturization and increased performance, semiconductor technology emerged as a game-changer. This shift became evident during the 1970s when integrated circuits took off. During this time, engineers recognized that semiconductor materials could be optimized to offer precise readings while occupying significantly less space than their predecessors.
Today, semiconductor temperature sensors reflect several decades of innovation, integrating advanced materials such as silicon and gallium arsenide, which not only improved efficiency but also opened up a wide range of applications—not just within industrial devices but also in consumer electronics. Their adaptability over time stands as one of the major milestones in sensor technology.
"The only way to do great work is to love what you do." - Steve Jobs
As we further explore the specific types, operating principles, and challenges faced by semiconductor temperature sensors, it becomes clear that their integration into various fields heralds a bright future for both technology and the optimization of thermal management.
Types of Semiconductor Temperature Sensors
In the realm of temperature sensing technologies, semiconductor sensors represent a crucial segment that merits exploration. These devices, celebrated for their precision and versatility, offer various types, each with unique characteristics and suited to specific applications. Understanding the different types of semiconductor temperature sensors not only enriches one’s knowledge but also supports informed decisions in relevant fields. Their roles in electronics, automotive safety systems, and environmental monitoring underscore their significance in our increasingly automated and data-driven world.
Thermistors: Characteristics and Applications
Thermistors are a type of semiconductor sensor with resistive properties that change significantly with temperature alterations. These sensors are usually made from ceramic materials that undergo a drastic change in resistance at specific temperature thresholds.
Maintaining accuracy in various thermal environments, thermistors can be classified as either negative temperature coefficient (NTC) or positive temperature coefficient (PTC). NTC thermistors decrease in resistance as temperature rises, making them highly favored for precision applications. Conversely, PTC thermistors experience a resistance increase with rising temperatures, adapting well to overcurrent protection scenarios.
Their extensive applications range from everyday household items like thermometers to complex automated systems in industrial settings. For instance, NTC thermistors often find roles in HVAC systems, enabling precise climate control by continually monitoring temperature fluctuations. This makes them vital in optimizing energy efficiency while maintaining comfort within spaces.
Moreover, thermistors are notably cost-effective, allowing manufacturers to implement them without breaking the bank. Their widespread availability in compact sizes enables usage in various devices while keeping the footprint minimal.
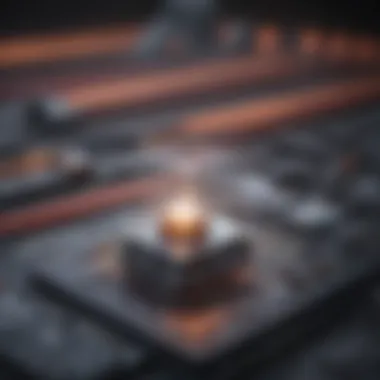
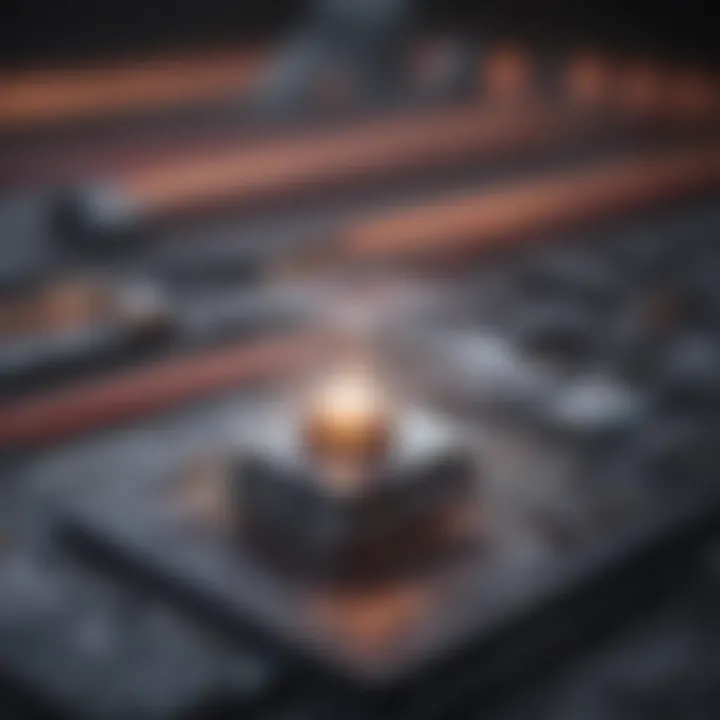
Silicon-Based Sensors
Silicon-based temperature sensors are another fundamental type that relies on the semiconductor properties of silicon. These sensors typically exploit the temperature dependency of silicon’s electrical conductivity.
Silicon sensors can range widely in design, from traditional diodes to advanced integrated circuit (IC) sensors. They often deliver an impressive linear output, making signal interpretation straightforward and reliable. Their widespread integration is evident in devices such as microprocessors, where precision temperature readings ensure optimal performance.
One of the critical advantages of silicon-based sensors is their ability to withstand harsh conditions and operate across a broad temperature range. This robustness makes them suitable for various industries, including aerospace and automotive, where reliability is paramount. Additionally, their compatibility with existing semiconductor fabrication processes speeds up production and integration.
Metal-Oxide Sensors
Metal-oxide sensors represent yet another innovative class of semiconductor temperature sensors. Compared to thermistors and silicon-based devices, metal-oxide sensors employ a different approach by utilizing metal oxides like tin oxide, which have desirable conductive properties. These materials can sense temperature variations through changes in their conductivity.
These sensors are praised for their sensitivity and response speed, often used in environmental applications where quick measurements are crucial. For instance, they are implemented in air quality monitoring devices, detecting temperature changes that may indicate the presence of pollutants. One of the standout features of metal-oxide sensors is their environmental resilience, enabling their use in diverse outside settings, from urban cities to remote monitoring stations.
Fundamental Operating Principles
The functionality of semiconductor temperature sensors hinges largely on their fundamental operating principles. Understanding these principles is crucial as they dictate how these sensors respond to temperature changes and subsequently impact their efficiency and accuracy in real-world applications. The underlying mechanisms involve the interaction of temperature with electrical properties, primarily resistance, voltage, and current. The insights from these principles can not only illuminate how to effectively utilize these sensors but also help in troubleshooting and optimizing their applications.
Temperature Dependence of Resistance
The relationship between temperature and resistance in semiconductor materials is one of the central tenants of these sensors. When temperature increases, the resistance of a semiconductor typically decreases due to the increased number of charge carriers. This characteristic is particularly pronounced in materials like silicon, which are commonly used in various sensors.
Understanding this temperature-resistance correlation can be beneficial in accurately interpreting sensor outputs. For instance, if a sensor shows a decrease in resistance, one can deduce that temperature is likely rising.
Key considerations include:
- Material Properties: Different materials exhibit varying temperature-resistance characteristics. Silicon, germanium, and certain metal oxides have unique curves that must be studied for specific applications.
- Calibration: Sensors need proper calibration to align their resistance changes with actual temperature measurements, ensuring reliable data.
- Linear vs. Non-linear Response: Some materials provide a linear relationship over a specific temperature range, while others do not, complicating the interpretation of results.
Voltage and Current Interpretation
Another significant aspect of semiconductor temperature sensors lies in the interpretation of voltage and current signals. These signals often evolve from the resistance changes as current flows through the sensor. When a voltage is applied, it induces a current which can then be analyzed to gauge the sensor's temperature readings. This process is foundational for various applications ranging from basic thermometry to complex control systems.
There's a few crucial elements to consider:
- Ohm's Law Application: In many cases, Ohm's Law plays a pivotal role in interpreting voltage and current relationship in the sensor. A clear understanding of resistance and how it varies can allow practitioners to predict outputs accurately.
- Signal Conditioning: Sensors must often undergo signal conditioning to convert raw data into usable information. Filtering and amplification techniques may be applied to enhance the sensor's output, granting clearer readings in noisy environments.
- Digital vs Analog Interpretation: Depending on the application, the signal can be processed in analog or digital formats. Digital algorithms, for example, might handle the data more efficiently, enabling higher accuracy in readings and more complex calculations.
"Understanding the fundamental principles of semiconductor temperature sensors not only aids in their application but also enhances performance across interdisciplinary fields, whether that be in electronics, automotive safety, or environmental monitoring."
Through these principles, one can appreciate the delicate interplay of temperature, resistance, current, and voltage in realizing the true potential of semiconductor temperature sensors. Recognizing how each factor contributes to the sensor’s functionality is vital for carrying out successful applications and improvements in technology.
Key Parameters Influencing Performance
The performance of semiconductor temperature sensors hinges on several critical parameters that directly impact their effectiveness and reliability in various applications. Understanding these factors is essential for anyone working with or studying these sensors. Choosing a semiconductor temperature sensor isn’t as simple as picking one off a shelf; it requires an informed decision based on how accuracy, speed, and operational range align with the intended use. In this section, we will delve into three primary parameters: accuracy and precision, response time, and temperature range limitations. Each of these elements plays a pivotal role in determining the overall utility of a sensor in real-world applications.
Accuracy and Precision Measures
Accuracy and precision are cornerstones of effective temperature measurement. While the two terms are often used interchangeably, they encapsulate different aspects of sensor performance. Accuracy is about how close the sensor readings are to the true temperature, while precision refers to the consistency of those readings under the same conditions.
In practical terms, suppose you are monitoring the operating temperature of a critical component in a high-performance electronics setup. If your sensor is accurate, the readings will reflect the actual temperature, ensuring the component operates within safe limits. On the flip side, if the sensor is precise but biased (i.e., consistently off by a few degrees), it might give you consistent data, but that won't help if you're acting on incorrect information.
- Calibration is integral to achieving high accuracy and precision. Regular adjustments based on known standard temperatures are necessary to keep sensors performing optimally.
- The manufacturing process also greatly affects these metrics; higher quality control during production can yield sensors with better accuracies and precisions.
Getting these measures right can make or break applications, especially in critical areas like medical devices, automotive safety systems, and environmental monitoring.
Response Time Considerations
Response time is another crucial parameter that can’t be overlooked. It refers to how quickly a temperature sensor can react to changes in temperature. In fast-paced environments, such as in automotive systems or electronic circuitry, a slow response can lead to ineffective adjustments or even failures.
For instance, consider a semiconductor sensor tracking the temperature of an engine. If the response time is long, the sensor may not detect a spike in temperature until it's too late, potentially causing damage. On the other hand, a sensor with a rapid response time can provide immediate feedback, allowing for timely interventions.
Some key contributors to response time include:
- Sensor mass: Lighter sensors tend to respond faster because they require less energy to heat up or cool down.
- Installation location: Sensors positioned in direct contact with the medium they are measuring may respond more quickly than those placed further away.
Temperature Range Limitations
All semiconductor temperature sensors have a defined operational range, within which they perform accuracy and reliability. This range is determined by both the material properties of the sensor and environmental factors.
For example, a silicon-based sensor might effectively measure from -55°C to +150°C. However, if the temperature exceeds these limits, the accuracy of the readings can drastically reduce, leading to potential hazards or malfunctions.
When selecting a semiconductor temperature sensor for a specific application, consider:
- Maximum and minimum temperatures: Ensure the sensor can operate comfortably within the extremes expected in your environment.
- Long-term stability: Sensors might drift over time when subjected to extreme temperatures, which could necessitate more frequent calibrations or replacements.
"Selecting a temperature sensor without understanding its range limitations is like wearing shoes that are two sizes too small—both impractical and risky for long-term use."
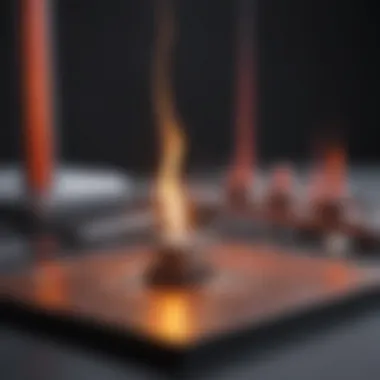
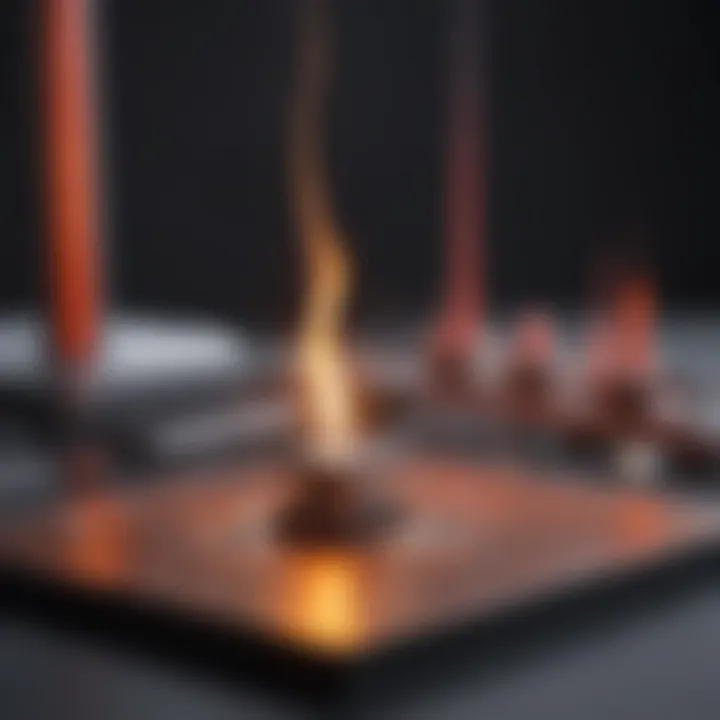
By understanding these key parameters—accuracy and precision, response time, and temperature range limitations—researchers and practitioners can better assess the appropriateness of semiconductor temperature sensors for their specific needs. These factors not only ensure effective temperature monitoring but also enhance the reliability and longevity of the systems in which these sensors are embedded.
Manufacturing Processes and Materials
In the realm of semiconductor temperature sensors, the processes and materials employed during manufacturing play a crucial role in defining sensor performance. Choosing the right materials and refining fabrication techniques can significantly impact reliability, accuracy, and overall utility of these devices. Semiconductor sensors must often perform under varying conditions, so understanding the interplay between materials and manufacturing helps unveil the full potential of these sensors.
Material Selection for Sensors
Material choice remains a cornerstone for developing effective semiconductor temperature sensors. The most common materials include silicon, germanium, and various metal-oxide compounds. Each of these materials brings unique properties that can be tailored to specific applications.
- Silicon: Often favored for its excellent electronic properties, silicon is widely used in the creation of a range of sensors. It offers good thermal conductivity and is relatively easy to integrate into existing electronic systems.
- Germanium: While less common than silicon, germanium is an alternative known for higher sensitivity. It is particularly effective in specific temperature ranges, making it suitable for niche applications.
- Metal-Oxide Compounds: Compounds like tin oxide or zinc oxide are also noteworthy. They exhibit unique sensor capabilities, particularly in environments that involve harsh chemicals or high humidity.
Selecting the right material depends on factors such as temperature range, desired sensitivity, and environmental conditions. Thus, each application brings its own set of requirements, emphasizing the need for thoughtful material selection.
Fabrication Techniques and Innovations
The methods employed in the fabrication of semiconductor temperature sensors are as varied as the materials used. Innovations in these techniques continue to advance the field, allowing for more precise and efficient production.
Recent trends in sensor fabrication often focus on miniaturization and enhanced performance. Techniques such as microelectromechanical systems (MEMS) fabrication—where mechanical and electronic components are integrated into one tiny chip—are noteworthy. This can lead to compact sensors that don’t compromise on performance. The benefits of such techniques include:
- Reduced Size: Smaller sensors can fit into tighter spaces without losing functionality.
- Improved Response Times: The minimized mass allows for quicker thermal response, essential for certain applications.
- Higher Integration: It’s easier to combine sensors with other electronic components, creating multifunctional devices.
Additonally, advances in printing technology are opening doors for innovative approaches to sensor design. Technologies like inkjet or screen printing enable the application of materials directly onto substrates, which can lower production costs and expedite processes.
"The evolution of manufacturing techniques plays a pivotal role in shaping the capabilities of semiconductor temperature sensors, as we forge a path toward smarter, more reliable technologies."
As industries continue to push the boundaries of what’s possible, the emphasis on material selection and innovative fabrication techniques will remain fundamental in driving the future of semiconductor temperature sensing.
Applications Across Various Industries
Understanding the role of semiconductor temperature sensors in various industries is crucial, as it directly influences product design, safety, and efficiency across different applications. By delving into specific applications, this section highlights how these sensors contribute not only to technological advancement but also to consumer safety and environmental sustainability. The benefits of using semiconductor temperature sensors span accuracy, response time, and miniaturization, underscoring their significance in multiple sectors.
Electronics and Computer Technology
In the realm of electronics, semiconductor temperature sensors are indispensable. Every modern electronic device, from smartphones to PCs, generates heat during operation. By employing these sensors, manufacturers can monitor temperature fluctuations, ensuring optimal performance and preventing overheating.
The integration of temperature sensors into circuit boards provides real-time data crucial for managing thermal conditions. For instance, in microprocessors, maintaining a steady temperature can prolong the lifespan of components and enhance reliability. Here’s a closer look at some specific applications:
- Thermal Management: The use of thermistors in laptops aids in cooling system optimization.
- Consumer Devices: Smart thermostats in households leverage these sensors for energy efficiency.
- Wearable Technology: Fitness trackers utilize semiconductor sensors to monitor body temperature, contributing to health metrics.
Automotive Safety Systems
The automotive industry has embraced semiconductor temperature sensors in automotive safety systems with open arms. These sensors are critical for maintaining optimal operating conditions in vehicles, especially in safety mechanisms.
For example, many modern vehicles utilize temperature sensors to monitor the engine's status. When a vehicle overheats, the system can trigger coolant flow or alert the driver, thereby avoiding potential breakdowns or accidents. Additionally, semiconductor sensors are vital in systems like air conditioning, ensuring comfort and efficiency. They play a pivotal role in:
- Temperature Regulation: Monitoring engine temperature to prevent overheating.
- Battery Management: In electric vehicles, sensors help manage battery temperature, which is crucial for performance and longevity.
- Interior Climate Control: Ensuring cabin temperatures are comfortable without wasting energy.
Environmental Monitoring
The environmental applications of semiconductor temperature sensors cannot be overlooked. With global discussions surrounding climate change and energy efficiency, these sensors have become tools for sustainable practices.
From monitoring the temperature of natural bodies of water to tracking weather patterns, semiconductor sensors gather essential data. Their role in:
- Climate Research: Assisting scientists in studying temperature fluctuations over time in different ecosystems.
- Pollution Control: Monitoring industrial emissions helps maintain compliance with environmental regulations.
- Smart Agriculture: Sensors in agricultural settings track soil and micro-climate temperatures, aiding in crop management and yield predictions.
"The accuracy and rapid response of semiconductor temperature sensors are critical for innovation not only in the tech industry but also in our efforts to mitigate environmental challenges."
The depth and versatility of semiconductor temperature sensors across various industries illustrate their necessity in contemporary applications. Their function goes beyond simple temperature measurement; it encompasses providing data that drives innovation and ensures safety, efficiency, and sustainability.
Signal Processing Techniques
Signal processing techniques play a significant role in enhancing the functionality and reliability of semiconductor temperature sensors. These techniques transform the raw data collected by the sensors into meaningful information that can be utilized effectively in various applications. By employing different methods, it is possible to improve the signal quality and accuracy, which is crucial in settings where precise temperature readings are essential.
Analog Signal Conditioning
Analog signal conditioning is a pivotal first step in the processing of temperature sensor signals. It involves preparing an analog signal for further processing or conversion into digital format. This conditioning can include amplification, filtering, and linearization. For example, when dealing with thermistors, their resistance changes with temperature, and this change needs to be converted into a voltage output. Here are some key points regarding analog signal conditioning:
- Amplification: Many sensors generate small output signals. Amplifiers strengthen these signals, enabling better resolution in subsequent measurements.
- Filtering: Noise can significantly affect signal integrity. By using filters, irrelevant signal components can be removed, leading to cleaner data.
- Linearization: The output of certain sensors can be non-linear. Linearization techniques ensure that the output signal relates directly to temperature, making it easier to interpret.
Analog conditioning is not just about making the signal stronger, but also smarter—enhancing clarity and reliability. In essence, it helps build a solid foundation for accurate temperature measurement.
Digital Conversions and Algorithms
Once the analog signal is conditioned, it’s time for digital conversion. Here, the analog signal is translated into a digital format through devices like analog-to-digital converters (ADCs). Digital conversions bring with them various advantages:
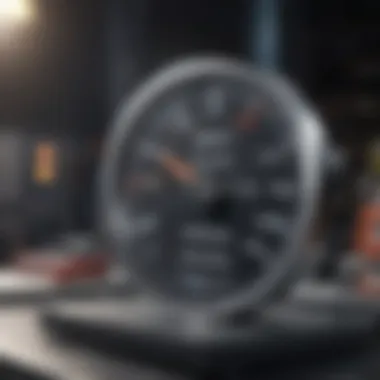
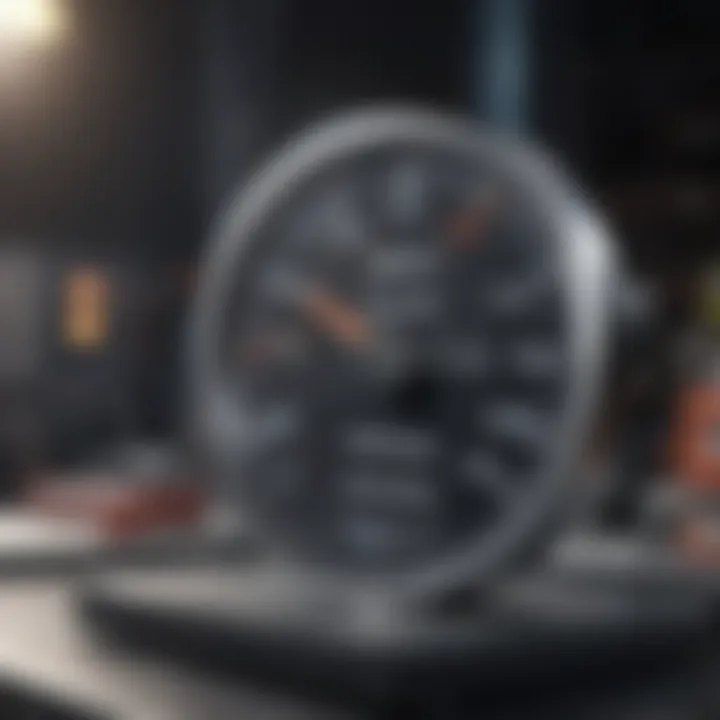
- Precision: Digital signals are less susceptible to noise and interference, which is crucial for accurate readings over time.
- Storage and Transmission: Digital data can be easily stored and transmitted without degradation, providing better long-term reliability.
Following conversion, algorithms come into play to further analyze the temperature data. These algorithms can range from simple averaging methods to complex statistical analyses. Implementing algorithms enables the extraction of meaningful insights from raw data.
As technology continues to improve, the algorithms are becoming more sophisticated, utilizing machine learning techniques to forecast temperature trends and detect anomalous behavior.
In summary, the integration of advanced signal processing techniques, including analog signal conditioning and digital conversions with algorithms, elevates the functionality of semiconductor temperature sensors. This ensures that the readings not only hold accuracy but also offer valuable insights for decision-making across various industries.
Challenges in Semiconductor Temperature Sensing
The quest to perfect semiconductor temperature sensors is not without its hurdles. Understanding these challenges is crucial for engineers, researchers, and anyone involved in sensor technology. Effectively tackling these issues can mean the difference between a reliable sensor and one that wavers under pressure—literally and figuratively. Factors like environmental influences and the incessant need for calibration play significant roles in determining how well these sensors perform.
Environmental Influences on Measurements
Semiconductor temperature sensors are, by nature, sensitive devices. They don't just measure temperature; they’re reactive to their surroundings. Ambient conditions such as humidity, electromagnetic interference, and physical vibrations can throw off the sensor's readings. For instance, a sensor placed in an industrial setting with heavy machinery may experience deviations in temperature readings due to the fluctuating electromagnetic fields emitted by the machines.
- Humidity: High moisture levels can disrupt the semiconductor materials, leading to altered performance.
- Temperature Gradients: If a sensor measures temperature in an area where there are heat sources or cold drafts, it can yield misleading results.
- Electromagnetic Interference: Devices that generate electric fields can affect the accuracy of readings by inducing noise in the sensor’s output.
Making reliable measurements often involves shielding the sensor or designing specialized enclosures to counter these environmental disturbances. Understanding how these external factors interplay with the internal workings of the sensors is an essential area of research.
Calibration and Maintenance Needs
Calibration is the cornerstone of ensuring accuracy in semiconductor sensors. An uncalibrated sensor can lead to erroneous readings, which can have dangerous implications in critical applications like automotive safety or medical devices. Regular calibration against known standards is necessary to maintain the integrity of measurements. Moreover, maintenance demands careful attention as dirt, debris, or even age can render a sensor ineffective.
- Frequency of Calibration: Depending on the application, some sensors require daily, weekly, or monthly calibration. For example, in medical instruments like thermometers, accuracy is paramount for patient safety.
- User Training: Users must be trained not only on how to use the sensors but on the importance of calibration routines and how to execute them effectively.
- Sustainability: The materials used in sensors can degrade over time, which necessitates regular checks and sometimes replacement.
"A calibrated sensor adds value to a system; an uncalibrated one can jeopardize its safety and functionality."
Reliability in semiconductor temperature sensing isn't solely about the materials used or the design itself; it encompasses continuous attention to these challenges. Facing them head-on is the only way the technology can evolve and meet the demands across various industries.
Future Trends and Developments
The landscape of semiconductor temperature sensors is evolving at a rapid pace, mirroring technological advancements in various sectors. Understanding these future trends not only highlights the progression of sensor technologies but also underlines the significance of these sensors in the context of modern applications.
As industries increasingly adopt automation and data analytics, the integration of IoT technologies into semiconductor temperature sensors plays a pivotal role. This integration enables devices to communicate data in real-time, which allows for more precise monitoring and control processes in various systems. For instance, consider the role of temperature sensors in smart homes; when coupled with IoT capabilities, these sensors not only react to temperature changes but also relay information about energy consumption, ultimately helping homeowners optimize their heating and cooling systems.
Additionally, the incorporation of machine learning algorithms into temperature sensor systems enhances their reliability and predictive capabilities. These smart sensors can learn from historical data and make informed decisions, which is invaluable in industrial settings where equipment failure can lead to significant downtime and financial loss.
"The rise of smart sensors is not just about innovation; it's about creating systems that can think independently and make proactive adjustments to maximize efficiency."
Integration with IoT Technologies
The melding of semiconductor temperature sensors with IoT technologies represents a significant stride toward smarter operations. Sensors embedded in devices like HVAC systems, industrial machinery, and consumer electronics can now not only collect data but also send this information over the internet for analysis and reporting. This process fundamentally alters how organizations approach maintenance and operational efficiency.
Some key benefits of integration include:
- Real-Time Monitoring: Immediate feedback on temperature variations allows for swift actions to be taken, preventing potential hazards or operational inefficiencies.
- Remote Access: Users and operators can monitor systems from afar, providing flexibility and responsiveness to issues that may arise.
- Data Accumulation for Analytics: Continuous data flow feeds into analytical tools, enabling trend analysis that can predict issues before they arise.
Considerations for implementing IoT technologies with semiconductor sensors also need to be addressed:
- Data Security: The increase in connectivity can expose systems to vulnerabilities; measures must be taken to safeguard against breaches.
- Compatibility: Ensuring sensors can function seamlessly with various IoT platforms is crucial for effective deployment.
Smart Sensors and AI Integration
The field of semiconductor temperature sensors is witnessing a transformative shift through the infusion of artificial intelligence. Smart sensors equipped with AI capabilities can process vast amounts of data and extract meaningful insights to operate more efficiently than ever before.
For example, in healthcare settings, smart temperature sensors can continuously monitor patient vitals. By leveraging AI, these sensors can identify abnormal readings and alert healthcare providers instantaneously, which can be critical for patient safety.
Important elements of smart sensors and AI integration include:
- Self-Calibration: AI can enable sensors to self-adjust their calibration based on environmental conditions and operational history, reducing the need for manual recalibrations and improving accuracy.
- Predictive Maintenance: By analyzing data trends, AI can predict when a sensor or system might fail, allowing for timely maintenance before issues disrupt operations.
- Optimized Energy Usage: AI algorithms in smart sensors can analyze usage patterns and adjust settings accordingly, contributing to significant energy savings.
Overall, the intersection of semiconductor temperature sensors, IoT technologies, and AI represents a frontier with immense potential. As these technologies continue to advance, they not only enhance the functionality of temperature sensors but also redefine how industries monitor and react to temperature variations, leading to more effective and efficient operations across the board.
Epilogue
The final thoughts on semiconductor temperature sensors are crucial for understanding their widespread relevance in today's technological landscape. Throughout this article, we've explored their functions, applications, and the evolving technologies that drive their effectiveness. These sensors are not just small components; they play a big role in the performance and reliability of countless systems, from consumer electronics to automotive safety features.
Summary of Key Insights
- Diverse Applications: Semiconductor temperature sensors are integral to various sectors, including electronics, automotive, and environmental monitoring. Understanding these applications helps us appreciate their versatility and significance in modern engineering.
- Technical Advancements: Innovations in materials and signal processing techniques continue to enhance the accuracy and response times of these sensors. Keeping abreast of these developments ensures that researchers and practitioners can implement the best solutions for their needs.
- Performance Influencers: Several parameters, such as accuracy, precision, and response time, significantly influence the operational reliability of these sensors. Being aware of these details allows for better design choices in projects involving temperature measurement.
- Challenges and Solutions: Environmental factors, calibration issues, and maintenance requirements pose challenges for semiconductor temperature sensors. However, ongoing research aims to address these obstacles effectively, ensuring long-term viability in various applications.
"The excellence of semiconductor temperature sensors lies not just in their accuracy but also in their adaptability to a rapidly changing technological environment."
Importance of Continued Research
The study of semiconductor temperature sensors is far from complete. Continued research is vital for several reasons:
- Evolution of Technology: As technology advances, so does the demand for more precise and efficient temperature sensors. Research helps to identify new materials and designs that can deliver improved performance.
- Integration with Emerging Technologies: The growing intersection of semiconductor sensors with IoT and AI technologies opens new avenues for innovation that researchers must explore to unlock the full potential of these sensors.
- Addressing Real-World Needs: Real-world applications bring unique challenges that need ongoing research to solve. With industries evolving, understanding these dynamics ensures that semiconductor sensors remain relevant and effective.
- Sustainability Considerations: As global focus shifts toward sustainability, research into eco-friendly materials and energy-efficient designs for sensors is essential.
In essence, the future of semiconductor temperature sensors is bright and interconnected with the continuing growth of technology. Fostering research and development in this field is imperative not just for technical progress but also for addressing societal needs related to safety, efficiency, and environmental impact.