Understanding p53 Molecular Weight: Key Insights and Implications
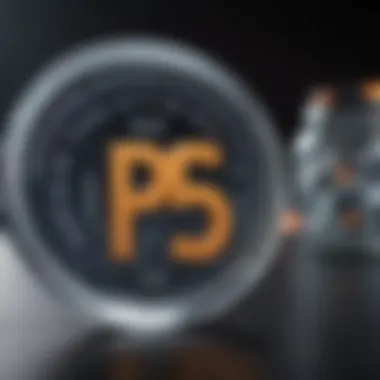
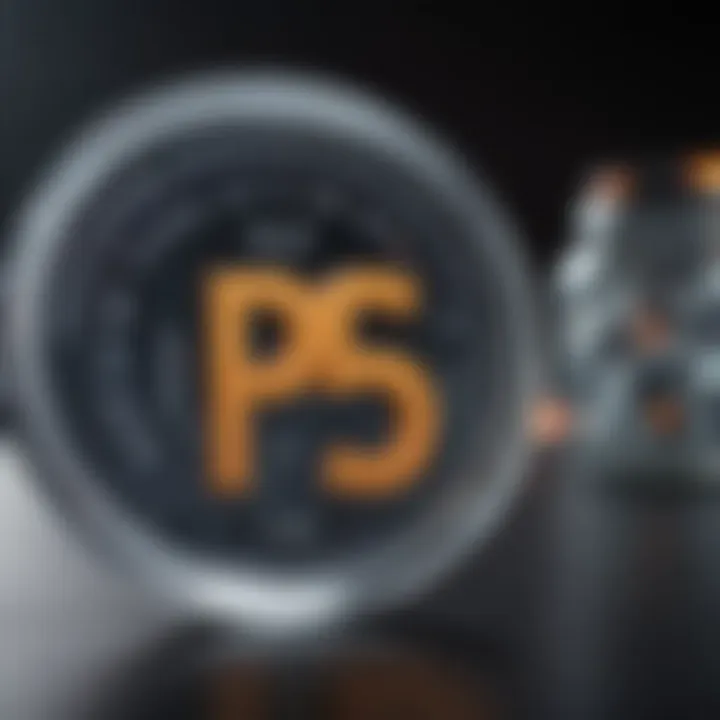
Intro
The p53 protein is often referred to as the "guardian of the genome" due to its pivotal role in maintaining genomic stability. Understanding the molecular weight of p53 provides crucial insights into its function and interactions within the cell. This article delves into the characteristics of p53, focusing on its molecular weight and the implications of this attribute in cancer biology.
Research surrounding p53 has expanded significantly in recent years, shedding light on its fundamental influence in cellular processes like apoptosis, cell cycle regulation, and DNA repair. As we delve into the subject, we aim to provide a comprehensive exploration of why understanding the molecular weight of p53 is essential for researchers and practitioners in the biomedical field.
Key Findings
Summary of the Main Results
The examination of p53's molecular weight reveals that it typically ranges between 53 and 60 kDa. This variability often arises from alternative splicing and post-translational modifications, affecting its functionality and molecular interactions.
"The p53 protein's role is not only essential in tumor suppression, but also in many cellular homeostatic functions."
Investigations show that variations in p53’s molecular weight can influence its ability to bind to DNA, interact with other proteins, and execute its role in cell cycle regulation. The presence of specific isoforms has been noted to correlate with different biochemical pathways within the cell, highlighting the complexity of its regulatory capabilities.
Significance of Findings Within the Scientific Community
The discoveries surrounding p53's molecular weight have generated significant interest in the scientific community. These findings underscore potential mechanisms by which mutations can lead to cancer progression. Understanding these mechanisms is imperative for the development of targeted therapies. Adjustments to p53’s molecular weight might reveal novel strategies for cancer treatment and early detection methods, further improving cancer management.
Implications of the Research
Applications of Findings in Real-World Scenarios
The insights gained from studying p53's molecular weight can be applied to various aspects of cancer research and treatment. Clinicians can potentially use specific markers associated with variations in p53 to assess tumor behavior, predict patient outcomes, or tailor individualized therapies.
Moreover, understanding how different p53 isoforms participate in tumor suppression could lead to new preventive strategies against cancer. The implications reach beyond just treatment; they could also enhance early diagnostic techniques, providing a method to identify cancer at a more treatable stage.
Potential Impact on Future Research Directions
The ongoing research concerning p53 highlights the possibility of new frontiers in cancer therapy and genomics. Future studies could focus on elucidating the mechanisms by which specific mutations alter the molecular weight of p53, leading to detrimental cellular changes. Furthermore, research could explore the tangible effects of manipulating p53’s molecular weight in therapeutic contexts or investigate its interactions with newly discovered partner proteins.
An increased understanding of these dynamics may pave the way for novel treatment modalities, contributing to a more profound grasp of not only p53 but also the broader spectrum of molecular behavior in oncology. Overall, continued exploration into p53 will be vital for enhancing both our scientific knowledge and clinical approaches to cancer.
Preface to p53
In the field of molecular biology, p53 stands as a formidable guardian of the genome. Its significance cannot be overstated. p53 plays a crucial role in regulating the cell cycle, serving as a tumor suppressor, and its stabilization is pivotal in preventing cellular mutations. Understanding the molecular weight of p53 is essential, as this characteristic influences its functionality and interactions within cellular environments. This article aims to elucidate these aspects and the wider implications for health and disease.
Historical Context
The story of p53 began in the early 1970s when it was first identified in tumor cells. Researchers noticed that this protein was consistently associated with various malignancies. Initial understanding of p53 was somewhat limited, and it wasn’t until the late 1980s that its role as a tumor suppressor was recognized. The discovery of the gene encoding p53, TP53, marked a significant milestone. Over the decades, advances in molecular biology techniques have allowed for an in-depth exploration of p53’s structure and function. The historical backdrop sets the stage for understanding current research and its implications in cancer biology.
p53 Functionality and Importance
p53 performs several indispensable roles in cellular processes. Primarily, it regulates the cell cycle by pausing division in the presence of DNA damage, thereby allowing for repair mechanisms to take precedence. If the damage is irreparable, p53 can initiate programmed cell death—apoptosis—thus preventing the proliferation of potentially cancerous cells.
The protein's importance can be summarized as follows:
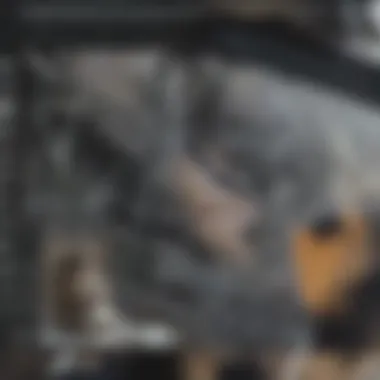
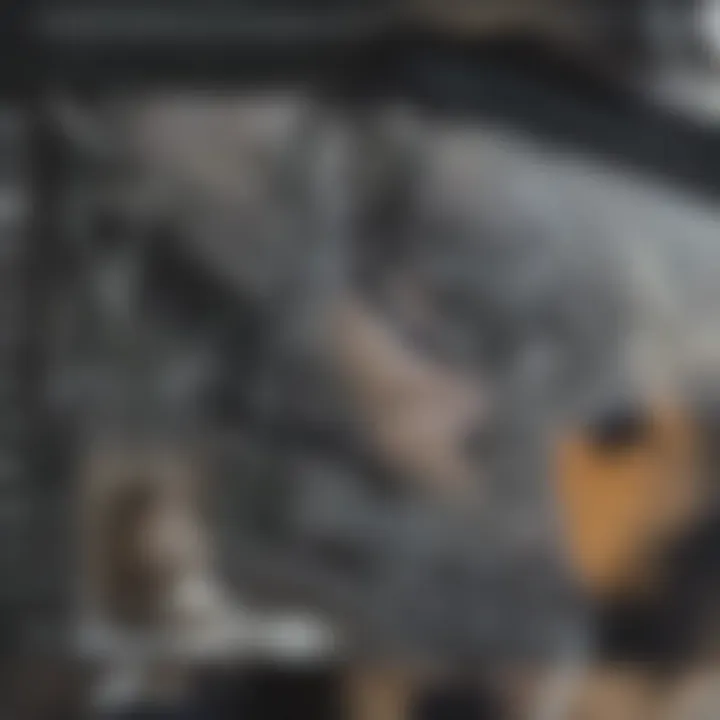
- Cell Cycle Regulation: p53 ensures that cells do not proceed to division if DNA is damaged.
- Tumor Suppression: By promoting apoptosis, it acts as a barrier against tumor formation.
- Genetic Stability: p53 repairs damaged DNA or eliminates compromised cells, safeguarding genetic integrity.
Given these functions, the molecular weight of p53 is more than a numerical value. It correlates with the protein's ability to engage in interactions with other molecules, influencing its efficacy in maintaining cellular homeostasis. The precise understanding of p53's molecular weight, therefore, carries substantial importance in the study of cancer and therapeutic strategies.
Biochemical Properties of p53
The biochemical properties of p53 are fundamental to its function in cellular processes. Understanding these properties is crucial for comprehending how p53 operates within the cell and its implications in cancer biology. This section aims to elucidate the intricacies surrounding the p53 protein's structure and molecular weight, which directly influence its role in tumor suppression and the regulation of the cell cycle.
Structure of p53
The p53 protein is constituted primarily of a central DNA-binding domain, which plays a pivotal role in its activity as a transcription factor. The structure is composed of several key regions:
- Transactivation Domain: This region is essential for initiating transcription of target genes. It contains various regulatory sites that influence the activity of the protein.
- DNA Binding Domain: The core of p53's functionality lies within this domain. It interacts directly with specific DNA sequences, allowing it to activate or repress genes involved in cell cycle regulation and apoptosis.
- Oligomerization Domain: p53 typically functions as a tetramer, which is crucial for its stability and activity. This multimeric state enhances its ability to form a stable complex with DNA.
The three-dimensional configuration of p53 is critical. Any alterations in its structure can lead to functional changes, which is often seen in cancerous cells. Mutations can disrupt its ability to bind DNA, diminishing its tumor-suppressive capabilities. Indeed, over half of human cancers harbor mutations in the p53 gene, underscoring its importance in maintaining genomic integrity.
Molecular Weight Overview
The molecular weight of p53 is approximately 53 kDa. This specific weight is a result of the protein's amino acid composition and sequence. The molecular weight plays multiple roles, particularly in its interactions with other molecules in the cellular environment:
- Impact on Protein-Protein Interactions: The size of p53 can affect its binding affinity with other proteins. In multiprotein complexes, a heavier molecular weight may influence how p53 interacts with co-factors and suppressor proteins.
- Stability and Half-life: Molecular weight can also relate to the protein's stability. Larger proteins may have a shorter half-life, making them more susceptible to degradation pathways. This degradation is vital in regulating p53's activity within the cell.
- Cellular Localization: The weight potentially impacts how the protein is transported within the cell. It can affect diffusion rates and the protein's ability to reach different cellular compartments efficiently.
Understanding the molecular weight of p53, along with its specific structural properties, is essential to grasp how it functions within cells and contributes to the regulation of critical cellular processes. These insights not only provide clarity on p53's role in cancer biology but also pave the way for potential therapeutic strategies targeting its pathways.
Determining Molecular Weight
Understanding the molecular weight of p53 is crucial. It directly influences how this protein behaves within the cell. An accurate measure of molecular weight can reveal information about the protein's folding, interactions, and overall stability. These factors are particularly important in a biological context because they impact p53’s role in cellular processes such as tumor suppression and DNA repair.
Determining the molecular weight assists researchers in understanding variations in p53 function across different conditions. Moreover, it can help in identifying potential biomarkers for diseases where p53 plays a significant role, such as various cancers. The techniques used to measure molecular weight may vary based on the experimental design; therefore, knowledge of these techniques is essential for correct interpretation of results.
Techniques for Measurement
Several techniques exist for measuring molecular weight, each with distinct advantages and limitations. One commonly used method is Mass Spectrometry. This technique allows researchers to analyze the p53 protein and obtain precise molecular weights with high sensitivity. Another widely utilized method is SDS-PAGE (Sodium Dodecyl Sulfate Polyacrylamide Gel Electrophoresis). This technique separates proteins based on their molecular weight and provides a visual representation of the size of p53 in samples.
A third method includes Size Exclusion Chromatography. This approach separates proteins based on their size by passing them through a porous gel. These techniques together provide comprehensive data on p53’s molecular weight, essential for studies that explore its functionality in cellular processes.
Factors Influencing Molecular Weight
Several factors can influence the molecular weight of p53. Mutations in the p53 gene can lead to changes in the amino acid sequence, which in turn may affect the protein's mass. Different mutations can either increase or decrease the molecular weight, depending on the nature of the modification.
Post-translational modifications are another critical factor. The p53 protein can undergo various modifications after its initial translation. Phosphorylation, acetylation, and ubiquitination can add additional molecular weight, affecting p53’s functionality and stability.
Additionally, factors such as environmental conditions, including pH and ionic strength, can also impact protein conformation and, consequently, its measured molecular weight. Understanding these influences helps researchers comprehend the complex nature of p53 and its behavior in different biological contexts.
The precise determination of molecular weight is essential in the study of p53, guiding researchers in understanding its functions and implications in disease.
Functional Implications of p53 Molecular Weight
Understanding the functional implications of p53 molecular weight is essential in appreciating its role in cellular regulation and tumor suppression. The molecular weight of p53 is not merely a characteristic; it is intricately linked to how the protein operates within the biological framework. This section dissects the ways in which molecular weight influences p53's tumor-suppressive capabilities and its interactions with other proteins.
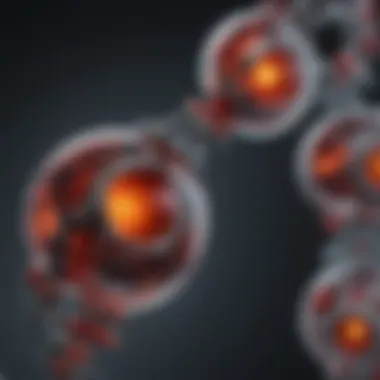
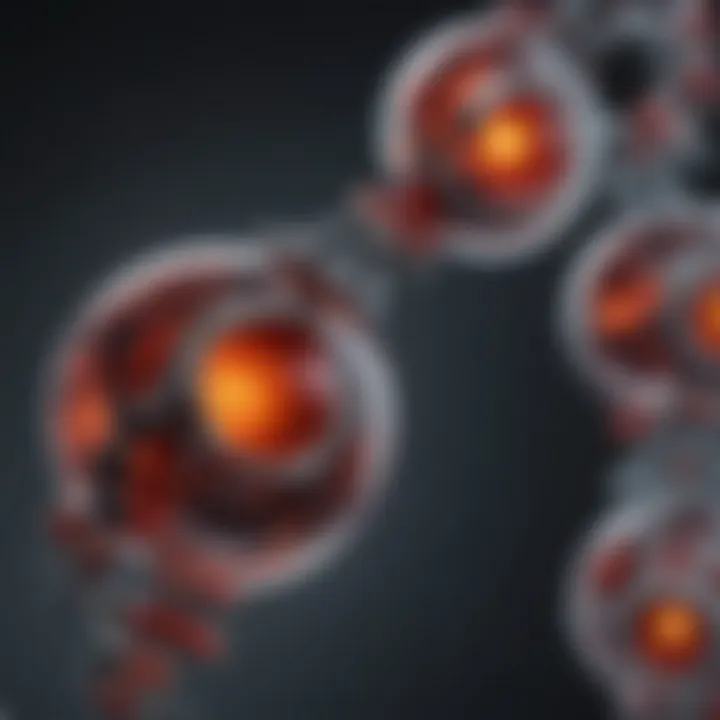
Molecular Weight and Tumor Suppression
The p53 protein plays a pivotal role in preventing cancer development, primarily through its tumor-suppressive functions. The molecular weight of p53 is approximately 53 kDa and is significant for a few reasons:
- Stability: The size and structure of p53 allow it to maintain functionality under various cellular conditions. Its molecular weight ensures that p53 can engage with DNA and other proteins effectively.
- Binding Affinity: Alterations in p53's molecular weight might impact its affinity for binding with target sequences in DNA. The binding is crucial for initiating cell cycle arrest and apoptosis in response to DNA damage.
- Regulatory Feedback: Tumor suppressor activity is closely tied to negative feedback mechanisms. Variations in p53’s molecular weight can affect its regulatory interactions, thus impacting its ability to control cellular responses to stress and DNA damage.
As a result, any mutations or modifications that cause shifts in p53’s molecular weight can lead to reduced tumor-suppressive activity, potentially contributing to cancer progression.
"The molecular weight of p53 must be maintained for its role as a guardian of the genome to function effectively."
Impact on Protein Interactions
The interactions that p53 has with other proteins are vital in its function as a tumor suppressor. The molecular weight of p53 influences numerous aspects of these interactions:
- Binding Partners: p53 interacts with various proteins, including MDM2, which regulates its stability and degradation. Changes in molecular weight can disrupt these interactions, leading to either overexpression or loss of activity.
- Complex Formation: The formation of multi-protein complexes is essential for p53's function. The molecular weight helps in determining how p53 fits within these complexes. A change in size may affect the assembly and function of these critical structures.
- Signal Transduction: p53’s interactions in signal transduction pathways, especially those related to stress response, are inherently linked to its molecular weight. Affects on p53's functionality can cascade through these pathways influencing cellular outcomes.
In summary, the molecular weight of p53 is not only a static measurement but a fundamental aspect that dictates its functionality in tumor suppression and protein interactions. Understanding these implications aids researchers and practitioners in evaluating potential therapeutic strategies aimed at restoring or enhancing p53 function in cancerous conditions.
Variability in Molecular Weight
The molecular weight of p53 is not a static measure; rather, it exhibits variability which is essential to understanding its roles within cellular mechanisms. The relevance of this variablity spans from the basic science of protein functionality to its implications in disease states. Understanding how p53's molecular weight can fluctuate helps to elucidate its multifaceted nature and importance in tumor suppression, cellular regulation, and interaction with other proteins.
This variability can arise from multiple factors, including genetic mutations and post-translational modifications. Each of these elements can alter p53’s properties, influencing how the protein engages in regulating the cell cycle and maintaining genomic integrity. Thus, exploring this variability is crucial for deciphering p53's contribution to both normal cellular functions and pathological conditions.
Mutations and Their Effects
Mutations in the p53 gene are one of the significant contributors to changes in molecular weight. These mutations are often found in several types of cancers. They can lead to the production of p53 variants with altered stability, functionality, and ultimately, a different molecular weight.
Depending on the type of mutation, several outcomes are observed:
- Loss of Function Mutations: These lead to truncated p53 proteins, which typically weigh less than the wild-type version.
- Gain of Function Mutations: Some mutations can cause the protein to acquire new properties, which may also influence its molecular weight.
Additionally, the accumulation of these mutations over time can result in substantial variability in the p53 populations observed in tumors. Research indicates that specific mutations correlate with higher incidence rates of particular cancers, demonstrating the biological importance of understanding p53’s mutation-driven variability in clinical settings.
Post-translational Modifications
Post-translational modifications (PTMs) significantly contribute to the variability in p53’s molecular weight. After translation, p53 undergoes several modifications, such as phosphorylation, acetylation, ubiquitination, and methylation. These changes can alter the protein's mass, interaction dynamics, and functional capacity in various pathways.
Some notable effects of PTMs on p53 include:
- Phosphorylation: Often occurs in response to DNA damage, impacting p53 stability and activity.
- Acetylation: Enhances p53’s ability to regulate transcription of target genes involved in cell cycle arrest and apoptosis.
- Ubiquitination: Generally marks p53 for degradation, potentially reducing its effective concentration within the cell.
Through these modifications, p53 can dynamically adjust its functions in various biological contexts. This adaptability is vital for p53's role in cancer biology, where it faces constant threats from cellular stressors.
In summary, the factors driving the variability in p53's molecular weight, such as mutations and PTMs, are crucial for understanding its biochemical role in health and disease. Knowledge of these nuances not only helps in basic research but also aids in developing targeted therapies aimed at manipulating p53's functions to combat cancer and other diseases.
p53 and Disease Associations
The association of p53 with various diseases extends beyond its well-known role in cancer. Understanding these relationships is crucial as they reveal how dysfunction in p53 contributes to disease pathology and influences therapeutic approaches. This section delves into two primary areas where p53 plays a pivotal role: cancer and genetic disorders. Unraveling these associations not only enhances our comprehension of p53's function but also sheds light on possible intervention strategies in clinical settings.
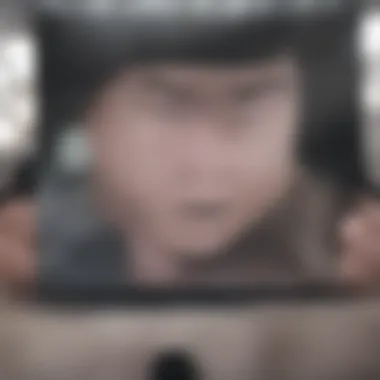
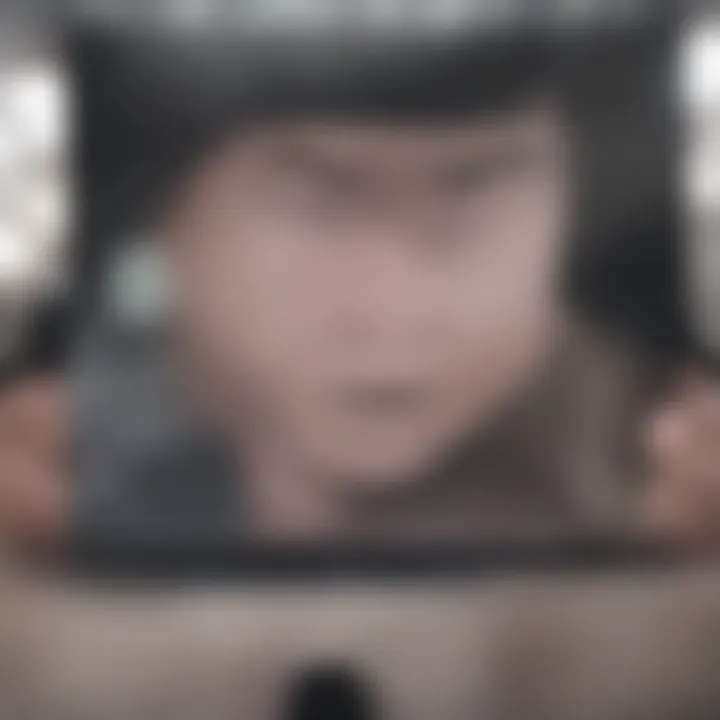
p53 in Cancer
The p53 protein is often described as the "guardian of the genome." Its primary responsibility is to maintain genomic stability, predominantly through regulating the cell cycle. When DNA damage occurs, p53 is activated, leading to either cell cycle arrest, DNA repair, or apoptosis if the damage is irreparable. Mutations in the TP53 gene, which encodes the p53 protein, disrupt this process and can lead to uncontrolled cell proliferation.
Research has shown that approximately 50% of all human cancers harbor mutations in the TP53 gene. This high prevalence underscores its significance in oncogenesis. These mutations can result in the production of a dysfunctional p53 protein that loses its tumor-suppressive capabilities.
Key points regarding p53's role in cancer include:
- Tumor Progression: Mutant p53 proteins can enhance tumor cell survival, proliferation, and metastasis.
- Therapeutic Resistance: Many cancers with p53 mutations exhibit resistance to conventional chemotherapy and radiation treatments.
- Target for Therapy: Restoring the function of mutant p53 or targeting its pathways is a promising area of research in cancer treatment. Studies are evaluating small molecules and gene therapy approaches to revive the activity of p53 in tumors.
"The presence of p53 mutations in tumor samples can serve as a biomarker for prognosis and treatment response."
p53 in Genetic Disorders
Beyond cancer, p53 is implicated in various genetic disorders, which are often characterized by defects in cell cycle regulation and apoptosis. Some of the notable conditions associated with p53 dysfunction include:
- Li-Fraumeni Syndrome: This hereditary disorder is marked by the early onset of multiple cancers due to TP53 mutations. Individuals with this syndrome have a significantly higher risk of developing soft tissue sarcomas, breast cancer, and brain tumors.
- Ataxia-Telangiectasia: In this disorder, frequent DNA damage occurs due to a defective ATM protein, leading to increased p53 activation. While this is a protective response, it also contributes to the neurological decline observed in these patients.
- Neurofibromatosis Type 1: Research suggests that p53 plays a role in the tumorigenesis observed in this condition. Increased p53 activity in cells with NF1 mutations contributes to the development of benign and malignant tumors.
In summary, the association of p53 with cancer and genetic disorders illustrates its critical role in maintaining cellular integrity and highlights the potential for innovative therapeutic interventions aimed at modulating its activity. Understanding these disease associations is vital for developing new treatment modalities.
Research Advancements and Future Directions
The research surrounding p53 has evolved significantly. Understanding the molecular weight of this protein is vital for comprehending its roles and implications in various biological processes, particularly in cancer. With advancements in technology and methods, researchers are beginning to unearth insights that were previously unattainable. This forward momentum not only enhances our grasp of p53's functionality but also its relevance in diverse contexts, including disease prevention and treatment approaches.
Current Studies on p53
Ongoing research efforts are uncovering the intricate details of p53's behavior and its associated pathways. Studies focus on the following key areas:
- Molecular Characterization: Investigations into p53 isoforms and their molecular weights help clarify the protein's diverse functions. This knowledge contributes directly to understanding its tumor suppressor activities.
- Cancer Pathophysiology: Many researchers are examining how variations in p53 affect cancer progression. Findings indicate that mutations or alterations in molecular weight may result in a loss of function, greatly influencing tumor development
- Cellular Response Mechanisms: Recent studies aim to elucidate how changes in the molecular weight alter p53 interactions with other proteins involved in cell cycle control. This can lead to detailed insights into cellular responses to DNA damage and stress.
- Benchmarking Techniques: New methodologies for measuring p53's molecular weight have been developed. These methods enhance the accuracy of research findings and improve the potential for reproducibility.
Research continues to yield promising results. The understanding of p53 has become more nuanced, providing a systematic approach to studying its various roles in health and disease.
Potential Therapeutic Applications
As research unveils the complexities of p53, the potential for therapeutic applications becomes apparent. These applications focus on leveraging p53’s functions for improved cancer treatment and prevention strategies. Some potential avenues include:
- Drug Development: There is growing interest in creating agents that can reactivate mutant p53. These drugs aim to restore the tumor-suppressing capabilities of the protein, offering a new treatment pathway for cancer patients.
- Gene Therapy: Researchers are exploring gene therapy techniques to deliver functional p53 genes to tumor cells. This could help manage or eliminate cancer more effectively.
- Biomarker Utilization: The molecular weight variations of p53 may serve as biomarkers for diagnosing certain cancers or predicting patient outcomes. Tracking these changes can provide critical information for personalized treatment plans.
- Other Disease Implications: Beyond cancer, emerging research suggests that p53 might play roles in other diseases, such as neurodegenerative disorders. Targeting its pathways could reveal new understanding and approaches for these conditions.
In summary, the advancement of research related to p53 and its molecular weight indicates a promising future in both understanding and treating cancer and possibly other disorders. Keeping abreast of these developments is crucial for anyone involved in biomedical research and therapeutic innovation.
"The ability to manipulate p53 and its pathways may redefine cancer therapies in our future research endeavors."
Closure
The conclusion serves as the final synthesis of the insights gained throughout the article, emphasizing the multifaceted role of p53 in cancer biology. It is essential to recognize that molecular weight is more than just a numeric value; it is indicative of p53's functional capacity and interaction with various cellular components like DNA and other proteins. Understanding these nuances allows researchers to grasp how alterations in p53, whether through mutations or post-translational modifications, can disrupt its tumor-suppressive functions, potentially leading to oncogenesis.
Summary of Key Points
- p53's Molecular Weight: The molecular weight of the p53 protein is a critical aspect that influences its behavior in cells.
- Functional Implications: Variations in molecular weight affect p53's ability to bind to DNA and interact with other proteins, impacting its role in the cell cycle and tumor suppression.
- Disease Associations: Alterations in molecular weight can correlate with various diseases, particularly different types of cancer and genetic disorders.
- Research Directions: Future studies may explore therapeutic measures targeting p53’s molecular characteristics to enhance its functions in oncological treatments.
Final Thoughts on p53 Research
Research on p53 continues to evolve, presenting opportunities for better understanding and therapeutic advances. Its pivotal role in cell regulation underscores its potential as a target in cancer therapies. As we move forward, the integration of technological advancements in proteomics and genomics could provide deeper insights into the protein’s molecular weight and functionality. This understanding not only enriches cancer biology but also opens doors to innovative treatments that could transform patient outcomes.
In a world where cancer prevalence continues to rise, elucidating the complex dynamics of p53 becomes increasingly vital. Engaging in multifarious research pathways will likely unearth previously overlooked connections, ultimately enhancing our ability to combat malignancies effectively.