Understanding Oxidative Stress Assays in Research
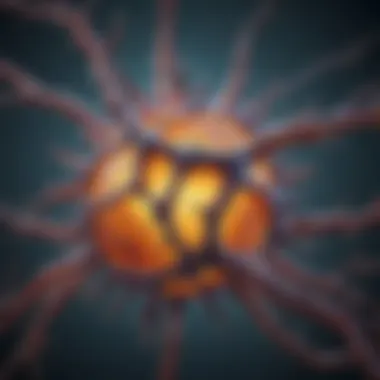
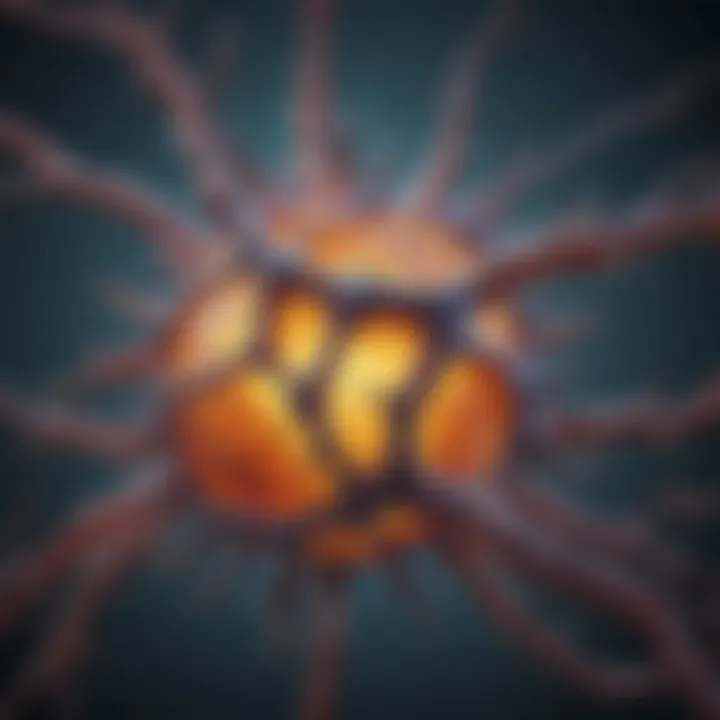
Intro
Oxidative stress refers to an imbalance between reactive oxygen species (ROS) and antioxidants in the body. This condition can lead to cellular damage and is linked to various health issues. To understand these processes, researchers use oxidative stress assays. These assays measure the levels of oxidative stress in biological samples, providing insight into cellular reactions. The significance of these assays goes beyond basic science; they have applications in clinical research, pharmacology, and environmental studies. The advance in methodologies and understanding of oxidative stress has yielded vast potential for improving health outcomes and disease management.
Key Findings
Summary of the Main Results
Oxidative stress assays reveal critical insights into cellular response mechanisms. Studies have shown that increased oxidative stress correlates with the progression of diseases such as cancer, diabetes, and neurodegenerative disorders. Recent findings highlight:
- Elevated levels of malondialdehyde (MDA) as a marker for lipid peroxidation, indicating cell membrane damage.
- The role of glutathione as a crucial antioxidant, showing deficiency in chronic diseases.
- Alterations in mitochondrial function linked to increased oxidative damage in cells.
These results reinforce the need for understanding oxidative stress in biomedical research and clinical settings.
Significance of Findings Within the Scientific Community
The findings underscore the relevance of oxidative stress in health and disease. They encourage a more integrated approach in experimental and clinical research. With robust assay methods, scientists can:
- Develop targeted therapies that modulate oxidative stress levels.
- Design preventive strategies focusing on antioxidant intake through diet or supplements.
- Dive deeper into the molecular pathways affected by oxidative stress.
Researchers are increasingly acknowledging the necessity of oxidative stress assessments as part of comprehensive evaluations in clinical trials.
Implications of the Research
Applications of Findings in Real-World Scenarios
In practice, the data derived from oxidative stress assays inform patient care and preventive strategies. For example:
- In oncology, measuring oxidative stress helps in assessing tumor behavior and therapy responses.
- Clinical nutrition focuses on antioxidants to mitigate the effects of oxidative stress on health.
- Environmental studies utilize these assays to evaluate the impact of pollutants on biological systems.
Today, many pharmaceutical companies are also interested in these assays to explore drug efficacy and safety profiles.
Potential Impact on Future Research Directions
The ongoing exploration of oxidative stress will likely lead to groundbreaking advancements. Anticipated developments include:
- Novel therapeutic interventions targeting oxidative damage.
- Enhanced biomarkers for early detection of diseases related to oxidative stress.
- Integration of oxidative stress findings into personalized medicine.
As technology progresses, we expect the methods for conducting oxidative stress assays to become more sophisticated, providing deeper insights into cellular health.
Intro to Oxidative Stress
Oxidative stress is a fundamental concept in numerous disciplines within scientific research. Understanding oxidative stress is crucial to grasp the relationships between environmental factors, cellular processes, and various diseases. This introduction outlines the importance of oxidative stress, setting the stage for a deeper exploration into its mechanisms and implications throughout the article.
Significance of Oxidative Stress
Oxidative stress occurs when there is an imbalance between reactive oxygen species (ROS) production and the body’s ability to detoxify these reactive intermediates. This imbalance can lead to cellular damage and has been implicated in a multitude of health conditions, including cancer, neurodegenerative disorders, and cardiovascular diseases. Recognizing how oxidative stress operates allows scientists to analyze its role in health and disease more effectively.
The measurement of oxidative stress provides valuable insight into the cellular response to various stressors, whether they are external factors like pollution or internal factors related to metabolism. By understanding how cells react to oxidative stress, researchers can better identify potential therapeutic targets or preventive measures to mitigate damage.
Implications for Research
Incorporating oxidative stress assays into scientific inquiry has several benefits. Firstly, these assays enable quantification and characterization of oxidative stress in diverse systems, ranging from cell cultures to animal models. This versatility enhances the potential for groundbreaking discoveries in various fields, such as medical research and environmental studies.
Furthermore, oxidative stress assays can aid in the evaluation of new pharmaceuticals that combat oxidative damage. By assessing the efficacy of these drugs in reducing oxidative stress, researchers gain insights into their mechanisms of action and potential applications in clinical settings.
Considerations in Understanding Oxidative Stress
While the benefits of oxidative stress assays are profound, researchers must also acknowledge the complexities inherent in measuring oxidative stress. Variability in assay results can arise from differences in experimental conditions, sample types, and detection methods. Developing standardized protocols is essential for producing reliable data that can contribute meaningfully to scientific literature.
Additionally, the interpretation of results from oxidative stress assays requires careful consideration. Factors affecting ROS levels may differ significantly between individuals, making it crucial for researchers to contextualize findings within specific biological and environmental frameworks.
"Understanding oxidative stress is vital for advancing scientific knowledge and clinical applications that can improve human health."
In summary, the introduction to oxidative stress lays the groundwork for a comprehensive exploration of oxidative stress assays throughout this article. By addressing the importance, implications, and considerations of oxidative stress, readers will be well-prepared to delve into detailed discussions on assay types, methodologies, and applications. This foundational understanding will ultimately engage students, researchers, educators, and professionals in informed discussions on oxidative stress's role in science.
Understanding Oxidative Stress and Its Implications
Understanding oxidative stress and its implications is fundamental in the field of biological research. Oxidative stress refers to an imbalance between reactive oxygen species (ROS) production and the body's ability to eliminate them or repair the resulting damage. This balance is crucial for maintaining cellular function and overall health. When oxidative stress occurs, it can lead to various cellular and molecular disruptions, with ramifications in numerous diseases, including cancer, cardiovascular disorders, and neurodegenerative conditions.
The significance of studying oxidative stress lies in its pervasive impact on human health. Researchers explore oxidative stress not only to comprehend these pathological processes but also to devise therapeutic interventions. The intricacies involved in oxidative stress underpin the rationale for rigorous assay development. These assays are essential for quantifying oxidative stress levels, which can provide insight into cellular responses, underlying causes of diseases, and potential therapeutic targets.
In essence, understanding oxidative stress allows scientists to delve deeper into how cells cope with environmental changes and physiological stressors. This has far-reaching implications for both basic science and applied research. Effective oxidative stress assays are instrumental in enabling researchers to draw connections between oxidative damage and disease processes, thereby fostering advancements in preventive and therapeutic strategies.
"Oxidative stress is not merely a consequence but a central player in the pathology of numerous diseases. Investigating its implications can reveal potential pathways for intervention."
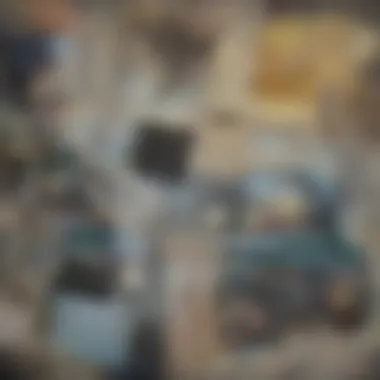
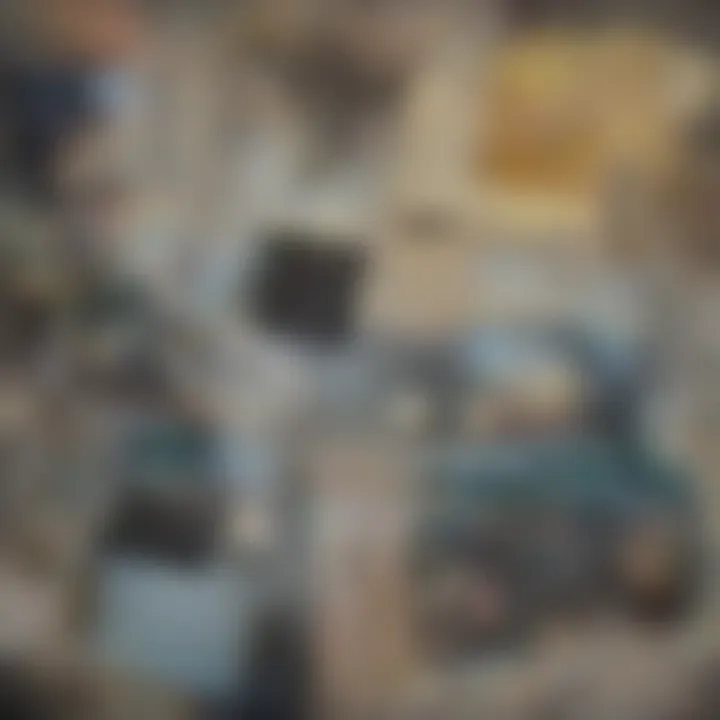
Definition of Oxidative Stress
Oxidative stress can be defined as an imbalance between ROS and antioxidants in the biological system. Reactive oxygen species are unstable molecules that can damage cellular components like DNA, proteins, and lipids. This instability leads to oxidative damage, which can overwhelm the body's defense systems, primarily composed of antioxidants. The body’s antioxidants include enzymes like superoxide dismutase and glutathione peroxidase, as well as non-enzymatic molecules like vitamin C and E. When ROS levels exceed antioxidant capacity, oxidative stress ensues, resulting in detrimental alterations to cellular structures and functions.
The concept of oxidative stress is vital because it highlights the dual role of ROS as both harmful by-products of metabolism and, under certain conditions, essential signaling molecules. Thus, understanding oxidative stress encompasses not only the detrimental effects but also the physiological roles of ROS, which is an evolving area of research.
Biochemical Mechanisms Involved in Oxidative Stress
Biochemical mechanisms of oxidative stress involve multiple pathways that lead to ROS formation and subsequent cellular damage. One primary source of ROS is through normal metabolic activities, primarily in mitochondria during aerobic respiration. Here, incomplete reduction of oxygen can lead to the production of free radicals.
Other mechanisms include:
- Inflammation: Phagocytes generate ROS as part of the immune response to eliminate pathogens, which can inadvertently cause damage to surrounding tissues.
- Environmental Factors: Exposure to UV radiation, pollution, and toxins can also increase ROS production.
- Impaired Antioxidant Defense: Factors like aging or nutritional deficiencies can lead to reduced antioxidant levels in the body, exacerbating oxidative stress.
These mechanisms highlight that oxidative stress results from a complex interplay of both endogenous and exogenous factors, further emphasizing the importance of robust assays to measure its effects.
Sources of Reactive Oxygen Species
The sources of reactive oxygen species are diverse, ranging from normal cellular processes to external factors. Understanding these sources is critical for mitigating oxidative stress and researching its effects.
Common sources include:
- Mitochondrial Respiration: The primary source of ROS production. Superoxide is generated as a by-product of electron transport.
- Enzymatic Reactions: Certain enzymes, such as xanthine oxidase and nitric oxide synthase, produce ROS during metabolic reactions.
- Environmental Stress: Exposure to radiation, heavy metals, and pollutants can induce ROS generation in cells.
- Inflammatory Responses: Immune cells produce ROS to combat pathogens but can also contribute to inflammatory diseases.
Understanding these sources is crucial for designing effective oxidative stress assays. By identifying the specific origins of ROS, researchers can better tailor their studies to address the impacts of oxidative stress across different fields of study.
Types of Oxidative Stress Assays
The examination of oxidative stress encompasses a variety of experimental approaches. Different types of assays play crucial roles in advancing our understanding of oxidative processes in cells. Each assay offers unique insights, with specific methodologies tailored to answer particular scientific questions. In essence, the choice of assay depends on the type of sample, the target molecules of interest, and the desired level of sensitivity.
In Vitro Assays
In vitro assays are designed to study oxidative stress in controlled environments outside of living organisms. These experiments typically utilize cell cultures, allowing researchers to manipulate and observe cellular responses to oxidative agents directly.
They are important for understanding cellular mechanisms underlying oxidative stress. Some common in vitro assays include the use of hydrogen peroxide to induce oxidative stress, followed by the measurement of cellular viability or specific markers that indicate oxidative damage.
The benefits of in vitro assays include:
- Controlled environments that minimize variability
- Cost-effectiveness compared to in vivo models
- The ability to test specific hypotheses about oxidative stress pathways
However, in vitro studies may not always accurately reflect physiological conditions. Careful consideration must be given to how results translate to living systems.
In Vivo Assays
In vivo assays involve the assessment of oxidative stress within living organisms. These methods provide a more comprehensive view of how oxidative processes affect systemic physiology. Animal models, such as mice or rats, are often utilized to explore various diseases associated with oxidative stress.
The advantages of in vivo assays are numerous:
- They account for complex interactions between different biological systems
- They can reveal the overall metabolic state and response to oxidative stress
- They aid in studying the efficacy of potential therapeutic interventions
Nonetheless, in vivo assays also come with challenges. The ethical considerations surrounding animal research must be handled effectively, and the results can be influenced by external factors in the physiological environment.
Enzymatic Assays
Enzymatic assays leverage the activity of specific enzymes that respond to oxidative stress. This method is significant because many enzymes, like superoxide dismutase and catalase, directly interact with reactive oxygen species (ROS). Subsequently, by measuring these enzymatic activities, researchers can infer the oxidative stress levels in the biological sample.
Key points of enzymatic assays include:
- High specificity for targets, enabling precise measurements of oxidative damage
- Ability to track changes over time in response to treatments or external factors
- Insight into cellular antioxidant defenses
While enzymatic assays offer specific insights, they may also be limited in scope. Factors like enzyme availability, stability, and interactions can affect the observed data.
Fluorometric and Colorimetric Techniques
Fluorometric and colorimetric techniques are widely used to detect oxidative stress biomarkers within samples. These assays involve the use of fluorescent or colored compounds that react with oxidative products, leading to measurable changes in fluorescence or color intensity. Methods such as the DCFH-DA assay for measuring intracellular ROS are common examples.
Benefits of fluorometric and colorimetric techniques include:
- High sensitivity and specificity, allowing detection at low concentrations
- Quick results, facilitating timely analysis
- Adaptable to high-throughput screening
However, users must be cautious of interference from other substances in the sample. Additionally, ensuring the proper calibration of instruments is vital for obtaining reliable results.
Each type of oxidative stress assay contributes valuable data towards understanding biological responses and disease mechanisms. Choosing the appropriate assay is essential for acquiring accurate and relevant information.
Methodology of Oxidative Stress Assays
The methodology of oxidative stress assays is critical for obtaining reliable and relevant data in scientific research. Each step in the assay process affects the quality of the results. Understanding the methodology allows researchers to optimize conditions for accurate measurements of oxidative stress, which is essential for linking oxidative damage to various biological processes and diseases. This section will cover three key components: sample preparation, detection techniques, and data analysis and interpretation.
Sample Preparation
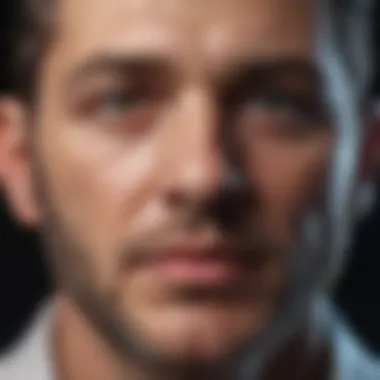
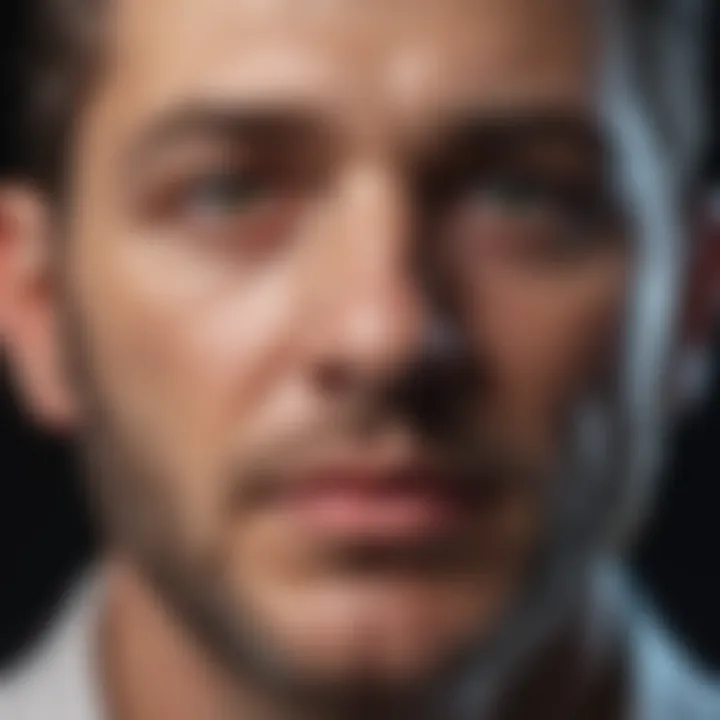
Sample preparation is the preliminary but essential step in oxidative stress assays. Proper preparation affects the overall outcome and reliability of the assays. Samples may include biological fluids like serum, plasma, or tissue homogenates. The goal is to maintain the integrity of reactive oxygen species while minimizing their degradation during the handling process.
- Selection of Samples: Researchers must choose appropriate samples based on their research objectives. For instance, blood samples can reveal systemic oxidative stress, while tissue samples may provide localized insights.
- Handling and Storage: To mitigate additional oxidative damage before analysis, samples should be kept on ice and processed promptly. If storage is necessary, freezing at -80°C is ideal for preserving sample integrity.
- Homogenization: For tissue samples, homogenization is key. It helps to release all cellular components, ensuring accurate measurement. The type of buffer used can influence the assay results, thus it is essential to choose a compatible one.
Detection Techniques
Detection technology is the backbone of oxidative stress assays. The choice of technique depends on the specific reactive oxygen species being measured, the sensitivity required, and the type of sample used.
- Fluorometric Techniques: These methods utilize specific fluorescent probes that react with reactive oxygen species. The increase in fluorescence correlates with the level of oxidative stress, providing quantitative results.
- Colorimetric Techniques: These assays rely on color changes resulting from the reaction of oxidative products with specific reagents. This method is often easy to perform, making it suitable for routine analyses.
- Enzymatic Assays: Enzymatic assays measure the activity of antioxidant enzymes, providing insight into the body’s response to oxidative stress. The assays often target specific enzymes like superoxide dismutase or catalase, revealing how effectively the body is combating oxidative damage.
"The choice of detection technique is paramount, as it determines the sensitivity and specificity of the assay results."
Data Analysis and Interpretation
The final step in oxidative stress assays is data analysis and interpretation. This step involves translating raw data into meaningful conclusions regarding oxidative stress levels.
- Statistical Analysis: Utilizing statistical tools is essential for reviewing assay results. An appropriate statistical test can confirm whether observed differences in oxidative stress are significant.
- Comparative Analysis: A comparative approach allows researchers to assess oxidative stress levels across different conditions or treatments. This can lead to insights about how certain interventions may mitigate oxidative damage.
- Biological Relevance: Finally, interpreting the data within the context of existing literature is crucial. Researchers should connect their findings to broader biological implications, considering how oxidative stress may influence various diseases or biological processes.
In summary, the methodology of oxidative stress assays is a crucial component in scientific research. With careful attention to sample preparation, accurate detection techniques, and thorough data analysis, researchers can gain substantial insights into the role of oxidative stress in health and disease.
Applications of Oxidative Stress Assays
Oxidative stress assays are crucial in the realm of scientific research. They serve multiple functions across various fields, enabling researchers to evaluate the effects of oxidative stress on biological systems. Understanding how these assays are applied can illuminate their significance in medical research, pharmaceutical development, and environmental studies. In this section, we will explore these applications in depth, providing insights into how oxidative stress assays contribute to advancements in these critical areas.
Medical Research
In medical research, oxidative stress assays play a vital role in elucidating the pathophysiology of numerous diseases. Conditions such as diabetes, cancer, and neurodegenerative disorders have been linked to oxidative stress. By measuring oxidative stress levels in patient samples, researchers can identify biomarkers that indicate disease progression or response to treatment. This can facilitate early diagnosis and tailored therapies, ultimately leading to improved patient outcomes.
Furthermore, oxidative stress assays can help assess the efficacy of antioxidant therapies. For instance, clinical trials may utilize these assays to measure changes in oxidative stress markers during treatment with substances like vitamin E or coenzyme Q10.
"Oxidative stress assays enhance our understanding of disease mechanisms, directly impacting patient care and therapeutic strategies."
Pharmaceutical Development
Pharmaceutical companies increasingly rely on oxidative stress assays during the drug development process. These assays provide key data regarding the safety and efficacy of potential medications. Understanding how a drug affects oxidative stress levels can help predict its therapeutic potential and adverse effects.
Moreover, oxidative stress assays inform the development of nutraceuticals. For example, supplements aimed at reducing oxidative stress are gaining popularity. By employing assays to screen these products, researchers can ensure that they possess the desired effects on oxidative balance. This is particularly important as consumers become more health-conscious and seek scientifically-backed products.
Environmental Studies
Environmental research has emerged as another significant area for oxidative stress assays. These assays allow scientists to assess the impact of environmental pollutants on living organisms. For instance, studies examining the effects of heavy metals or pesticides often utilize oxidative stress assessments to determine how these substances affect cellular integrity.
Additionally, the relationship between oxidative stress and climate change is garnering attention. Studies are investigating how increased temperatures and changing conditions may exacerbate oxidative stress in various species, prompting shifts in ecosystems and biodiversity.
In summary, the applications of oxidative stress assays extend from individual health to global environmental concerns. The insights gained from these assays continue to shape research directions and therapeutic innovations. Their relevance across diverse fields underscores the importance of further advancements in assay techniques.
Oxidative Stress and Disease Correlation
Oxidative stress is increasingly recognized as a critical factor in the development and progression of various diseases. Understanding this correlation is essential, as it guides research towards innovative treatment strategies. Several diseases show notable connections to oxidative stress, making it imperative to explore these relationships comprehensively. Through examining oxidative stress, scientists can potentially identify new biomarkers and therapeutic targets that may improve patient outcomes.
Role in Cancer
The link between oxidative stress and cancer is multifaceted. Reactive oxygen species (ROS), the byproducts of oxidative stress, can cause damage to DNA, proteins, and lipids. This damage is a precursor to mutations that lead to cancerous changes in cells. Research has shown that the accumulation of oxidative stress contributes not only to the initiation of cancer but also to its progression and metastasis. The intricate balance of oxidation and antioxidation within cells is crucial in determining whether cells thrive or succumb to dysfunction.
Furthermore, some cancer treatments aim to exploit this vulnerability by increasing oxidative stress to levels detrimental to cancer cells. For example, chemotherapeutic agents such as doxorubicin generate ROS, thereby targeting cancer cells more aggressively while sparing normal cells. Understanding oxidative stress’s role in cancer encourages the exploration of synergistic treatments that can maximize therapeutic effects while minimizing side effects.
Impact on Neurodegenerative Diseases
Neurodegenerative diseases such as Alzheimer's, Parkinson's, and Huntington's disease show significant associations with oxidative stress. Elevated ROS levels can lead to neuronal damage and are implicated in the neuroinflammatory process that characterizes these conditions. The accumulation of oxidative damage in neuronal tissues is a common feature across multiple neurodegenerative disorders.
For example, in Alzheimer's disease, oxidative stress is believed to enhance tau phosphorylation and amyloid-β aggregation, two hallmark features of the disease. Investigating antioxidant therapy has emerged as a therapeutic strategy intended to mitigate the effects of oxidative stress. Some studies suggest that compounds such as curcumin or resveratrol may hold promise in protecting neuronal integrity and function. Hence, understanding these connections not only aids in improving current therapeutic options but also pushes for novel treatment strategies that center on combating oxidative stress.
Cardiovascular Effects
The cardiovascular system is highly sensitive to oxidative stress due to its reliance on proper endothelial function and vascular tone. Oxidative stress contributes to atherosclerosis by promoting inflammation, endothelial dysfunction, and plaque formation. Increased oxidative damage to lipoproteins, particularly low-density lipoprotein (LDL), can facilitate the development of atherosclerotic lesions.
Moreover, conditions such as hypertension, heart failure, and ischemic heart disease are also influenced by the balance between oxidants and antioxidants. Studies show that antioxidants such as vitamin E and vitamin C can have a protective effect against cardiovascular diseases, reinforcing the importance of oxidative stress in cardiac health. Ultimately, understanding these relationships emphasizes the need for ongoing research into antioxidant supplementation and lifestyle changes that may mitigate harmful oxidative effects on cardiovascular health.
Implications in Metabolic Disorders
Metabolic disorders, including obesity and diabetes, are closely linked to oxidative stress. Elevated levels of ROS in adipose tissue can lead to insulin resistance and contribute to the development of type 2 diabetes. The proposed mechanism involves oxidative stress affecting signaling pathways essential for insulin function, which can exacerbate metabolic dysregulation.
Oxidative stress also promotes inflammation in metabolic tissues, further complicating the clinical picture. Strategies aiming to lower oxidative stress, such as dietary improvements or weight management, have shown benefits in blood glucose control and overall metabolic health. Furthermore, examining the interplay between oxidative stress and metabolic disorders is vital for developing comprehensive management plans that include both dietary and pharmacological interventions.
The correlation between oxidative stress and disease opens avenues for novel therapeutic approaches. Understanding these dynamics can lead to better prevention and treatment strategies across various fields of medicine.
Challenges in Oxidative Stress Research
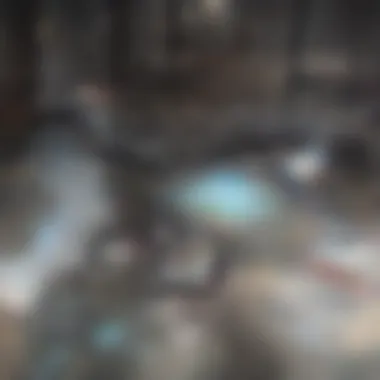
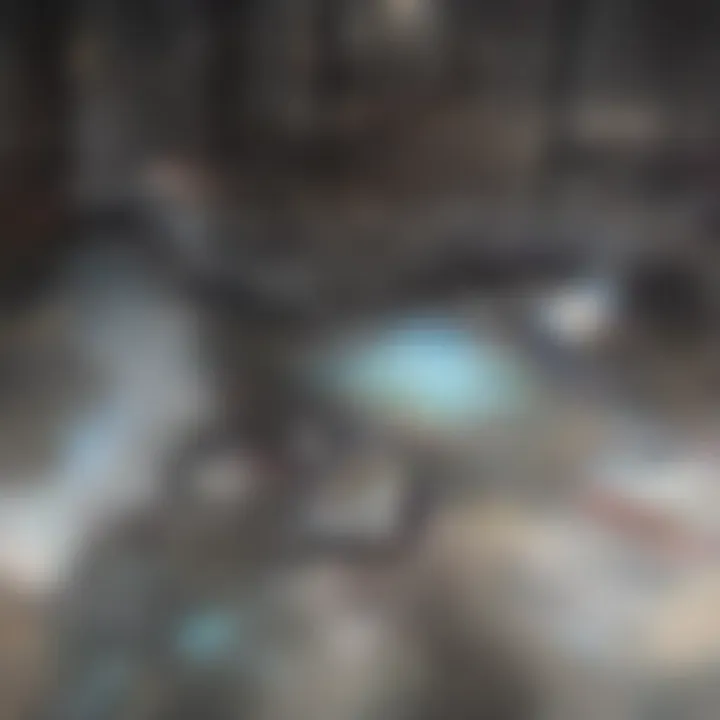
The study of oxidative stress presents numerous challenges that can hinder the process and outcomes of scientific research. Understanding these challenges is crucial because they fundamentally affect the reliability and validity of data gathered through various oxidative stress assays. It is essential to recognize the nuances behind these issues as they can impact the interpretation of results in both basic and applied research settings.
Variability and Standardization Issues
One major challenge in oxidative stress research is the variability in results due to a lack of standardization across different laboratories. Each research facility may employ slightly different methodologies, sample handling procedures, and assay techniques. This leads to discrepancies in how data is interpreted and reported. Such variability can stem from:
- Different types of assays employed.
- Variations in reagents or equipment.
- Environmental conditions during experimentation.
This inconsistency creates difficulty in comparing results across different studies, which can lead to conflicting conclusions in the literature. It raises concerns about the reproducibility of findings and complicates meta-analyses, a critical tool for consolidating knowledge in the scientific community. Standardizing procedures and reagents could improve comparability and enhance the reliability of oxidative stress research.
Limitations of Current Assay Techniques
Current assays for measuring oxidative stress have their own limitations that must be acknowledged. A primary issue is the potential for interference from other biomolecules present in biological samples. This can result in:
- False positives or negatives in oxidative stress measurements.
- Inconsistencies in the detection thresholds of reactive oxygen species.
Additionally, many techniques fall short regarding sensitivity and specificity. For instance, some assays may not differentiate between various types of reactive oxygen species or fail to account for antioxidant levels adequately, leading to skewed representational data.
Moreover, the complexity of biological systems often causes challenges. Oxidative stress does not occur in isolation; its effects are interlinked with numerous cellular processes. Current assays may not capture this complexity sufficiently, which oversimplifies the interpretation of data.
Recent Advancements in Oxidative Stress Assays
Oxidative stress plays a crucial role in various biological processes, significantly influencing health and disease outcomes. Recent advancements in oxidative stress assays help researchers understand its effects more precisely. New techniques and improved technologies enable more accurate and sensitive measurement of oxidative stress levels in various biological contexts. This section discusses these advancements and their implications for scientific research.
Innovative Techniques and Technologies
The field of oxidative stress research has seen a surge in innovative techniques. For example, advances in mass spectrometry have heightened the ability to detect reactive oxygen species (ROS) at much lower concentrations than before. This allows researchers to explore oxidative stress in real-time within living organisms.
Nanotechnology is another area that has transformed oxidative stress assays. Nanoparticles are used as delivery systems for antioxidants, enhancing the study of their efficacy against oxidative stress. Techniques like microfluidics further improve assay sensitivity by allowing for the detection of oxidative products in minimal sample volumes.
Modern techniques are making assays more accessible and efficient, empowering researchers.
Additionally, biosensors that adapt to changes in redox states provide dynamic feedback on oxidative stress levels. These developments not only enhance the sensitivity of assays but also allow for continuous monitoring over time.
Progress in Biomarkers Identification
Identifying specific biomarkers linked to oxidative stress is essential for understanding the underlying mechanisms of associated diseases. The development of new assays has led to the discovery of numerous biomarkers, facilitating more accurate assessments.
Isoprostanes are one such example; they are reliable indicators of lipid peroxidation. They provide insight into oxidative damage in various diseases like cardiovascular diseases and neurodegenerative disorders. Other biomarkers, such as 8-hydroxydeoxyguanosine, contribute to understanding DNA damage resulting from oxidative stress.
Moreover, proteomics and metabolomics have expanded the identification of biomarkers. Advanced mass spectrometry and data analysis techniques have led to the discovery of a wider spectrum of oxidative stress-related molecules. The integration of these findings into study designs provides valuable context for therapeutic interventions and disease prevention strategies.
In summary, advancements in both the technologies used and the identification of biomarkers are pivotal for the evolution of oxidative stress assays. These progressions not only enhance the reliability of the assays but also expand their applications across various fields, from medicine to environmental studies.
Future Directions in Oxidative Stress Studies
Exploring the future directions in oxidative stress studies is essential for advancing scientific research. The increasing awareness of oxidative stress’s impact on health and disease underlines the need for more refined techniques and approaches. This section will discuss two significant areas: integration with other research fields and potential clinical applications. Each area offers unique benefits, implications, and considerations that could enhance our understanding and treatment of oxidative stress-related conditions.
Integration with Other Research Fields
Integrating oxidative stress research with other scientific domains can yield comprehensive insights. For instance, merging findings with genetic studies allows for a deeper understanding of how oxidative stress interacts with genetic predispositions to various diseases. Additionally, linking oxidative stress assays with studies in nutrition can reveal how different diets affect oxidative stress levels, providing valuable information for dietary recommendations.
Furthermore, collaboration with fields like molecular biology can help clarify the mechanisms behind reactive oxygen species and their roles in the cellular environment. This synergy can lead to innovative approaches in therapy, where targets in oxidative stress pathways can be identified and modulated more accurately.
- Benefits of Integration:
- Enhanced understanding of diseases' etiology.
- Development of multi-faceted treatment strategies.
- Collaboration can accelerate discoveries.
Thus, this integration of fields helps create a more holistic view of oxidative stress, leading to potent strategies for intervention.
Potential for Clinical Applications
The clinical implications of oxidative stress studies are profound. As we refine oxidative stress assays, we enhance their applicability in clinical settings. This can facilitate early diagnosis and monitoring of diseases such as cancer, cardiovascular diseases, and neurodegenerative disorders.
- Clinical Uses of Oxidative Stress Assays:
- Biomarker for disease progression.
- Assessment of treatment efficacy.
- Risk stratification in patient populations.
As we explore disease states more deeply, these assays can be tailored to assess oxidative stress markers specific to certain conditions. Moreover, their role in therapeutic monitoring is crucial. Tracking changes in oxidative stress levels can help gauge how well patients respond to treatments, steering clinical decision-making.
In summary, the potential for clinical applications of oxidative stress assays is vast. They promise improved diagnostics and personalized medicine approaches, enhancing overall patient care.
The End
The conclusion of this article underscores the significance of oxidative stress assays in scientific research, highlighting their multifaceted roles and applications across various disciplines.
Oxidative stress assays represent a vital tool for understanding cellular mechanisms and their implications in health and disease. These assays enable researchers to quantify the levels of reactive oxygen species, assess antioxidant defenses, and evaluate the oxidative status of biological systems. Through these measurements, researchers can gain insights into how oxidative stress contributes to the pathogenesis of various diseases, including cancer, cardiovascular disorders, and neurodegenerative diseases.
Key points discussed in the article include:
- The various types of oxidative stress assays, including in vivo and in vitro settings, which cater to different experimental conditions and objectives.
- Methodologies that are critical for ensuring reliable results, such as sample preparation and data interpretation, which ultimately dictate the validity of findings.
- The challenges that persist in this area of study, emphasizing the need for standardization and the improvement of existing techniques. This consideration is crucial for comparing results across different studies and making meaningful interpretations.
- Recent advancements in assay technologies and biomarker identification that promise to enhance the accuracy and efficacy of oxidative stress measurement.
- Future directions that integrate oxidative stress research with other scientific disciplines, opening avenues for novel clinical applications and therapeutic strategies.