Understanding Atomic Force Microscopy Principles
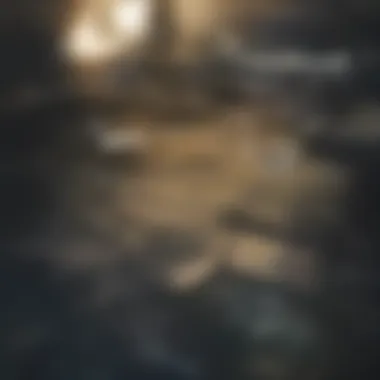
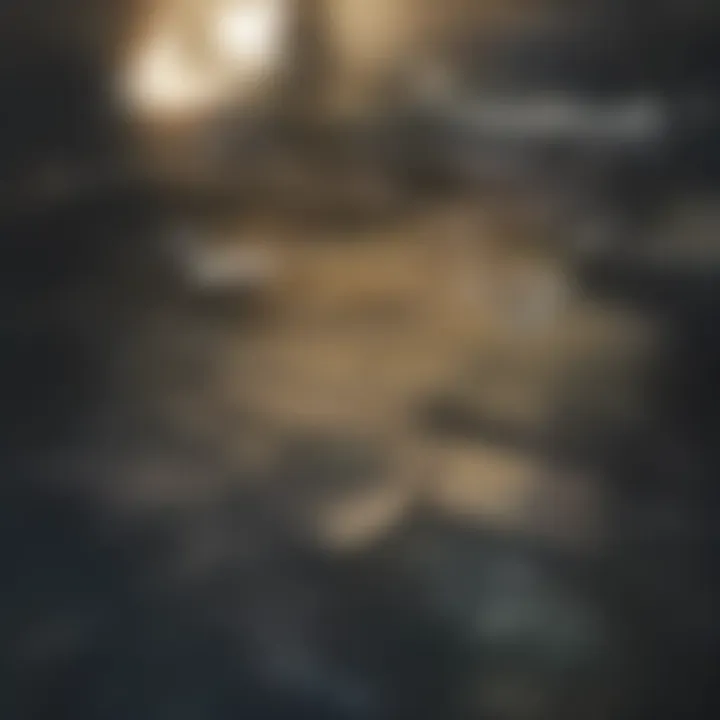
Intro
Atomic Force Microscopy (AFM) is a highly effective tool that allows scientists to visualize and manipulate materials at the atomic level. It stands at the intersection of physics, chemistry, and materials science, promoting advancements in nanotechnology. This technique empowers researchers to move beyond traditional optical methods, providing unparalleled insight into the surface characteristics of nanoscale materials.
AFM operates through a simple yet ingenious principle: a sharp probe scans the surface of a sample. As the probe interacts with the surface, it measures forces between the probe and the sample, producing detailed topographical maps. This non-destructive method is key for analyzing various materials, guiding numerous applications in research and industry.
Understanding the principles behind AFM not only enhances its usage but also sheds light on its importance in contemporary scientific discovery. Key findings reveal how this technique outpaces others, providing compelling advantages but also posing challenges that users must navigate. Let's delve into the significant aspects of this remarkable imaging technique.
Key Findings
- Summary of the Main Results: The core attributes of Atomic Force Microscopy include its ability to provide high-resolution images and measure surface forces. AFM can resolve features down to the nanometer scale, making it indispensable for studying biological samples, polymers, and semiconductors. The methodology enables users to attain information about surface roughness, mechanical properties, and even electrical characteristics.
- Significance of Findings Within the Scientific Community: AFM has reshaped the landscape of material characterization. Its contribution is profound, facilitating advancements in fields such as materials science, biology, and nanotechnology. Researchers are now able to uncover characteristics previously unattainable with conventional techniques, expanding knowledge and applications of nano-materials.
"The ability to visualize nanoscale structures not only drives innovation but also opens new avenues for technology development in various fields".
Implications of the Research
- Applications of Findings in Real-World Scenarios: The implications of AFM extend widely into practical applications. In nanotechnology, AFM aids in developing nanostructures crucial for electronics, drug delivery systems, and biomaterials. Furthermore, its capability to function in various environments—such as liquid, air, or vacuum—allows for versatile usage across chemical and biological domains.
- Potential Impact on Future Research Directions: As researchers harness the potential of AFM, its role in future discovery is expected to grow. This technique enables the study of new materials and their interactions, laying the groundwork for breakthroughs in quantum computing, energy storage, and medical diagnostics. Therefore, understanding AFM is not just an academic exercise; it’s a stepping stone for future technological advancements.
Exploring Atomic Force Microscopy reveals a glimpse into the future of material science and its transformative potential, underscoring its vital role in advancing research across multiple disciplines.
Preamble to Atomic Force Microscopy
Atomic Force Microscopy (AFM) represents a significant advancement in the field of microscopy, allowing researchers to visualize nanoscale structures with exceptional clarity. The rising importance of AFM stems from its unique ability to provide three-dimensional surface profiles of materials at the atomic level. This opens up new avenues for understanding the properties of materials and biological specimens in ways previously unattainable.
The reason AFM has gained such traction in scientific research is largely due to its versatility. It can be employed in various disciplines, such as materials science, biology, and nanotechnology. Researchers can use AFM to study surface roughness, assess mechanical properties, and even observe dynamic processes in real-time.
Moreover, AFM does not require extensive sample preparation, which enhances its practicality in many research settings. It can operate in different environments, including air and liquid, thus making it suitable for a broad range of applications.
Understanding AFM is crucial for any scientist working at the nanoscale, since this technique aids in the exploration of fundamental principles of matter. Its ability to generate high-resolution images stems from its interaction with surface forces, setting it apart from traditional optical microscopy. In the sections that follow, we will discuss various microscopy techniques, the significance of AFM in scientific research, its operating principles, and its practical applications which demonstrate why it has become a cornerstone of contemporary microscopy.
Principle of Operation
The principle of operation is a foundational aspect of Atomic Force Microscopy. Understanding how AFM works aids in its proper application across many scientific fields. It delves deep into the working mechanisms, providing insight into how materials are visualized and manipulated at the nanoscale. By grasping these principles, researchers can make informed decisions on utilizing AFM based on specific requirements.
Basic Working Mechanism
The basic working mechanism of AFM relies on a probe that scans the surface of a sample. This probe is attached to a cantilever, which bends when forces between the probe and the surface come into play. The bending of the cantilever is then measured to derive surface topography. It is important to understand that AFM does not require light, making it distinct from other microscopy techniques.
The probe tip can approach very close to the sample, allowing for high-resolution imaging. The AFM captures various types of surface interactions leading to a detailed depiction of the material surface. This mechanism enables the examination of materials in a range of environments, including air and liquid. The ability to work in multiple environmental conditions is a significant benefit.
Interaction Forces in AFM
Interaction forces play a crucial role in how AFM operates. These forces determine the probe's behavior as it approaches the sample. Understanding these forces is essential for successful imaging.
Van der Waals Forces
Van der Waals forces are weak intermolecular forces that become significant at short distances. In the context of AFM, these forces are important because they allow the probe to interact with the sample at nanoscale distances. The key characteristic of Van der Waals forces is that they can create a measurable signal even when the probe is not touching the surface.
This feature makes them a beneficial aspect of AFM because it allows for imaging in a non-contact mode, avoiding potential damage to the sample. However, the weakness of these forces can also be a disadvantage, as they may limit the resolution in certain conditions
Capillary Forces
Capillary forces arise due to the presence of liquid films on the surfaces. These forces contribute significantly when the AFM operates in ambient conditions that are not perfectly dry. A key characteristic of capillary forces is their strength in attracting the probe to the surface, which can assist in providing clearer images.
In the context of AFM, capillary forces can be a double-edged sword. While they enhance the interaction with the sample, they can also lead to unwanted effects like droplet formation, which can affect data quality. Understanding this balance is crucial for obtaining accurate results.
Electrostatic Forces
Electrostatic forces are important when dealing with conductive materials. They arise from the charged surface of the sample or the probe. The key characteristic of electrostatic forces is their sensitivity to charge densities. This allows AFM to identify surface charges and potential differences on materials.
Electrostatic forces are a popular choice in AFM due to their ability to provide additional information about the sample surface. However, the challenge lies in ensuring that the sample does not get damaged due to excessive force from the probe, requiring careful calibration during operation.
In summary, the principle of operation in AFM is intertwined with various interaction forces that dictate the system's performance. A thorough understanding of these forces enhances the effective application of AFM in research and technological innovations.
Components of an AFM System
The functionality of Atomic Force Microscopy (AFM) heavily relies on its components. Understanding these parts is crucial to grasp how AFM operates effectively. Each component plays a key role in generating high-resolution images and collecting precise data.
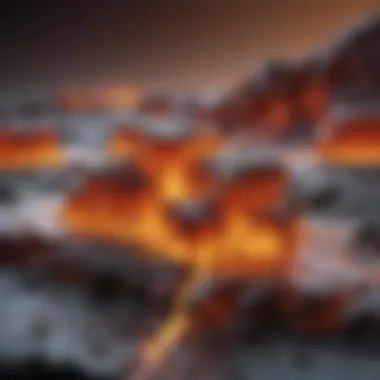
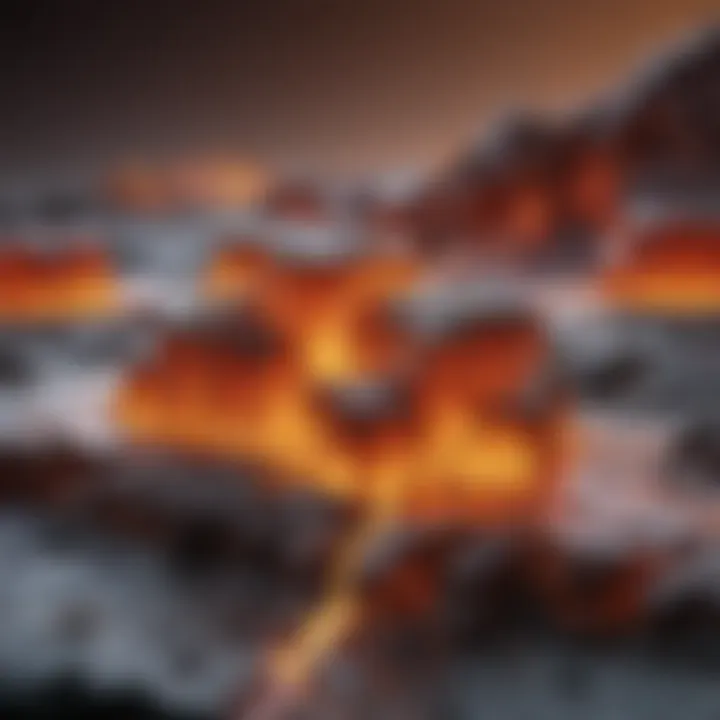
Cantilever and Tip
The cantilever and tip are fundamental to the AFM system. The cantilever acts as a lever, with the tip positioned at its end. This setup allows the system to scan surfaces with extreme precision.
Design Considerations
When considering design for the cantilever, flexibility and spring constant are critical aspects. A cantilever that is too stiff will have difficulties in measuring delicate surfaces, while one that is too flexible may cause instability during scanning. Thus, the optimum spring constant ensures proper interaction with the sample under investigation. A common characteristic of well-designed cantilevers is minimal thermal noise, which contributes to better resolution in imaging.
Moreover, the design intricacies such as length and thickness influence its behavior. Longer cantilevers tend to be more sensitive, while thickness can affect robustness. Balancing these factors gives AFM systems higher performance levels, making them versatile tools.
Material Selection
The material selection for cantilevers significantly impacts their operational capabilities. Silicon is one of the most used materials due to its mechanical properties and availability. It provides an excellent balance between strength and flexibility. An alternate choice is silicon nitride, which offers higher stiffness and less thermal drift. The unique feature of silicon nitride is its ability to operate under harsher conditions without degradation. However, such materials can also result in higher costs. Therefore, selecting the right material is pivotal, depending on the specific needs of the research.
Drive Mechanisms
Drive mechanisms are integral in the operation of AFM systems. They control the movements of the cantilever and tip. Piezoelectric actuators are commonly utilized in this context. These actuators transform electric signals into controlled movements, allowing for meticulous scanning and positioning. Their precision plays a decisive role in achieving high-resolution images. The design efficiency of drive mechanisms thus has direct consequences on image clarity and operational success.
Detection Systems
Detection systems in AFM track the deflection of the cantilever. They are vital for interpreting the data obtained during imaging. Two prominent detection methods are optical and piezoelectric detection.
Optical Detection
Optical detection is a widely used method due to its high sensitivity and ease of integration. It typically employs a laser beam directed onto the back of the cantilever. Any deflection results in a measurable shift of the laser spot on a photodetector. This method’s key characteristic is its capability to detect very small movements with high accuracy, thus capturing intricate nanoscale features. Its versatility makes it a popular choice in various AFM applications.
Piezoelectric Detection
On the other hand, piezoelectric detection systems utilize piezoelectric materials to sense cantilever deflection. This method is distinct in its ability to provide direct electrical signals proportional to the cantilever’s movement. A significant advantage of piezoelectric detection is its robustness in various settings. However, it might not achieve the same level of sensitivity as optical detection under certain conditions. Each detection system presents its own set of strengths and limitations, making the choice dependent on the application requirements.
In summary, the components of an AFM system are crucial in their operation, with each part contributing to the overall effectiveness of the technique for imaging and analysis.
Imaging Modes in AFM
The imaging modes in Atomic Force Microscopy (AFM) are fundamental to how the technique captures detailed information about a sample at the nanoscale. Each mode has its unique principles of operation and specific applications, making it crucial for optimizing imaging based on the desired outcomes. Understanding the various imaging modes allows researchers to select the most effective approach for their material or biological specimen, leading to better data quality and insights.
Contact Mode
Contact mode is one of the earliest and most straightforward imaging modes utilized in AFM. In this mode, the tip of the cantilever makes direct contact with the sample surface during scanning. The interaction forces between the tip and sample influence the deflection of the cantilever, which is monitored as the feedback mechanism to create a topographical map of the surface. One of the major advantages of contact mode is its ability to provide high-resolution images at a relatively fast scanning rate. However, this mode can also lead to sample damage or alteration, particularly in soft materials.
It is important for users to consider the following points when utilizing contact mode:
- Material Sensitivity: Delicate samples may not withstand the applied forces during imaging, which can cause deformation.
- Utilization Environment: A stable environment is necessary to avoid external interference that could distort results.
- Image Artifacts: Careful calibration and tuning are essential to minimize artifacts caused by tip-sample interactions.
Tapping Mode
Tapping mode, also recognizable as intermittent contact mode, offers a middle ground between contact and non-contact mode. In this approach, the tip oscillates near its resonant frequency while making brief contact with the sample's surface during the downward swing of the oscillation. This results in reduced lateral forces compared to contact mode, effectively reducing the risk of sample damage. Tapping mode is particularly advantageous for imaging biological samples and soft materials, providing high-resolution imaging without compromising the integrity of the specimen.
Key considerations when using tapping mode include:
- Resonance Frequency: Proper tuning of the cantilever oscillation increases imaging quality and resolution.
- Sample Properties: Certain materials may still experience unwanted alterations, even in tapping mode, therefore selection is crucial.
- Environmental Control: As in contact mode, maintaining a stable environmental condition aids in achieving optimal results.
Non-Contact Mode
Non-contact mode operates with the AFM tip oscillating above the sample surface, without making actual contact. Instead, it senses the attractive forces, such as Van der Waals forces, between the tip and sample. This mode is advantageous when it comes to imaging soft or delicate samples, as there is minimal interaction that could cause damage. \nNon-contact mode can achieve high spatial resolution, although it is generally slower compared to the other imaging modes.
Some important elements to observe in non-contact mode are:
- Distance Control: Proper adjustment is vital for maintaining an effective distance to enhance resolution while avoiding tip-sample collisions.
- Sensitivity to Vibrations: Non-contact mode can be more susceptible to environmental vibrations and should be conducted in stable settings.
- Time-Consuming: The slower processing times may require considerations in experimental design to manage throughput.
In summary, understanding the three primary imaging modes in AFM—Contact, Tapping, and Non-Contact modes—enables researchers to select the best approach tailored to their specific samples and research goals. Each mode offers distinct benefits and challenges, making it essential to consider their operational mechanics and implications carefully.
Advantages of AFM Compared to Other Methods
Atomic Force Microscopy (AFM) stands as a pivotal technique within the realm of nanoscale imaging. Its advantages over alternative methodologies offer critical insights, making it a preferred choice in numerous scientific fields. Significant benefits include exceptional resolution, surface property analysis, and the ability to gather both topographical and mechanical information simultaneously. The relevance of these advantages is underscored by the continuous evolution of AFM methods, which align with the growing demands of modern research.
High Resolution Imaging
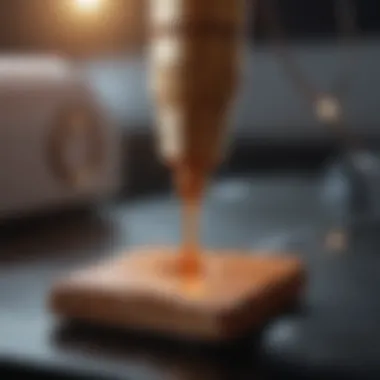
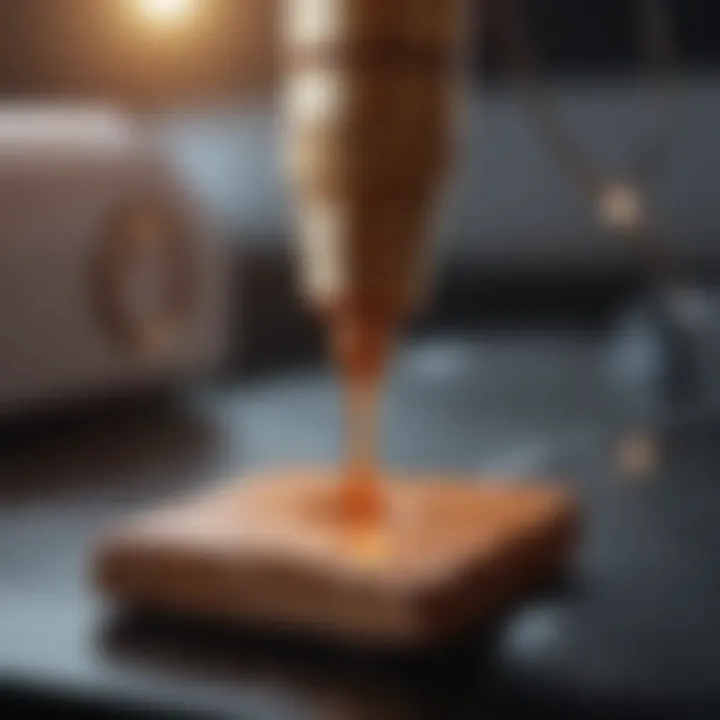
One of the most notable advantages of AFM is its high-resolution imaging capability. AFM can achieve resolutions in the nanometer range. This precision allows researchers to visualize structures that other microscopy methods may not resolve. For instance, techniques such as scanning electron microscopy (SEM) may provide surface images but lack the ability to measure forces at such minute scales. The ability of AFM to probe samples at atomic resolutions makes it a key tool in fields such as materials science and nanotechnology.
Surface Properties Analysis
Surface properties play a crucial role in many applications, from semiconductor manufacturing to biological research. AFM excels in this area through two primary means: topography analysis and mechanical property mapping. The unique capabilities of AFM in analyzing surfaces provide valuable data that informs the development and production of various materials.
Topography Analysis
Topography analysis is one of the central benefits of AFM. This method offers a detailed view of the sample's surface, capturing features such as height variations and surface roughness. The high-resolution imaging inherent to AFM allows researchers to discern even minor surface changes, which is vital in fields that rely on precise material characteristics. The unique feature of topography analysis lies in its non-destructive nature, allowing the investigation of samples without altering their original conditions. While advantageous, it does require careful calibration to ensure accurate results, especially in complex biological samples.
Mechanical Property Mapping
Mechanical property mapping is another significant aspect of AFM that contributes to understanding material behaviors. This method enables the assessment of mechanical properties such as stiffness, elasticity, and adhesion on a localized scale. The ability to correlate structural and mechanical data enhances the depth of analysis possible with AFM. A key characteristic of this approach is its capacity to detect variations across a sample, giving insights into heterogeneities that might be missed by conventional techniques. However, caution must be exercised when interpreting data, as environmental factors can influence mechanical responses.
The advantages of Atomic Force Microscopy are essential not only for academic research but also for practical applications across various industries, reaffirming its place in the toolkit of modern science.
Applications of AFM
Atomic Force Microscopy (AFM) has a wide range of applications across various fields, making it an essential tool in modern scientific research. Its versatility allows researchers to visualize and manipulate materials at the nanoscale, leading to significant advancements in multiple domains. This section will emphasize the importance of AFM applications in materials science, biological research, and nanotechnology.
AFM is characterized by its ability to provide high-resolution imaging and analysis, making it invaluable for studying surfaces and interfaces. The technique enables researchers to gather detailed topographical and mechanical information about a sample, which is crucial across disciplines from materials engineering to biology.
Materials Science
In materials science, AFM is critical for characterizing different types of materials, from polymers to metals. Researchers utilize AFM to analyze surface roughness, grain size, and phase distribution, among other properties. The high spatial resolution of AFM allows for the detection of structural irregularities that can affect material performance.
Through AFM imaging, scientists can investigate the mechanical properties of materials, such as Young's modulus and adhesion forces. These insights are essential for developing new materials that meet specific performance criteria. AFM also plays a role in the development of nanocomposites and smart materials, enabling researchers to optimize composition and structure at the nanoscale.
Biological Applications
AFM significantly contributes to biological applications by providing a platform that does not require extensive sample preparation, thus preserving the natural state of biological materials.
Cell Surface Studies
Cell surface studies via AFM focus on the characterization of cell membranes, allowing the investigation of the topography and mechanical properties at the cell level. This aspect is beneficial in understanding cell behavior and interaction with their environment. The unique feature of AFM is that it provides both qualitative and quantitative data at the nanoscale, making it a popular choice for biological research.
However, one challenge in cell surface studies is the potential for sample damage due to contact forces with the AFM tip. Despite this, the advantages of gaining precise insights into cellular structures make it a valuable tool in the study of cell biology.
Protein Interactions
Protein interactions are another vital area where AFM has a significant impact. AFM helps in mapping protein interactions by providing detailed information about binding kinetics and molecular forces. This application is crucial for understanding biochemical processes and interactions within cells.
The highlight of studying protein interactions with AFM is the ability to directly observe and measure these interactions at the molecular level. It allows researchers to analyze how proteins behave in real-time under various conditions. A consideration is the effect of environmental factors on protein stability, which may influence the accuracy of measurements. In summary, AFM's capability to explore protein interactions provides essential insights into cellular mechanisms.
Nanotechnology
In nanotechnology, AFM is revolutionary for its role in the fabrication and characterization of nanomaterials. It is used to manipulate nanoparticles and analyze nanostructures with precision. AFM enables the realization of nanoscale modifications to surfaces, which is essential for developing devices at the nano-scale level.
Overall, the applications of AFM span across various scientific fields, providing unique insights and facilitating advancements through detailed analysis and manipulation of materials at the nanoscale.
Challenges in AFM Imaging
Atomic Force Microscopy (AFM) provides a wealth of detailed information for researchers, yet it is not without challenges. Understanding these challenges is important to improve imaging results and ensure reliable data. Common issues arise in sample preparation, environmental factors, and the calibration process. By addressing these limitations, scientists can unlock the full potential of AFM imaging.
Sample Preparation
Proper sample preparation is essential for successful AFM imaging. The state of a sample can significantly influence the outcomes of measurements. Samples may require careful cleaving, coating, or even drying to minimize interference in the imaging process. Each material presents its own unique challenges. For instance, soft biological samples may need gentle treatment to preserve their structure. On the other hand, hard materials might need specific techniques to ensure a proper surface to scan.
Researchers often encounter difficulties in achieving the optimal thickness and morphology before imaging. Unprepared samples can lead to increased background noise, leading to inaccurate results. Therefore, understanding the requirements for each sample type is crucial for effective imaging.
Environmental Effects
Environmental factors can significantly affect AFM imaging results. Fluctuations in temperature, noise from machinery, and other vibrations can disrupt the stability of the AFM system.
Vibration Sensitivity
Vibration sensitivity refers to how susceptible the AFM setup is to external vibrations. High sensitivity to vibrations can cause distortions in images and alter the force measurements conducted by the AFM tip. This is a critical issue since AFM aims to achieve extremely high resolution, often down to nanometer scale. Researchers must minimize these vibrations through the installation of vibration isolation systems or placing the setup on sturdy surfaces.
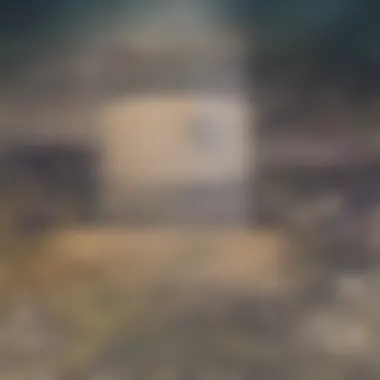
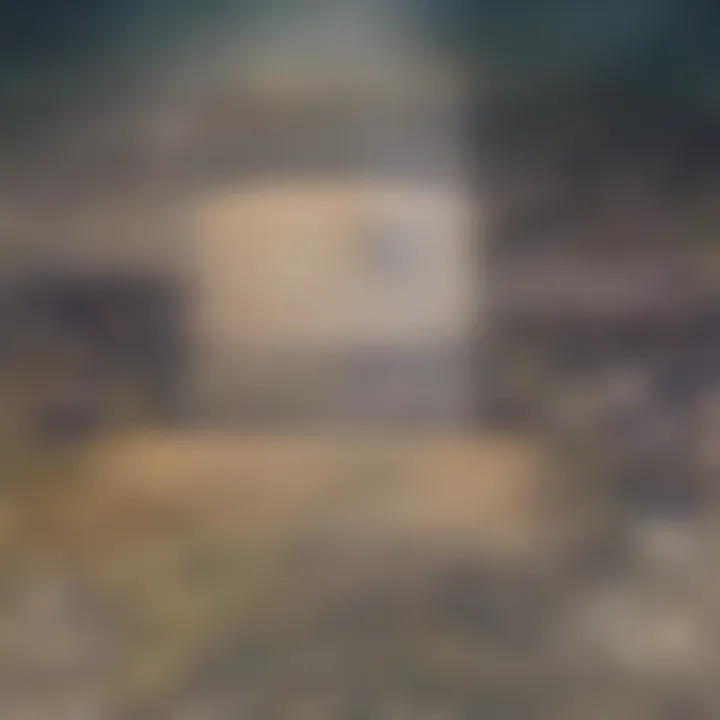
Key key characteristic of vibration sensitivity is its variable nature. Different AFM machines may handle vibrations differently based on their design. As a result, choosing the right setup can enhance data quality significantly. However, vibration sensitivity can also be an advantage when operated correctly, allowing the AFM to explore a wider range of sample types.
Humidity Effects
Humidity affects many aspects of AFM imaging, particularly in biological applications. Changes in moisture levels can alter the surface properties of materials. Hydrophilic materials tend to absorb moisture, affecting tip-sample interactions. This can result in reduced imaging accuracy. In contrast, too low humidity can lead to increased static charge, complicating the imaging process.
The key characteristic of humidity effects is its dual impact. While too much humidity can introduce problems, some moisture is necessary for the stability of soft biological samples. Balancing humidity levels is vital for conducting AFM studies without compromising results.
Calibration and Standardization
Calibration and standardization are paramount in AFM imaging. Without proper calibration, results can be inconsistent and unreliable. The precision of measurements depends on the accuracy of the AFM tip's positioning and the forces involved during scanning. Regular calibration to reference standards ensures that the data obtained remains trustworthy.
In addition, standardization aids in comparing results across different labs and studies. Establishing a common approach allows for meaningful comparisons in research, enhancing collaboration within scientific communities.
Overall, addressing these challenges enhances the feasibility of AFM imaging in various applications. Understanding and mitigating these issues leads to the production of high-quality data, facilitating advancements in nanotechnology and biological research.
Case Studies
Case studies play a vital role in understanding the principles and applications of Atomic Force Microscopy (AFM). They provide practical examples that illustrate theoretical concepts, making it easier for readers to grasp how AFM contributes to various fields. This section emphasizes specific instances where AFM has proven significant, highlighting its innovative applications and the benefits that arise from real-world usage.
Innovative Use in Nanofabrication
In the realm of nanofabrication, AFM has emerged as a powerful tool. Its precise imaging capabilities allow researchers to manipulate materials at the nanoscale, leading to advancements in semiconductor devices and nanoscale electronics. The ability to visualize surface structures and features enables more accurate fabricating processes.
Several studies have shown how AFM can be employed for the fabrication of nanostructures. For instance, researchers developed custom AFM tips to etch nanoscale patterns on surfaces, enabling the creation of unique materials. This innovative approach reduces costs and time compared to traditional methods.
In addition to its use in etching, AFM is instrumental in characterizing surface roughness and morphology, which are critical parameters in nanofabrication. By analyzing data gathered from AFM, scientists can refine techniques and improve the reliability of nanoscale production.
AFM in Biological Research
In biological research, AFM has opened new avenues for understanding cellular structures and interactions. The high-resolution imaging capabilities of AFM allow for the visualization of live cells without the need for labeling, significantly reducing any invasive impact on the specimen.
An example can be seen in the study of protein interactions. AFM provides insights into the mechanical properties of proteins, complex formations, and their behaviors in various environments. Researchers can examine cellular processes at the surface level, establishing a link between structure and function.
Moreover, AFM has been applied to study disease-related changes in cell surfaces. For instance, cancer cells can be analyzed for their topographical features, enabling better understanding of how these cells interact with their environment. This knowledge can assist with targeted therapies and drug design.
"AFM allows biologists to explore nanoscale interactions that were previously beyond reach, enabling profound insights into life at the molecular level."
The incorporation of case studies emphasizes the transformative role of AFM in both nanofabrication and biological applications. They illustrate the practical benefits of AFM technology and underscore its potential to advance scientific research across different disciplines.
Future of Atomic Force Microscopy
The future of Atomic Force Microscopy (AFM) holds significant potential as the demands of science and technology continue to evolve. This section addresses the relevance of AFM within the context of emerging technological advancements and interdisciplinary collaborations. AFM's capacity to analyze materials at the nanoscale makes it a crucial tool in both academic and industrial research. As we explore the future directions of AFM, it becomes evident that ongoing innovations will shape its applications and enhance its capabilities.
Emerging Technologies
Emerging technologies are poised to transform the landscape of microscopy. One notable trend is the integration of AFM with other imaging techniques. Hybrid systems that combine AFM with techniques like Scanning Electron Microscopy (SEM) or X-ray Photoelectron Spectroscopy (XPS) can provide complementary data. This integration enhances imaging resolution and surface characterization, enabling researchers to derive deeper insights from their samples.
Additionally, advances in nanomaterials are impacting AFM. Researchers are continually developing new types of cantilevers and probe tips tailored for specific applications. For instance, functionalized tips can increase specificity in biological applications, allowing for targeted investigations of cellular structures. Moreover, high-speed AFM is emerging, enabling real-time imaging of dynamic processes at the nanoscale. This capability can revolutionize our understanding of biological processes, such as protein folding and cell dynamics.
Overall, as these technologies evolve, they will empower researchers to tackle complex challenges across various fields, enhancing AFM’s role in scientific exploration.
Interdisciplinary Approaches
Interdisciplinary approaches are crucial for the advancement of Atomic Force Microscopy. The convergence of disciplines such as materials science, biology, and physics enriches the research landscape. For example, collaboration between physicists and biologists can lead to innovative applications in studying biomolecules. AFM offers unique insights that help bridge gaps between these disciplines, fostering a collaborative environment that encourages new methodologies.
Furthermore, the fusion of AFM with computational modeling is significant. Advanced algorithms and machine learning techniques can analyze AFM data more efficiently. This synergy of experimental data with computational tools leads to improved accuracy in interpreting material properties, facilitating advances in nanotechnology and materials design.
"The combination of experimental and computational approaches is fundamental for addressing the complexities of nanoscale research."
Finale
In the realm of scientific research, understanding Atomic Force Microscopy (AFM) is crucial. This conclusion synthesizes the various components of AFM and emphasizes its impact across multiple fields. The principles of AFM not only enable high-resolution imaging but also facilitate detailed analysis of material properties.
Summary of Key Insights
Through our exploration, we delved into the fundamental mechanisms at play in AFM. The combination of cantilever dynamics and interaction forces such as Van der Waals, electrostatic, and capillary forces provides a unique imaging technique unmatched by traditional methods. AFM's versatility, especially in analyzing surfaces at the nanoscale, allows for applications spanning materials science to biological research. Its ability to reveal fine structural details and surface characteristics emphasizes its significance.
Implications for Future Research
With the continuous evolution of AFM technology, the future holds immense potential. Researchers are likely to see advancements that enhance imaging speed and resolution. Furthermore, interdisciplinary approaches may lead to novel applications in biotechnology and nanomaterials. Future developments might focus on integrating AFM with other imaging modalities, enhancing its functionality. As methodologies evolve, understanding these implications is essential for advancing scientific knowledge and innovation.
"AFM serves as a bridge between understanding nanoscale properties and their application in real-world scenarios."
This conclusion encapsulates the transformative role of AFM while highlighting the importance of ongoing research to unlock its full potential in various disciplines.