Mole Analysis: The Key to Chemistry Measurement
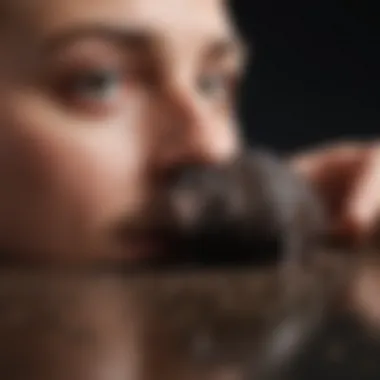
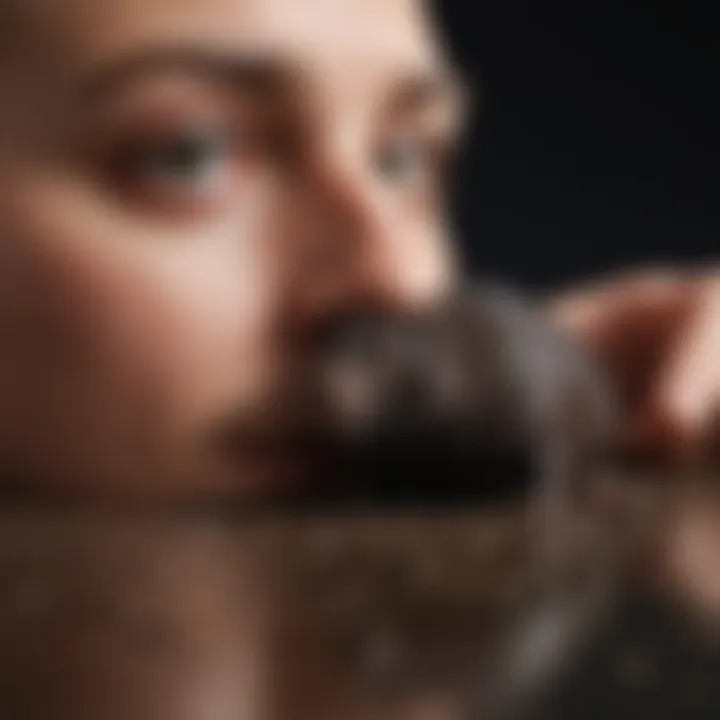
Intro
The concept of the mole is not merely a unit of measurement; it serves as a fundamental bridge connecting the macroscopic world we perceive with the molecular and atomic realms that govern chemical behavior. Understanding mole analysis is essential for anyone engaged in the field of chemistry, whether they are students, researchers, or professionals. By appreciating the historical context, mathematical underpinnings, and practical applications of the mole, one can grasp the significance of this unit in various scientific disciplines.
Mole analysis enables chemists to quantify substances precisely, facilitating accurate chemical reactions, formulations, and analyses. The history of the mole traces back to the late 19th century, where scientific advancements began to demand a standardized means of measurement. The establishment of the mole as a unit has transformed methodologies in experimental chemistry, providing clarity and coherence in measurements of matter.
This article systematically delves into the essential aspects of mole analysis. It starts with key findings that elucidate its importance and significance in research and industry. Following that, the implications of mole analysis will show how these findings apply in real-world contexts, ultimately painting a comprehensive picture of its relevance in scientific inquiry and practice.
Prologue to Mole Analysis
Mole analysis serves as a foundation in the study of chemistry. Understanding this concept aids in grasping how chemical reactions occur and how substances interact at a molecular level. The mole is more than just a number; it represents a crucial link between the macroscopic world we observe and the microscopic world of atoms and molecules. It allows chemists to quantify and communicate the amount of substance in a standardized way.
Definition of the Mole
The mole, often denoted as "mol," is defined as the amount of substance that contains as many entities as there are atoms in 12 grams of carbon-12. This value corresponds to Avogadro's number, which is approximately 6.022 x 10²³ entities per mole. Whether discussing atoms, molecules, or ions, the mole provides a consistent method for measuring and comparing quantities in chemical equations. It simplifies the process of converting mass to the number of particles, facilitating calculations that are pivotal in both academic and practical settings.
Historical Context
The concept of the mole has evolved significantly over time. Its origins can be traced back to the early studies of chemistry in the 19th century. Amedeo Avogadro, an Italian scientist, introduced the idea that equal volumes of gases, at the same temperature and pressure, contain an equal number of molecules. This was crucial for understanding gas behavior and led to the eventual establishment of the mole as a unit of measurement. Over the years, advancements in scientific knowledge and technology further refined and solidified the mole's definition. The mole's acceptance as an SI unit marked a pivotal moment in chemistry, allowing for greater precision in the field.
Importance in Chemistry
The importance of the mole in chemistry cannot be overstated. It is fundamental for several reasons:
- Quantitative Analysis: The mole allows for precise calculations of reactants and products in chemical reactions. This is vital in both laboratory and industrial settings.
- Stoichiometry: Understanding the mole is key to mastering stoichiometry, the study of the relationships between quantities of reactants and products in chemical reactions.
- Standardization: The mole provides a standardized unit that facilitates communication and collaboration among scientists worldwide.
The Concept of the Mole
Understanding the concept of the mole is crucial in chemistry, as it serves as the bridge between the microscopic world of atoms and molecules and the macroscopic world we observe. The mole is a unit that quantifies the amount of substance, facilitating the calculations necessary for chemical reactions and formulations. By using the mole, chemists can systematically describe amounts of substances, making it easier to relate to their physical properties or behaviors in reactions.
Avogadro's Number
Definition and Significance
Avogadro's number, approximately 6.022 x 10^23, defines the number of particles—be it atoms, molecules, or ions—in one mole of a substance. This number is pivotal because it allows chemists to convert between the mass of a substance and the number of entities it contains. The significance of Avogadro's number lies in its universality; it applies to all substances, enabling consistent and comparable measurements across different chemical contexts. A key characteristic of this number is its role in stoichiometry, allowing for accurate calculations in chemical reactions. The principle of using Avogadro's number simplifies understanding the proportions in which reactants combine.
"Avogadro's number is essential for converting between quantity and mass in chemical processes."
Applications in Mole Analysis
Avogadro's number has diverse applications in mole analysis. It simplifies the understanding of gas laws, where volume can be related to the number of moles of gas under standard conditions. Another application is in determining molecular weights, where it directly relates to the number of moles present in different substances. This approach vastly reduces complexities in interpreting experimental results. However, reliance on Avogadro's number can lead to oversimplifications, especially in non-ideal conditions, such as when dealing with high pressures or low temperatures.
Molar Mass and its Calculation
Defining Molar Mass
Molar mass refers to the mass of one mole of a substance, usually expressed in grams per mole (g/mol). It is a fundamental characteristic that serves to relate the mass of a substance to its amount in moles. Defining molar mass is important because it allows chemists to convert mass into the number of moles, facilitating stoichiometric calculations. A benefit of understanding molar mass is that it enhances clarity in quantitative analysis. Knowing the molar mass of a compound is a powerful tool in determining how much of each reactant is necessary for a reaction.
Calculating Molar Mass of Compounds
Calculating the molar mass of compounds involves summing the molar masses of the constituent elements, multiplied by their respective quantities in the molecular formula. This calculation is critical in practical applications, such as preparing reactions in labs or determining concentrations in solutions. The ease of calculating molar mass provides efficiency in both educational settings and professional laboratories. However, potential issues could arise if there is a lack of precision in measuring the element's molar mass or misinterpretation of the molecular formula.
The concept of the mole, bolstered by Avogadro's number and molar mass, is not just a theoretical idea but a practical necessity in the field of chemistry. Through understanding these elements, chemists gain the tools necessary for effective experimentation and data interpretation.
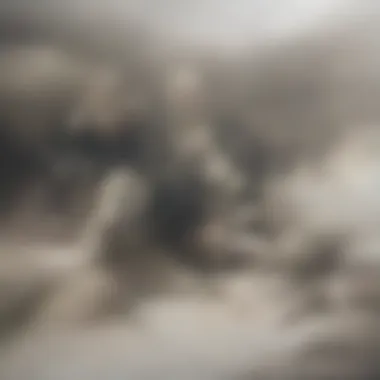
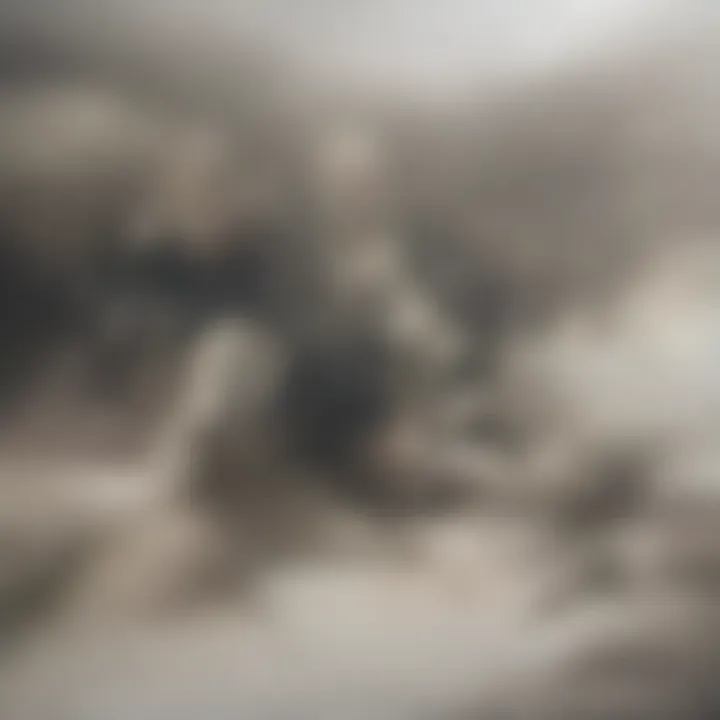
Methods of Mole Analysis
Understanding the methods of mole analysis is fundamental in chemistry. It provides scientists and researchers with effective tools to measure and analyze substances accurately. The methods discussed here are gravimetric analysis and volumetric analysis. Each method has its own advantages and applications, serving various analytical needs. Knowing which method to use is essential for achieving reliable data in chemical experimentation and research.
Gravimetric Analysis
Gravimetric analysis is a technique used to determine the quantity of a substance by converting it into a stable form. It primarily relies on mass measurements, thus making mass a central component of this analytical method. The principle of gravimetric analysis is built on the accurate measurement of mass to quantify analyte concentration. This is critical because precise measurements yield trustworthy results, which are necessary in comparing different studies in chemical science.
Principles of Gravimetric Analysis
The key characteristic of principles of gravimetric analysis is that substances are transformed into solid precipitates. This transformation allows for easy separation from the solution, simplifying the analysis. The unique feature of this method is its reliance on the mass of these precipitates to reach conclusions about the analyte.
Advantages of this method include its overall reliability, and its ability to offer high precision and accuracy in many cases. However, the disadvantages may include a longer time requirement compared to other methods and the necessity for careful handling of samples to avoid contamination.
Steps Involved in the Procedure
The steps involved in gravimetric analysis are systematic and crucial for successful implementation. Initial preparation involves dissolving the sample in a suitable solvent, followed by the addition of a precipitating agent, which will cause the desired compound to form a solid. This solid is then filtered out, washed, and dried to a consistent mass.
A key characteristic of these steps is the attention to detail required during filtration and drying processes. This meticulousness guarantees sample purity and accuracy in mass measurement. The unique feature here is how each step interrelates to ensure that the final measurement reflects the true amount of the substance of interest.
The advantages of following these precise steps include the production of reliable and repeatable results, while the disadvantages could be the full complexity of the procedure which necessitates a solid understanding of chemical principles.
Volumetric Analysis
Volumetric analysis is another primary method used to analyze the composition of substances. It is often faster than gravimetric analysis, thus making it preferable for situations requiring rapid results. This method involves measuring the volume of a solution needed to react with the analyte to determine its concentration.
Titration Methods
Titration methods are a specific application of volumetric analysis. This technique provides the user with a visual endpoint, typically indicated by a color change when a reactive solution (titrant) interacts with the analyte. A key characteristic of titration is its ability to provide quick results with reasonable accuracy.
The unique feature of titration methods lies in their flexibility. They can be adapted for various reactions and can be used for different types of analysis, such as acid-base or redox titrations. Nevertheless, the disadvantages may include the requirement for a well-calibrated setup, and the potential for human error during the titration process.
Interpreting Titration Data
Interpreting titration data involves understanding the relationship between the titrant volume used and the concentration of the analyte. This aspect of volumetric analysis is crucial, as it allows chemists to derive quantitative conclusions regarding substance concentrations based on the volume of titrant consumed.
A significant characteristic of interpreting titration data is that it often involves calculations that are foundational for stoichiometric evaluations. The unique feature here is that the data analyzed can inform future experiments by providing insights into the efficiency and precision of reactions.
Advantages of interpreting this data include the ease of drawing conclusions and the ability to compare reactions systematically. However, potential disadvantages exist in terms of accuracy based on user technique and measurement precision.
Applications of Mole Analysis
Mole analysis serves as a keystone in chemistry and countless scientific disciplines. Its importance lies in the ability to quantify relationships between substances involved in chemical reactions. By evaluating the moles of reactants and products, scientists can predict outcomes, optimize conditions, and ensure safety in various processes. This section delves deeper into two primary applications of mole analysis: quantifying chemical reactions and environmental chemistry.
Quantifying Chemical Reactions
Chemical reactions form the bedrock of chemistry, as they explain how substances interact. Mole analysis aids in quantifying these reactions, which is vital for predicting yields and understanding reaction dynamics.
Balancing Chemical Equations
Balancing chemical equations ensures the law of conservation of mass holds true. Each element's concentration must remain constant before and after the reaction, making it a core principle in chemical analysis. Its significance lies in providing a clear and structured representation of the transformation that occurs during a reaction.
The key characteristic of balancing chemical equations is its clarity. It simplifies the communication of complex chemical processes. Professionals find it beneficial as it gives immediate insight into reactant-product relationships. Nonetheless, balancing can be challenging, particularly for intricate reactions involving multiple steps or components.
Stoichiometry in Reactions
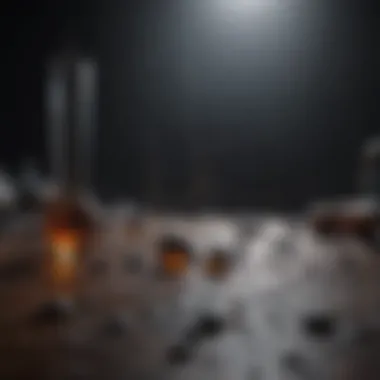
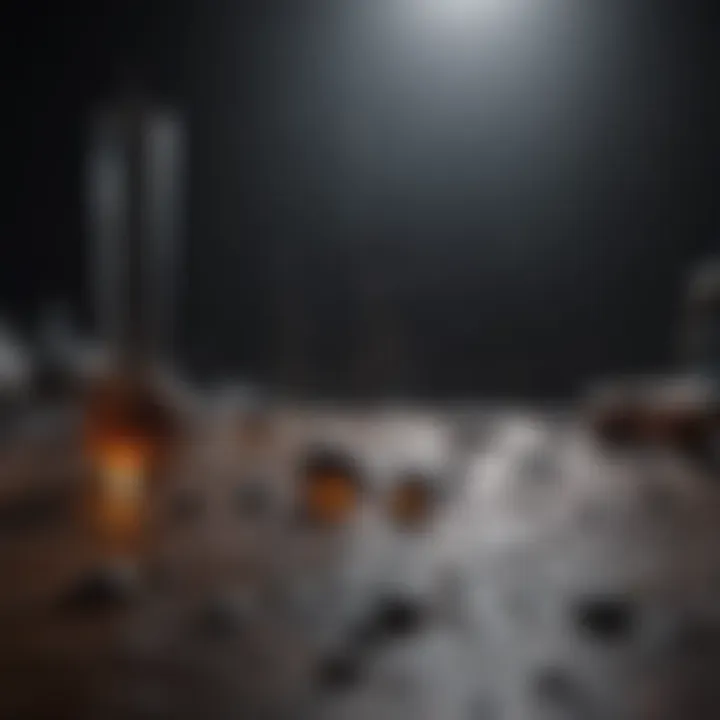
Stoichiometry allows chemists to quantify reactants and products. It helps determine the exact amounts needed to produce a desired quantity of product. The calculations performed in stoichiometry are based on the coefficients derived from balanced chemical equations, thus linking theory to practical applications.
Its main strength is in its accuracy. Accurate stoichiometric calculations enable researchers to minimize waste and improve efficiency in reactions. However, limitations exist, especially when dealing with side reactions or incomplete reactions, which can complicate predictions and outcomes.
Environmental Chemistry
The application of mole analysis in environmental chemistry is another vital area of study. It aids in understanding pollutants and their effects on ecosystems. Accurate measurements can influence policies and intervention strategies aimed at combating pollution.
Analyzing Pollutants
Analyzing pollutants involves measuring toxic substances in various environments. This assessment is crucial for monitoring environmental health and implementing regulatory measures. It enables scientists to determine the concentration of pollutants and their potential impacts on human and ecological health.
A key feature of this analysis is its comprehensive scope. By analyzing the moles of various pollutants, researchers can derive insightful conclusions about contamination levels. However, challenges exist such as distinguishing between various sources of pollutants and understanding their transformations in the environment.
Assessing Chemical Concentrations
Assessing chemical concentrations allows for determining how much of a substance is present in a given volume. This analysis is significant for setting safety standards and understanding exposure risks. Monitoring the concentration of chemicals in air, water, and soil is crucial in environmental science.
The main advantage is that it provides a solid foundation for understanding environmental chemistry. It enables the assessment of compliance with environmental regulations. Conversely, this process may be complicated by variations in sample collection and external factors that affect the concentration observed.
"A precise understanding of the role of moles in environmental chemistry is essential to effective risk assessment and pollution management."
Mole analysis thus serves as an indispensable tool in both quantifying chemical reactions and environmental assessments, reinforcing its role as crucial in advancing scientific inquiry and application.
Challenges in Mole Analysis
Mole analysis is a fundamental aspect of chemistry, yet it is not without its challenges. These challenges can significantly impact the accuracy and reliability of results in chemical analysis and experimentation. Recognizing these challenges is crucial for chemists, educators, and students alike. Understanding the common pitfalls and limitations helps to ensure that mole analysis is conducted with a high degree of precision.
Error Analysis
Sources of Error
Sources of error in mole analysis can arise from various factors, including sample purity, measurement techniques, and environmental conditions. A key characteristic of these sources is their potential to compromise the integrity of the analytical results. For instance, impurities within a sample can lead to misleading calculations of molar mass or concentration. This aspect is particularly relevant to this article, as it emphasizes the need for meticulous sample preparation and handling.
Errors can be broadly categorized into systematic errors and random errors. Systematic errors tend to be consistent, while random errors fluctuate. The unique feature of understanding sources of error lies in its ability to guide chemists towards improving their methodologies, thereby enhancing reliability. However, addressing these errors often requires significant time and resources, which may be a disadvantage in fast-paced research environments.
Minimizing Measurement Errors
Minimizing measurement errors is an essential strategy in mole analysis. This involves using precise instruments and standardized procedures. A key characteristic of this approach is the implementation of best practices, such as calibrating equipment before use and applying statistical methods to assess data reliability. This is beneficial for this article because it underscores the importance of establishing robust protocols that can combat prevalent sources of error.
The unique feature of this minimization process is its emphasis on continuous improvement. By thoroughly analyzing previous results and identifying discrepancies, researchers can refine their techniques over time. The significant advantage is that it leads to more reliable data that can inform future experiments. However, the downside could be the increased time commitment required to ensure consistent accuracy, which some researchers may find burdensome.
Limitations of Traditional Methods
Precision and Accuracy
Precision and accuracy are crucial considerations in mole analysis. The precision refers to how reproducible results are, while accuracy indicates how close results are to the true value. The interplay between these two factors is vital for reliable mole analysis. A key characteristic is that high precision with low accuracy can lead to significant errors in interpreting mole data, making this a critical point in the article.
Understanding both precision and accuracy in traditional methods allows chemists to identify potential discrepancies in their findings. The unique feature here is the ability to quantify these metrics through statistical analysis, providing a clearer picture of data reliability. However, traditional methods may sometimes fall short in delivering both high precision and high accuracy, particularly in complex mixtures or reactions.
Emerging Analytical Techniques
Emerging analytical techniques play a significant role in advancing mole analysis. Techniques such as mass spectrometry and high-performance liquid chromatography offer enhanced sensitivity and accuracy. One key characteristic of these emerging methods is their ability to handle complex analyses that traditional methods often struggle with. This advance is beneficial to this article as it showcases the ongoing evolution in analytical chemistry.
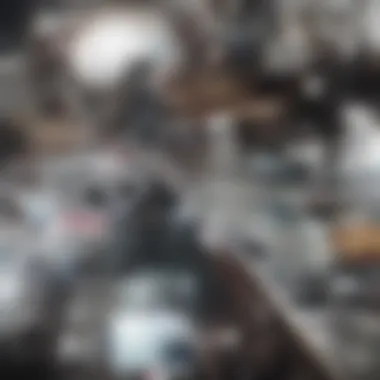
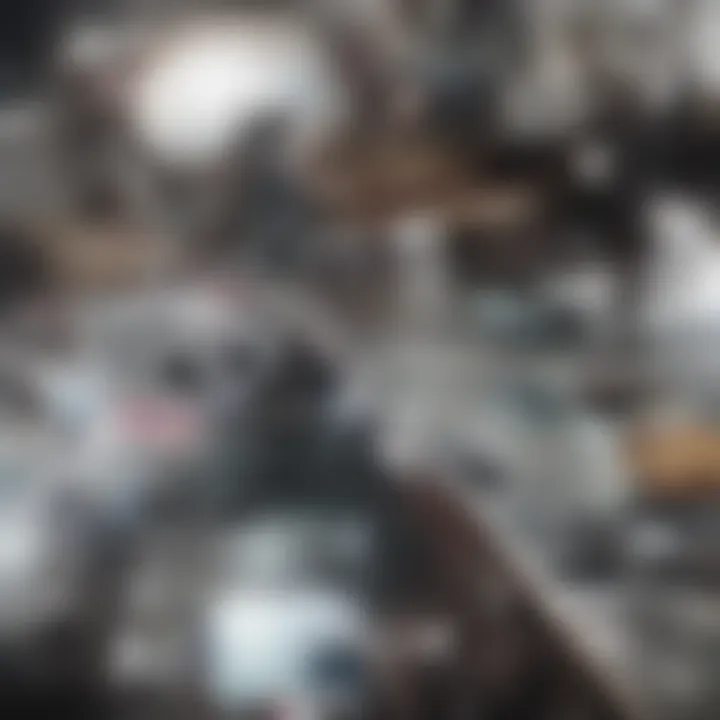
The unique feature of these techniques is their adaptability to various sample types, which allows researchers to explore new areas of study and gather more comprehensive data. While these modern techniques can provide significant advantages, they may also present disadvantages, such as the high cost of equipment and the need for specialized training. Despite these challenges, the integration of advanced methods remains a crucial direction in the field of mole analysis.
Future Directions in Mole Analysis Research
Research in mole analysis continues to evolve, driven by advances in technology and education methods. The future of mole analysis is integral to its role in enhancing scientific accuracy and expanding its applications across various fields. Understanding future directions is essential not only for academics but also for professionals who rely on precise measurements in their work. The integration of innovative technologies and educational practices presents unique opportunities for growth in this area.
Integration with Technology
Use of Software in Mole Calculations
The utilization of software in mole calculations has reshaped how chemists approach molecular quantification. These tools provide users with quick access to complex calculations that would traditionally require significant effort and time. Key characteristics of this software include user-friendly interfaces and the ability to update in real-time as new data becomes available. Popular choices such as ChemDoodle or Mendeley provide such capabilities.
Additionally, the unique feature of automated calculations allows chemists to focus on analysis rather than arithmetic. However, reliance on these technologies can pose disadvantages, such as the risk of software errors or misinterpretation of results. Overall, software enhances the efficiency of mole calculations but should be used with a critical eye.
Advances in Measurement Techniques
Emerging measurement techniques, particularly those involving spectrometry and chromatography, are pushing the boundaries of mole analysis. These advances provide greater sensitivity and accuracy when detecting and quantifying substances. The key characteristic of these techniques is their ability to analyze samples at a molecular level, providing comprehensive data that was previously difficult to obtain.
One unique feature of these advanced techniques is their ability to handle complex mixtures, making them essential in environmental and pharmaceutical analysis. Their advantages are substantial, but they often require expensive instruments and advanced technical skills, which can be a barrier for many laboratories. Despite these challenges, the potential for innovation in measurement techniques promises to greatly enhance mole analysis in the future.
Educational Innovations
Improving Curriculum on Mole Concepts
Enhancements in the curriculum surrounding mole concepts are vital for fostering a deeper understanding among students. This improvement involves integrating practical applications alongside theoretical foundations, making the subject more relevant to real-world scenarios. By utilizing modern teaching tools, educators can create dynamic learning experiences that engage students.
The key characteristic of these educational innovations is their focus on hands-on experiments. This approach not only reinforces learned concepts but also builds essential skills in measurement and analysis. The drawbacks may include the need for increased resources and trained instructors, but the long-term benefits of an improved understanding of mole concepts are significant.
Public Understanding of Chemistry
Raising public awareness and understanding of chemistry is crucial for fostering a scientifically literate society. Initiatives that demystify chemical processes can lead to better decision-making regarding public health and environmental concerns. The key characteristic of this effort is its accessibility; educational programs, public demonstrations, and online resources aim to engage a broader audience.
One unique feature of these initiatives is their interdisciplinary approach, highlighting the connections between chemistry and everyday life. The advantages include greater public interest in science, but challenges remain, such as the potential for misinformation and the need for accurate representation. Nevertheless, improving public understanding of chemistry is vital for advancing mole analysis research and its applications in society.
"Education is the most powerful weapon which you can use to change the world." - Nelson Mandela
Epilogue
The conclusion of this article serves as a critical reflection on the concept of mole analysis. It encapsulates the significance of the mole as a fundamental unit in chemistry. Understanding mole analysis is essential for interpreting various chemical phenomena. In scientific disciplines, the mole provides a common framework, allowing for precise measurements and reliable calculations. This helps researchers adequately assess the quantities of reactants and products in chemical reactions.
Moreover, mole analysis integrates mathematical principles with practical applications. It is not just a theoretical construct but a vital tool in laboratory settings and industrial applications. By grasping these concepts, students and professionals can appreciate the depth of chemical interactions.
Summarizing Key Insights
Key insights from this article highlight the historical context, mathematical foundation, and methodologies of mole analysis. The mole has a rich history that traces back to early chemical discoveries. Its definition, particularly Avogadro's Number, remains pivotal in calculations involving quantities of substances.
The essence of mole analysis lies in its practical applications. Key takeaways include:
- The mole's role in stoichiometry, enabling accurate measurement of reactants and products.
- Various methods of mole analysis such as gravimetric and volumetric techniques, which provide reliable data.
- The importance of understanding error sources and limitations in mole analysis to enhance measurement precision.
Implications for Future Research
Looking forward, mole analysis continues to hold potential for further exploration. The integration of technology in educational frameworks can stimulate new methods of teaching these concepts. For example, innovative software can assist with complex mole calculations, making the subject more accessible.
Future research could also focus on refining analytical methods, addressing limitations in current practices. As the field of chemistry evolves, new approaches in mole analysis will likely emerge, enhancing our understanding of chemical measurements.
Recognizing the implications of mole analysis extends to environmental studies, pharmaceuticals, and beyond. The ongoing evolution of this fundamental concept will ensure its relevance in addressing contemporary scientific challenges.
"The future of mole analysis lies in technological integration and innovative educational practices."
Increasing public understanding of these concepts can foster a new generation of informed chemists and researchers.