Microfluidic Devices Transforming Biomedical Practices
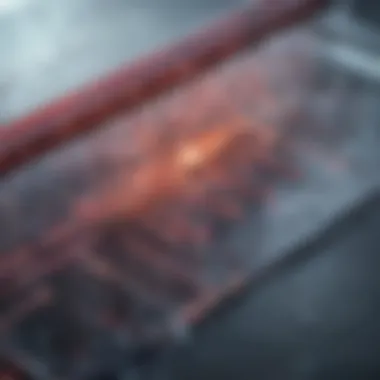
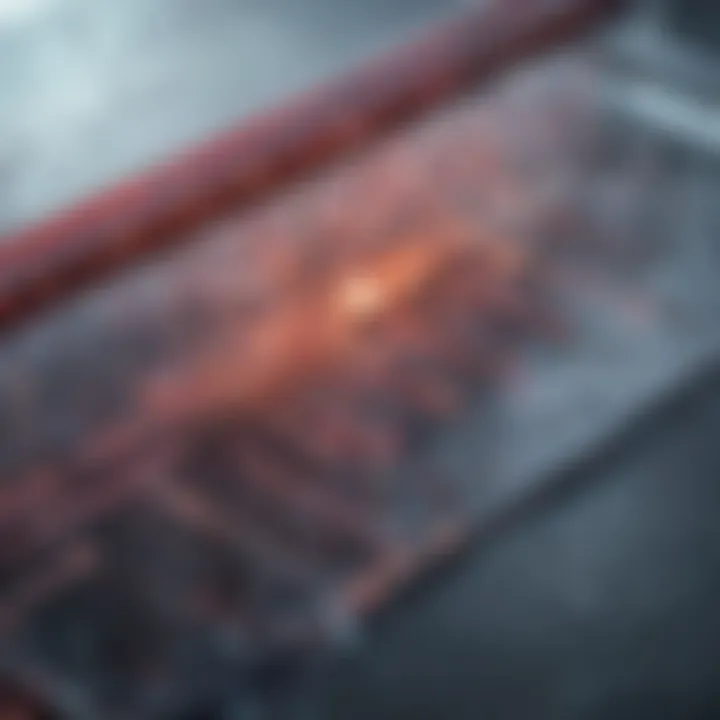
Intro
Microfluidic devices are increasingly becoming the backbone of innovative solutions in the biomedical field. They are not just a fad; they truly are a game-changer with their ability to manipulate tiny amounts of fluids with remarkable precision. From diagnostics to drug development, the implications are as vast as the ocean. As we navigate through the intricacies of these devices, it’s essential to grasp their design, functionality, and integration into various applications.
Designed at the micro-scale, these devices can handle volumes as minuscule as a few picoliters, allowing for unprecedented control and efficiency. Imagine you need to analyze a single drop of blood for various biomarkers; with microfluidics, this is not only possible but also affordable and quick. This capacity to perform multiple analyses concurrently on microsized samples opens new avenues in personalized medicine, bringing tailored healthcare solutions closer to reality.
"Microfluidics represents a paradigm shift in how we approach diagnostics and therapeutic applications, underscoring a future where precision is paramount."
Over the years, remarkable advancements have been made in microfluidics technology. Researchers have developed systems that not just engage in fluid manipulation but can do so in highly complex environments, such as mimicking human organs-on-a-chip. These developments have profound implications, both practically and theoretically, leading to new paradigms in medical research and patient care. Understanding these devices and their applications is critical for all stakeholders—students, professionals, and researchers alike.
As we delve deeper into this subject, we will explore key findings, implications of ongoing research, and how these technologies could shape the future of healthcare.
Preamble to Microfluidics
Microfluidics refers to the manipulation of fluids on a microscale, typically within channels that measure less than one millimeter in diameter. This fascinating area of study has immense implications, especially when applied to the biomedical field. The ability to precisely handle minute amounts of fluids paves the way for innovations in diagnostics, drug delivery, and personalized medicine. From the mechanics of fluid dynamics to the intricate designs of devices, understanding microfluidics is key for anyone involved in health science, research, and technology.
Definition and Fundamentals
In simpler terms, microfluidics can be thought of as the science of controlling and directing tiny quantities of liquids in very small spaces. At the core of this technology is the aim to replicate or enhance biological processes on a scale that is easier to manage and analyze. Microfluidic devices can take various forms, including chips that resemble a circuit board, but instead of managing electrical signals, they orchestrate the flow of liquids. These devices rely on principles from physics, chemistry, and engineering to function effectively.
One fundamental aspect lies in the concept of hydrodynamic flow, which dictates how liquids behave when introduced into narrow channels. The Reynolds number, a dimensionless quantity in fluid mechanics, helps determine whether the flow will be laminar or turbulent. In the case of microfluidics, laminar flow is dominant, allowing for distinct control over mixing, reactions, and movement of the fluids involved.
"Microfluidic technology reshapes paradigms in diagnostics and therapeutics, showcasing what can be achieved when we push the boundaries of conventional techniques."
Historical Context
The roots of microfluidics trace back to the 1990s, during which time scientists began to explore the potential of miniaturizing laboratory processes. Early work focused on developing simpler methods for isolating and analyzing cells or biomolecules. One notable figure is George Whitesides, whose work in applying principles of fluid mechanics set the stage for microfluidics to capture ample attention in scientific circles.
Fast forward, the 2000s saw rapid advancements, with researchers introducing various methods for fabricating microfluidic devices from materials like PDMS (polydimethylsiloxane). The flexibility and ease of use made PDMS a favored choice, allowing for a range of applications from diagnostics to biosensing. Today, we witness an explosion of innovations stemming from those initial breakthroughs, with countless laboratories globally leaning into the potential of microfluidics to push the boundaries of what we know about biological systems.
This journey from theoretical exploration to practical application encapsulates the spirit of microfluidics. With an eye toward not only improving existing technologies but also developing new therapeutic strategies, the importance of this field continues to grow, promising a future rich with potential breakthroughs.
The Mechanisms of Microfluidic Devices
Understanding the mechanisms behind microfluidic devices is crucial for grasping their significance in biomedical applications. These devices manipulate fluids at a microscale, enabling precise control over biological assays, diagnostics, and therapeutic solutions. The mechanisms that operate within microfluidics not only distinguish them from traditional fluid handling systems but also contribute to their growing adoption in research and clinical settings.
Fluid Dynamics in Microscales
When we talk about fluid dynamics, we usually think about water flowing in rivers or the air circulating in a room. But at the microscopic level, things change dramatically. In microfluidics, one of the key principles guiding fluid behavior is the concept of surface tension, which becomes disproportionately impactful when dimensions shrink. This phenomenon leads to different behaviors such as capillary action, where liquids can rise or fall in tiny channels without any external force.
Additionally, the term ‘laminar flow’ comes into play. At this scale, fluid flows in thin layers, with minimal mixing between them. This is significant because it allows for greater control and predictability in chemical reactions or cellular interactions.
When designing microfluidic devices, researchers must account for factors like fluid viscosity and flow rate. For example, while higher viscosity fluids may move slower, they can provide more accurate results in certain applications. Thus, understanding these microscopic dynamics is critical, as it influences everything—from the layout of the channels to the overall efficiency of the devices used in specific biomedical research.
"The mastery of fluid dynamics in microscale environments is like learning to dance within a grain of sand; every movement, every decision can trigger vast responses."
Control Mechanisms
Transitioning to the control mechanisms, we find that microfluidic devices rely heavily on various approaches to manage fluid movement effectively. Some key control mechanisms include:
- Pressure-driven Flow: This mechanism uses pressure differences to push fluids through channels, allowing for fast and efficient movement. It’s similar to squeezing a toothpaste tube—pressure at one end makes the paste flow seamlessly at the other.
- Electrokinetic Flow: Here, electric fields are applied to induce movement in charged particles within the fluid. This method is particularly useful for rapid mixing and separation processes, providing a level of control not achievable through pressure alone.
- Pneumatic Control: Utilizing gas pressure, pneumatic control allows for on-demand adjustments in microfluidic channels. This is notable in applications that require precision, such as during cell sorting or handling delicate biological samples.
It's also worth mentioning that integration with digital technologies has enabled automated control of these mechanisms. Software algorithms now play an essential role, allowing for fine-tuning in real-time, which is key for applications like point-of-care diagnostics where quick responses are vital.
The interplay between fluid dynamics and control mechanisms ultimately shapes the design and functionality of microfluidic devices, making them versatile tools in the biomedical landscape. Without a firm grasp of these mechanisms, progress in devices could be like building a house on sand—unstable and unreliable at best.
Materials in Microfluidic Device Fabrication
In the arena of microfluidics, the materials used in the fabrication of devices play a pivotal role, influencing their functionality, durability, and applicability. Knowing the right material can be the difference between a successful experiment and a costly failure. The choice of materials isn't merely a matter of preference; it significantly affects the outcome of the device's application in biomedical research and diagnostics. This section will explore common materials utilized in microfluidic device fabrication and the criteria for selecting them, providing a comprehensive overview essential for researchers and professionals in the field.
Common Materials
Microfluidic devices can be constructed from various materials, with some standing out due to their unique properties:
- Polydimethylsiloxane (PDMS): Widely favored for its optical transparency and flexibility, PDMS allows for easy manufacturing processes like soft lithography. It's especially useful in applications that require manipulation of small fluid volumes. However, it’s worth noting that PDMS can absorb small molecules, which might interfere with certain assays.
- Glass: Glass channels are known for their low background fluorescence and high chemical resistance, making them ideal for sensitive optical detection methods. They provide a sterile surface but are often more brittle, which poses challenges in terms of fabrication and handling.
- Thermoplastics (like PMMA and polycarbonate): These materials offer a good balance between durability and ease of manufacturing. They can be thermally bonded and are suitable for high-throughput applications. However, their optical clarity is often less than that of glass or PDMS.
- Silicon: Silicon wafers have been utilized for their excellent electronic properties, especially in devices intended for biosensing applications. Yet, the cost and complexity of working with silicon can be drawbacks for some applications.
Each material has its strengths and weaknesses, and understanding these can significantly steer the direction of microfluidic device design and application.
Material Selection Criteria
When it comes to selecting materials for microfluidic devices, several critical factors need thoughtful consideration:
- Chemical Compatibility: Materials must be compatible with the fluids being manipulated. For example, solvents or reagents that can degrade certain materials must be avoided to ensure the integrity of the device.
- Optical Properties: A transparent material is essential for applications relying on light-based detection methods. This ensures that the results are reliable and accurate.
- Mechanical Properties: Flexibility and tensile strength are important, especially for devices subjected to pressure or bending. In some cases, too much flexibility can lead to undesired effects like leakage.
- Fabrication Methods: The chosen materials should align with the available manufacturing techniques. Some materials are better suited for certain methods, such as photolithography, casting, or molding.
- Cost-effectiveness: In research settings, particularly in academia, budget constraints can significantly influence material choice. Balancing quality with cost is often necessary without compromising the experimental integrity.
"Choosing the right material is like laying a solid foundation for a house; without it, everything that follows is at risk."
Ultimately, selecting the right materials for microfluidic device fabrication requires a holistic view of both the intended application and the material properties. As technology advances, new materials may emerge, promising even more innovative applications in the biomedical field.
Applications in Biomedical Research
The integration of microfluidic devices into biomedical research is nothing short of transformative. These systems offer a level of precision and control over fluid dynamics that traditional methods simply can't match. This capability not only enhances the efficiency of experiments, but also enables researchers to explore complex biological interactions at a fundamentally new scale. With decreasing sample volumes and accelerated processing times, microfluidics holds immense significance for diagnostics, drug testing, and the development of personalized therapies.
Laboratory Automation
One of the standout features of microfluidic devices is their ability to facilitate laboratory automation. Automated systems have become crucial in modern research settings, where time and resources are often at a premium. By incorporating microfluidic technologies, repetitive tasks that previously required substantial manual input can now be performed with high throughput and minimal human intervention.
For example, microfluidic platforms can integrate various laboratory functions like sample preparation, reaction monitoring, and even data analysis into a single, compact device. This means that researchers can run multiple assays concurrently, significantly speeding up the workflow. In this context, microfluidic systems can be programmed to handle tasks sequentially and precisely.
- Reduced Human Error:
- Cost-Effectiveness:
- Automation minimizes the likelihood of errors that come with manual pipetting and sample handling, resulting in more reliable outcomes.
- Scaling down sample sizes translates to lower reagent costs, making it financially viable for various labs, especially in academic settings.
In short, laboratory automation through microfluidic devices not only saves time but also enhances accuracy, allowing researchers to focus more on analyzing results rather than conducting repetitive tasks.
Cell and Tissue Studies
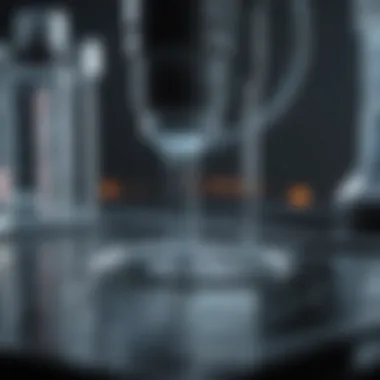
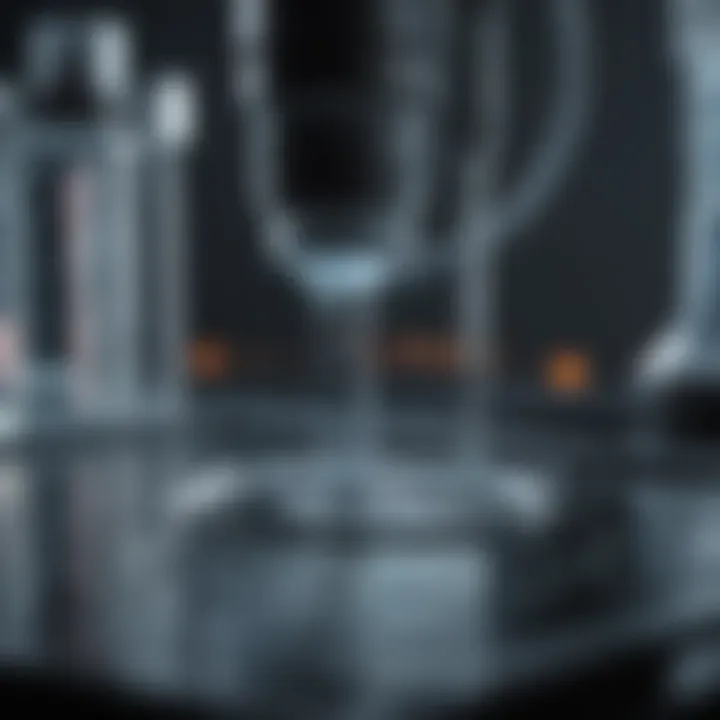
The application of microfluidic devices in cell and tissue studies pushes the envelope of what is possible in biomedical research. These devices excel in creating microenvironments that closely mimic in vivo conditions, which is essential for studying cellular behavior.
By providing precise control over factors like fluid shear stress, nutrient gradients, and cellular interactions within a contained system, researchers can better understand how cells operate in their natural state. Importantly, microfluidic devices can facilitate the growth of organoids and biomimetic tissues, thereby paving the way for more relevant preclinical testing and drug discovery processes.
To illustrate this point, consider the use of microfluidics in cancer research. Through the creation of tumor-on-a-chip models, scientists are able to examine how cancer cells interact with different therapies under controlled conditions.
- Customization:
- High-Throughput Analysis:
- Researchers can tailor the microfluidic chips to study specific cell types or responses, enhancing the relevance of their findings.
- Microfluidics allows for simultaneous analysis of various conditions, providing rich data sets to identify effective treatments quickly.
"The precision of microfluidic systems enables a level of biological interrogation that was previously unattainable, offering new insights into cellular mechanisms and disease pathology."
In summary, the use of microfluidic devices in laboratory automation and cell and tissue studies signifies a leap forward in biomedical applications. As these technologies continue to evolve, the potential to advance our understanding of complex biological systems will undoubtedly expand, highlighting the unrelenting pace of innovation in this field.
Diagnostic Applications
In the realm of biomedical applications, diagnostic capabilities hold paramount importance. Microfluidic devices have ushered in a new era of efficiency and precision in diagnostics, streamlining processes that were, until now, cumbersome, manual, and often prone to error. The demand for rapid, accurate diagnostic tools has never been more critical, particularly in the context of infectious diseases, chronic health conditions, and even routine health maintenance.
Microfluidics enables the manipulation of tiny volumes of fluids, often just microliters, enhancing the sensitivity of diagnostics while reducing waste. The overarching benefits can be categorized as follows:
- Speed: Quick results can facilitate immediate treatment decisions.
- Cost-effectiveness: Reduced reagent use lowers overall testing costs.
- Miniaturization: Compact devices can be easily integrated into various settings, from sophisticated labs to remote locations.
- Versatility: The ability to conduct a broad range of tests from a single sample streamlines workflows.
With these attributes, microfluidic diagnostic applications significantly contribute to patient care by delivering vital information more efficiently than traditional methods.
Drug Development and Delivery
The landscape of drug development and delivery is undergoing an extraordinary transformation, largely thanks to microfluidic devices. These tools enable researchers and pharmaceutical companies to test and develop new drugs more efficiently and with greater precision. The miniaturization of laboratory processes not only accelerates research pace but also reduces costs and resource expenditure. With the increasing urgency in drug development—particularly in adapting to emerging health crises—microfluidics plays a crucial role.
One of the notable benefits of utilizing microfluidics in drug development is the enhancement of screening processes. By integrating advanced fluid manipulation at a microscopic level, researchers can optimize and automate high-throughput screening methods. This provides a broader analysis of candidate compounds with minimal sample volumes. For instance, in a typical high-throughput screen, hundreds or thousands of drug candidates can be tested simultaneously in smaller wells or channels compared to traditional methods. This efficiency not only saves time but also resource allocation, leading to a promising yield of actionable data.
High-Throughput Screening
High-throughput screening (HTS) is vital in the early phases of drug discovery. Microfluidic devices facilitate the parallel testing of various drug candidates under controlled conditions. Researchers can manipulate fluid flows — adjusting volumes, mixing reagents, or even controlling environmental factors directly on the device. This precise control allows for rapid evaluation of potential drugs and their interactions with biological systems.
Some key aspects of high-throughput screening using microfluidics include:
- Decreased Sample Volume: Microfluidics requires significantly less sample volume, making it cost-effective.
- Fast Analysis: The speed at which experiments can be conducted allows for quicker decision-making in drug candidate selection.
- Data Generation: More data means better predictive models for drug efficacy and safety, providing insight that would take longer through manual tasks other methods.
Importantly, HTS powered by microfluidics is not without its challenges. The initial setup, including the development of the appropriate microfluidic systems, can be resource-intensive. However, as automation and device design improve, these hurdles are gradually being overcome, bringing us closer to a new era of rapid drug testing and personalized medicine.
Controlled Release Systems
Controlled release systems are another fascinating aspect of drug delivery enhanced by microfluidic technology. These systems are designed to release therapeutic agents at a predetermined rate, optimizing the therapeutic window of drugs and minimizing side effects.
Microfluidic devices enable the design of sophisticated release mechanisms by embedding drugs within a polymer matrix or using microcapsules that degrade over time. This precise control over how and when a drug is released into the system can result in increased patient compliance and treatment efficacy.
Consider several benefits of controlled release systems using microfluidics:
- Improved Drug Stability: Drugs can be protected from degradation, ensuring they remain effective until needed.
- Targeted Delivery: By customizing release profiles, drugs can be delivered more effectively to the site of action, enhancing therapeutic outcomes.
- Flexible Dosage Forms: Microfluidics allows for the fabrication of various dosage forms, such as patches or complex injectable formulations, making them suitable for varied treatment regimens.
In summary, both high-throughput screening and controlled release systems exemplify the pivotal role microfluidics plays in modern drug development. These innovations are not only improving the efficiency and safety of drug discovery but also paving the way for more personalized approaches to healthcare.
Personalized Medicine
Personalized medicine represents a significant leap forward in the biomedical field. It refers to tailoring medical treatment to the individual characteristics of each patient, taking into account their genetic makeup, lifestyle, and environment. In this context, microfluidic devices play a pivotal role by enabling precise manipulation of small volumes of fluids, which is critical for testing and analysis in personalized medicine.
The importance of personalized medicine cannot be overstated, as it brings a more nuanced and effective approach to healthcare. By focusing on the specific needs and conditions of individual patients, it has the potential to maximize therapeutic efficacy and minimize adverse effects. This level of customization can lead to better patient outcomes and reduced healthcare costs in the long run.
Tailored Drug Dosing
When it comes to tailored drug dosing, microfluidic devices offer innovative solutions for determining the optimal dose of medication for each patient. This involves using micro-scale technologies to conduct experiments that help understand how various drugs interact with individual biological systems. By employing these devices, researchers can assess the correct dosages needed for effective treatment.
Benefits of tailored drug dosing include:
- Enhanced efficacy: Tailored drug doses ensure that patients receive the right amount of medication based on their individual response, improving the likelihood of successful treatment.
- Reduced side effects: By refining dosages, patients are less likely to experience adverse effects commonly associated with standard dosing.
- Better adherence: When patients see good results from their treatment, they’re more inclined to stick with their medication plans.
Genetic Profiling
Genetic profiling is another cornerstone of personalized medicine that benefits greatly from microfluidics. By using these advanced devices, healthcare professionals can perform detailed analyses of a patient’s genetic material. This information can guide physicians in making informed decisions about the best treatment options available.
The integration of microfluidic technologies in genetic profiling allows for the processing of multiple samples simultaneously with minimal errors. This high throughput capability is vital for analyzing vast amounts of genetic data quickly and efficiently.
Key advantages of genetic profiling include:
- Precision in treatment: Understanding a patient’s genetic makeup helps in choosing drugs that are most likely to be effective based on their genetic variations.
- Identification of risks: Genetic profiling can reveal predispositions to certain diseases, allowing for preventive measures to be taken before the onset of the illness.
- Customization of therapies: Treatments can be adjusted based on genetic and molecular factors, leading to more successful outcomes.
"Personalized medicine aims to revolutionize healthcare by moving away from a one-size-fits-all approach to one that recognizes and respects individual differences."
In summary, personalized medicine is enhanced through the use of microfluidic devices, fostering a healthcare landscape that is more attuned to the individual. Microfluidics not only facilitate tailored drug dosing and genetic profiling but also set the stage for a future where healthcare becomes more precise, effective, and patient-centered.
Technological Innovations in Microfluidics
Technological innovations have paved the way for groundbreaking changes in the field of microfluidics, significantly impacting biomedical applications. The capacity to manipulate small amounts of fluids with high precision is not only a scientific marvel, but it also carries far-reaching implications for diagnostics, drug delivery, and even personalized medicine. With ongoing advancements in technology, microfluidic devices are emerging as indispensable tools in research laboratories and clinical settings.
Advances in Fabrication Techniques
Recent years have seen remarkable progress in fabrication techniques which have allowed scientists to create more complex and intricate microfluidic devices. Traditional methods like soft lithography have now been enhanced by novel approaches such as 3D printing and laser ablation.
These innovations enable researchers to design devices with greater flexibility and precision, allowing for designs that were previously beyond reach.
For instance, 3D printing offers a rapid prototyping solution that facilitates the modification of device designs with minimal downtime. Users can quickly iterate their prototypes to optimize their functionalities. On the other hand, laser ablation provides precise control over feature sizes, which enhances the capability to form intricate channel geometries. The result? Devices that are more efficient at mixing, separating, and analyzing samples than ever before.
There’s also a shift toward using biologically compatible materials, which is vital for clinical applications.
- Polydimethylsiloxane (PDMS): Widely used in manufacturing due to its flexibility and ease of use.
- Hydrogels: Emerging as a substance that can mimic biological environments, allowing for better interaction between cell cultures and devices.
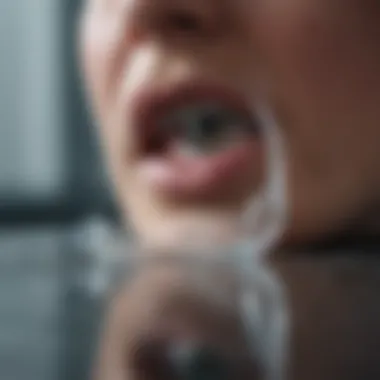
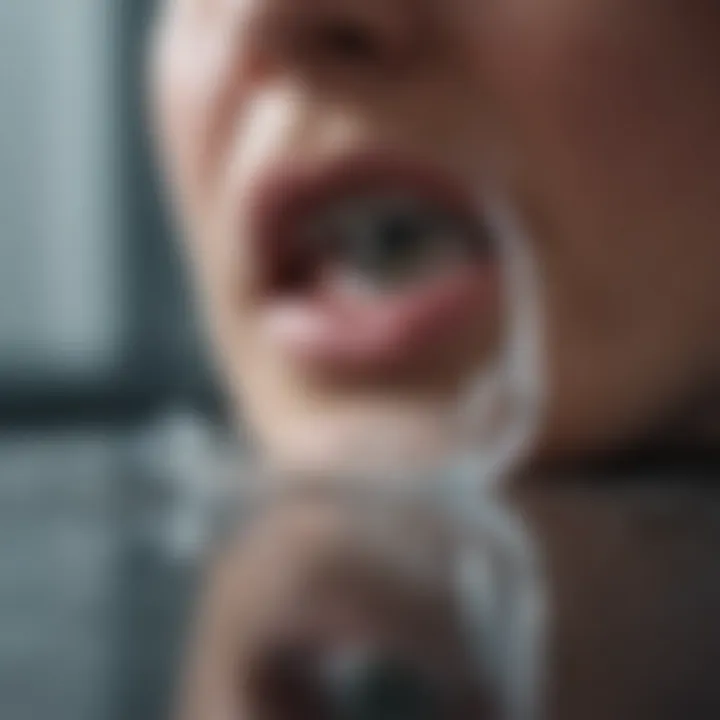
Key Benefits of these Advances:
- Enhanced throughput, reducing the time to gather results.
- Greater accessibility for small labs with limited resources.
- The ability to craft custom devices suited to specific research needs.
Integration with Other Technologies
The integration of microfluidics with other advanced technologies has opened new frontiers in biomedical research. The combination of microfluidic systems with technologies such as lab-on-a-chip, optical imaging, and biosensors enhances the capabilities of diagnostics and therapeutic applications.
- Lab-on-a-chip systems consolidate multiple laboratory functions into a single device, facilitating chemical reactions and analyses at a microscale. This reduces reagent consumption and minimizes waste, making processes both economical and eco-friendly.
- Optical imaging integration allows for real-time monitoring of reactions, offering instantaneous insights into experimental outcomes.
- Biosensors embedded in microfluidic devices enhance detection capabilities, enabling rapid identification of biomarkers.
This convergence of technologies allows for an unprecedented level of miniaturization and automation in various biomedical processes. The potential to streamline testing procedures could mean faster diagnoses, particularly in time-sensitive medical cases.
Considerations for Integration:
- Interfacing with existing laboratory protocols can be complex, requiring training and adaptation.
- Developing standard operating procedures is critical to ensure consistent outcomes across various users.
As we look towards the future, technological innovations in microfluidics continue to hold promise. The progressive merging of these sophisticated techniques undoubtedly creates a ripple effect, influencing every facet of biomedical science.
Challenges in Microfluidic Device Development
The development of microfluidic devices isn't all smooth sailing. Despite their transformative potential in biomedical fields, a host of challenges can impact their broader adoption and functionality. These challenges range from technical limitations to regulatory hurdles, both of which demand careful consideration by researchers and developers. Addressing these issues is fundamental not only for enhancing the devices themselves but also for ensuring that they effectively meet clinical needs and comply with industry standards.
Technical Limitations
At the heart of any technological evolution, limitations often shape the trajectory of progress. In microfluidics, technical limitations are aplenty, influenced by factors such as fluid dynamics, scaling issues, and material properties.
For example, the manipulation of fluids at the microscale comes with unique challenges. Unlike larger systems, fluids in micro-channels behave differently, often dictated by surface tension and viscosity. This phenomenon can lead to problems in maintaining precise control over fluid flow rates, thereby affecting the reliability of experiments and assays. Adapting known principles of fluid dynamics becomes a painstaking process, with researchers needing to rethink methodologies that worked well in traditional lab environments.
Moreover, material selection plays a pivotal role in performance. Common substrates like polydimethylsiloxane (PDMS) may exhibit limitations such as gas permeability, which impacts the longevity and efficacy of biological samples. Transparency for optical detection is often desired, but higher costs can come into play when moving towards more advanced materials like glass or silicone. This trade-off between performance and affordability can stifle innovation, particularly in smaller laboratories trying to operate on limited budgets.
"Technical hurdles are not just a mere inconvenience; they challenge the very nature of device functionality and reliability in a clinical context."
Regulatory Hurdles
The path to getting microfluidic devices from the lab bench to real-world applications is often mired in regulatory complexities. Entrusted with public safety, regulatory bodies impose stringent standards that developers must navigate.
Clearing regulatory pathways often involves extensive validation and documentation processes. Each device must demonstrate its effectiveness and safety, which requires rigorous testing across numerous conditions and environments. This can delay the commercialization of new devices significantly. Additionally, the lack of clear guidelines tailored specifically for microfluidic technologies can lead to confusion among manufacturers on how to meet these expectations.
One significant concern involves data security, given the nature of patient-related samples often processed by these devices. Developers must be vigilant about maintaining patient privacy and ensuring data is handled in compliance with established regulations, like HIPAA in the United States. Failing to adhere to these legal frameworks can lead to serious repercussions, further complicating the route to successful market entry.
These regulatory hurdles can sometimes feel like climbing a mountain. They require diligent planning and foresight to overcome, yet they are necessary to safeguard public health and trust in emerging technologies.
Navigating the landscape of microfluidic devices, it becomes clear that overcoming these challenges is not only about technical prowess but also about understanding the broader implications of their integration into healthcare. By recognizing and addressing the obstacles in development, we can pave the way for more effective, reliable, and widely adopted microfluidic devices in the biomedical arena.
Future Perspectives
The rapidly evolving landscape of microfluidic devices promises an exciting frontier for biomedical applications. Understanding the future perspectives of this technology is critical not just for current practitioners but also for students and researchers anticipating the next wave of innovations. The integration of microfluidic applications into mainstream healthcare opens doors to enhanced diagnostics, streamlined drug development, and tailored medical interventions. Key elements to consider include technological advancements, the shift towards personalized medicine, and the integration of these devices into existing healthcare frameworks.
Potential Developments
The potential developments in microfluidics are immense and multifaceted. One primary area of growth is the integration of artificial intelligence and machine learning with microfluidic systems. By leveraging data analytics in real-time with fluidic data, healthcare professionals can make better predictions and enhance diagnostic accuracy. The fusion of AI might also lead to the development of adaptive microfluidic systems, which adjust operational parameters based on incoming data.
Moreover, advancements in materials science are paving the way for more biocompatible and responsive devices. For instance, researchers are exploring polymers and hydrogel materials that offer greater flexibility in designing the channels and elements of microfluidic devices. These materials can enhance detection sensitivity in point-of-care tests, which are crucial in quick clinical decisions.
- Digital Microfluidics: An evolving segment that facilitates precise control via electrokinetic forces—leading to compact devices with multiple functionalities.
- Single-cell Analysis Techniques: A rising trend aimed at uncovering cellular heterogeneity that traditional methods overlook.
- Miniaturized Lab-on-a-Chip Systems: Enabling on-the-spot medical diagnostics that contribute to faster decision-making.
A Vision for Healthcare Integration
To truly harness the power of microfluidics, a well-coordinated vision integrating these devices into healthcare systems is essential. This vision must consider several aspects, including regulatory pathways, integration with existing technologies, and training for healthcare professionals.
The concept of point-of-care testing must evolve into a structured network, where microfluidic devices can seamlessly connect with electronic health records. By doing so, practitioners can access real-time patient data, enabling better and faster medical responses. Thus, improving patient outcomes hinges on this technology's ability to integrate smoothly with healthcare infrastructures.
Furthermore, partnerships between universities, private companies, and healthcare institutions will play a critical role in advancing the adoption and further research into microfluidic technologies. Collaborative efforts can lead to breakthroughs that address specific patient needs and healthcare challenges.
Key Considerations for Integration:
- Training Programs: To equip medical staff with the necessary skills to utilize these advanced tools.
- Regulatory Frameworks: Developing guidelines that ensure safety and efficacy without stifling innovation.
- Public Awareness Campaigns: To educate patients on the benefits and use of microfluidic technology in their healthcare.
"The future of microfluidic technology in healthcare is not just about better devices—it's about better lives."
In summary, the future perspectives on microfluidics signify a promising trajectory that is full of potential and opportunities. By focusing on technological advancements and integration into healthcare systems, professionals in the field can position themselves at the forefront of this burgeoning landscape.
Ethics and Microfluidics
The integration of ethics into the discourse around microfluidic devices is not just a minor detail; it impacts the very backbone of research and application in biomedical contexts. As these devices transform diagnostics, drug delivery, and personalized medicine, they raise various ethical considerations that warrant careful attention. Briefly putting it, what we do with technology can be just as significant as the technology itself. Recognizing the ethical dimensions helps in paving the way for responsible innovation and application.
Ethical Considerations in Research
When it comes to the ethical aspects of microfluidics in research, one must consider the implications of using these devices in settings involving human samples. Informed consent is a core principle that underlines ethical research, so it’s critical that researchers adequately inform participants about how their biological specimens may be used. This includes explaining any potential risks, benefits, and the future uses of the data obtained.
Additionally, there are concerns about the manipulation of biological materials. The accuracy of the outcomes derived from microfluidic devices can influence diagnoses, treatment plans, and research findings. If errors arise, the ramifications could be severe. Hence, researchers also have to establish transparency around their methodologies to gain trust from the public and the scientific community.
Some primary ethical considerations include:
- Transparency in Methods: Ensuring that research protocols are fully disclosed can prevent conflicts of interest.
- Respect for Human Dignity: Upholding the rights and dignity of those contributing samples must always be paramount.
- Benefit to Society: Assurance that findings from microfluidic research ultimately serve broader societal goals is essential.
The intersection of ethics and innovation offers an opportunity for constructive dialogue, shaping not only regulations but also the future trajectory of research processes toward more responsible outcomes.
Patient Privacy and Data Security
With the rise of microfluidic technologies comes an increase in the volume of sensitive data generated—be it genetic, biochemical, or diagnostic information. Managing this data responsibly is not just a legal obligation; it is also critical for maintaining trust between health professionals and patients. Privacy and data security are inextricably linked to ethical principles in healthcare, especially as microfluidic applications often involve real-time monitoring and analysis.
To bolster patient privacy, researchers and practitioners must adhere to strict protocols for data handling. This includes:
- Data Anonymization: Patient data should be stripped of personally identifiable information whenever possible.
- Secure Data Storage: Employing robust encryption techniques is essential for protecting sensitive information against unauthorized access.
- Clear Communication: Patients must be made aware of what data is being collected, how it will be used, and how it will be stored. This builds trust and empowers patients to make informed decisions regarding their health.
"Ensuring that data security measures are in place is not only an ethical responsibility but also an imperative for successful implementation of microfluidic technologies in healthcare."
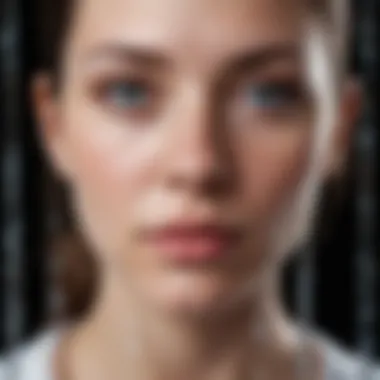
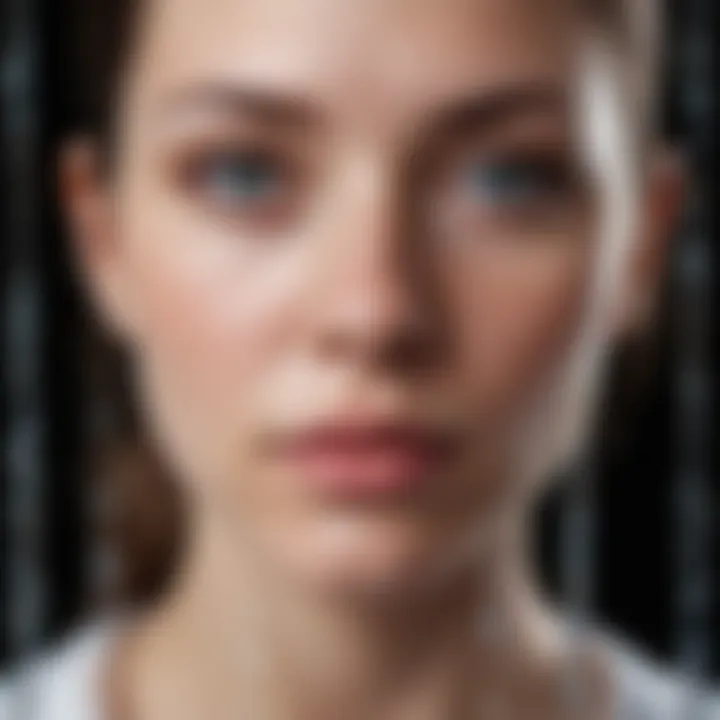
In summary, the ethical landscape surrounding microfluidic devices is multifaceted. As innovations push the boundaries of what is possible in medicine, a steadfast commitment to ethical research practices and data protection will certainly shape the future of microfluidics.
By prioritizing these ethical considerations, the field can continue to evolve responsibly, ensuring that advancements in technology translate into genuine benefits for society.
Comparative Analysis with Traditional Methods
Microfluidic devices represent a significant shift from traditional methods of handling fluids, particularly in the biomedical field. This comparison is vital, not only to understand the advantages of microfluidics but also to appreciate the contexts in which conventional techniques may still hold ground. Traditional methodologies often involve larger fluid volumes and are constrained by limitations in terms of speed, efficiency, and precision. As we dive into this analysis, various elements will come forward that highlight how microfluidic technology surpasses these age-old practices.
Advantages over Macrofluidics
Microfluidics offers several compelling advantages when compared to macrofluidic systems. First and foremost, it allows for precise manipulation of small volumes. This not only conserves reagents but also minimizes waste, which is quite crucial in high-stakes biomedical research. For instance, testing a single sample in a microfluidic device can often yield results that are comparable to, or even superior than, analyses done with larger sample sizes in traditional settings.
- High Throughput: Microfluidic devices can streamline workflows, enabling researchers to conduct multiple tests in parallel without the cumbersome steps associated with larger setups.
- Speed: Reactions that usually take considerable time in traditional systems can occur in a fraction of the time within microscale environments, thanks to enhanced mass transport and reduced diffusion distances.
- Integration Capability: Many microfluidic systems can seamlessly integrate various functions such as mixing, analyzing, and even dispensing fluids into one compact unit. In contrast, traditional systems often require multiple separate instruments.
Furthermore, the scale of microfluidics lends itself to increasing sensitivity in analytical measurements. For example, advancements in microfluidic design have allowed for techniques such as digital droplet PCR, which enhances the detection of low-abundance nucleic acids far beyond what traditional methods could achieve.
Limitations and Trade-offs
However, microfluidic devices are not without their challenges, and it’s essential to consider the limitations and trade-offs associated with this technology. For instance, the complexity of device fabrication can lead to variability that may not be present in traditional methods.
Some key points of limitations include:
- Fabrication Challenges: Creating microfluidic devices requires sophisticated techniques and expensive materials, which can pose financial hurdles for some labs.
- User Expertise: Operating these devices may demand a higher level of technical skill, which could be a barrier for wider adoption, especially in resource-limited settings.
- Scaling Issues: Although microfluidics excels in high-throughput scenarios, scaling up to fulfill larger volume requirements can be problematic. Traditional methods can handle larger volumes without requiring significant adjustments.
Another consideration is the potential for adaptation to clinical settings. While microfluidic devices have proven successful in research, their application in routine clinical settings has been slower due to regulatory standards and the need for validation. Traditional systems often enjoy the advantage of established protocols and longer histories of use that many institutions favor.
Global Perspectives on Microfluidics
Microfluidics represents a remarkable intersection of innovation and application across the globe, especially within the biomedical field. Its significance transcends geographical boundaries as different regions bring forth unique advancements and challenges. These global perspectives offer a rich tapestry of understanding that underscores the collaborative efforts in this domain.
Describing microfluidic devices often brings to mind images of intricate networks allowing for small volumes of fluids to be manipulated with precision. But on a larger scale, the implications of these devices touch on healthcare access, patient outcomes, and even economic growth in various countries. When we consider regional developments and collaborative efforts, we see a concerted push towards harnessing the full potential of these devices. This is not merely a tech endeavor; it's about addressing healthcare inequalities, enhancing diagnostic capabilities, and transforming drug development processes.
Regional Developments
Different regions have unique strengths when it comes to microfluidics.
- North America has established itself as a leader in innovation, primarily through universities and skilled startups. Institutions like MIT and Stanford are at the forefront, pushing the boundaries of what's possible. Commercialization efforts are robust, resulting in a thriving ecosystem where research swiftly transitions into market-ready products.
- Europe showcases a collaborative spirit, with many countries pooling resources for research and development. Initiatives like Horizon 2020 encourage cross-border collaborations, fostering advancements that are beneficial for all involved. Notable developments focus on integrating microfluidics with diagnostic platforms, ensuring that innovations can directly impact patient care.
- Asia, with its burgeoning biotechnology sector, particularly in countries like China and Japan, is rapidly adopting microfluidic technology to enhance healthcare access. The focus here is often on affordable solutions that can reach diverse populations. New facilities are springing up, dedicated to leveraging microfluidics for diagnostics and personalized medicine, thus aligning with global health goals.
Understanding these regional strengths helps inform best practices and can lead to enhanced outcomes in biomedical applications.
Collaborative Efforts and Consortia
The importance of collaboration in the microfluidics landscape cannot be overstated. Academic institutions, industry players, and government agencies often join forces in consortia to tackle the burgeoning challenges of healthcare.
"No one entity can create transformative healthcare solutions alone. It requires the collective expertise of various stakeholders working in harmony."
- Joint Ventures: Partnerships between companies exploit complementary skills. For example, a start-up specializing in technology might partner with a pharmaceutical company to develop a drug delivery system, harnessing microfluidic technology for more effective medication dispersal.
- Research Networks: Collaborative research networks are rising, exemplified by initiatives like the Microfluidics Consortium, which fosters communication among researchers globally, sharing findings and techniques to push forward the development of microfluidic devices.
- Funding Initiatives: Governments are increasingly recognizing the potential of microfluidics for boosting their healthcare systems. Funding programs are often directed towards collaborative projects aimed at solving pressing healthcare challenges through innovative technologies.
In summary, the global perspective on microfluidics reflects a unifying thread of dialogue and innovation across various regions. As researchers, educators, and healthcare professionals navigate this evolving landscape, it becomes evident that fostering collaboration will be essential for harnessing the full power of microfluidic devices in biomedical applications.
Case Studies in Microfluidic Applications
Understanding how microfluidic devices perform in real-world scenarios is crucial for grasping their potential impact. Case studies provide tangible examples that showcase not just the technical feasibility of these devices but also their application in addressing complex biomedical challenges. By examining both success stories and lessons from failures, one can glean insights into the benefits, limitations, and broader implications of microfluidic technologies in healthcare and research.
Successful Implementations
Microfluidic devices have made significant strides in numerous biomedical applications, yielding noteworthy case studies that illuminate their efficiency and versatility. One such success can be seen in the realm of cancer diagnostics. Researchers developed a microfluidic platform capable of isolating circulating tumor cells. This system not only enhances the sensitivity of cancer detection but also allows real-time monitoring of therapeutic effects. Such implementations can potentially revolutionize how oncologists address treatment options and patient management.
Another compelling example is the use of microfluidic devices in the realm of personalized medicine. A notable project involved the creation of a microfluidic chip designed for rapid metabolic profiling of individuals. This chip facilitated the screening of various enzymes and metabolic markers, allowing for tailored drug therapy plans based on a patient’s unique metabolic profile. The outcome not only increased the efficiency of treatments but also minimized adverse reactions, showcasing the scalability of microfluidic applications in personalized healthcare.
Failures and Lessons Learned
While there are numerous success stories, it's equally important to consider unsuccessful microfluidic implementations to gain a rounded perspective on the technology’s limitations. One illustrative case involved a microfluidic device designed for large-scale immunoassays. Initially, the goal was to streamline the process of analyzing protein interactions in biological samples. However, the system consistently failed to achieve reproducible results, largely due to inadequate fluid management and sensor integration.
This failure highlighted critical aspects to consider for future designs: the importance of precise fluid dynamics in microchannels and the integration of robust sensor technology. Developers learned that simply miniaturizing existing technologies does not guarantee success. It emphasizes that a thorough understanding of microfluidic principles and rigorous testing is essential before implementing these devices for clinical use.
In summary, the examination of case studies in microfluidic applications unveils the dynamic landscape of opportunities and challenges. Each successful implementation drives innovation forward, while failures underscore the necessity for continuous improvement and adaptation. As microfluidics evolves, both success stories and setbacks will inform the development of next-generation biomedical devices, ensuring that they meet the rigorous demands of healthcare.
End
The final segments of a study often serve as a captain’s log, charting the waters traveled and plotting the course ahead. This article not only illuminated how microfluidic devices stand at the forefront of biomedical applications but it also stressed their undeniable significance in advancing healthcare and research. As we draw the curtains on this discussion, several crucial elements come into focus.
Summary of Findings
The profound impact of microfluidic technology permeates various areas, particularly diagnostics, drug development, and health care integration. Key takeaways include:
- Precision in Manipulation: Microfluidics allows unmatched control over fluid dynamics, proving crucial in creating accurate diagnostics and personalized medicine.
- High Throughput Capability: The ability to conduct multiple analyses in parallel revolutionizes traditional methods, making processes faster and more efficient.
- Reduction in Sample and Reagent Use: With micro-level operations, the consumption of valuable resources is minimized, presenting a cost-effective solution in research and clinical settings.
- Versatility: The adaptability of microfluidic devices to various applications—ranging from early-stage drug discovery to tailored patient therapies—highlights their transformative potential in medicine.
Overall, the exploration of these findings underlines microfluidics as not just a tool but a catalyst for change, spurring innovation across the biomedical field.
Future Directions
Looking ahead, microfluidic devices appear poised for further breakthroughs, resonating strongly with the ongoing evolution of healthcare technology. Future directions include:
- Integration with AI and Data Analytics: The fusion of artificial intelligence with microfluidic operations could enhance decision-making processes by providing real-time data analysis for more precise diagnostics and personalized treatments.
- Customization for Patient-Specific Needs: Development will likely steer towards more personalized devices that adapt to individual physiological characteristics, ensuring optimized therapeutic interventions.
- Sustainability Initiatives: As environmental concerns mount, future innovations will focus more on sustainable practices in microfluidic device production and application.
- Enhanced User Accessibility: Making these sophisticated devices user-friendly and accessible to smaller laboratories and clinics can democratize the benefits of microfluidics, ensuring that advancements reach a broader audience.
Importance of References in This Article
Given the rapidly evolving nature of microfluidic technologies, substantiating claims with solid references is imperative. Here, references function not simply as a list of sources; they enrich the discourse, enabling readers to verify concepts and dive deeper into specific studies that have paved the way in this field. For instance, works published in journals like Lab on a Chip and Biomicrofluidics are indispensable because they showcase cutting-edge research and innovations.
Specific Elements of References
- Establishing Context: References help in placing the discussion within a broader context, illustrating how microfluidic devices have evolved over time and how their applications have expanded.
- Supporting Claims: Each assertion regarding the efficacy of microfluidics in diagnostics or personalized medicine is bolstered by research studies that validate such claims. This makes the argument not only compelling but also scientifically sound.
- Connecting Ideas: By citing a range of studies, one can interconnect various concepts. For instance, findings related to drug delivery systems can intertwine with personalized medicine practices, illustrating a cohesive narrative.
- Encouraging Further Exploration: Well-placed references invite readers to explore beyond the article, increasing their understanding and potentially inspiring future research.
"References act as the backbone of academic writing, providing structural integrity to the claims made."
Benefits of Adequate Referencing
- Enhances Credibility: A well-referenced article speaks volumes about the author’s commitment to quality and accuracy, attracting a discerning readership that values factual integrity.
- Guides Future Research: References highlight gaps in existing studies, guiding other researchers toward avenues of exploration that remain unexplored.
- Facilitates Knowledge Sharing: By pointing readers to original sources, references democratize information and promote knowledge sharing across disciplines, which is particularly vital in interdisciplinary fields like biomedical engineering.
Considerations About References
In constructing a list of references, one must consider the reliability and relevance of each source. Peer-reviewed articles are generally favored over non-peer-reviewed materials for their rigorous evaluation standards. Furthermore, it's beneficial to ensure a balance between foundational texts and contemporary studies that explore current advancements.