Metafiber: The Future of Advanced Materials and Technology
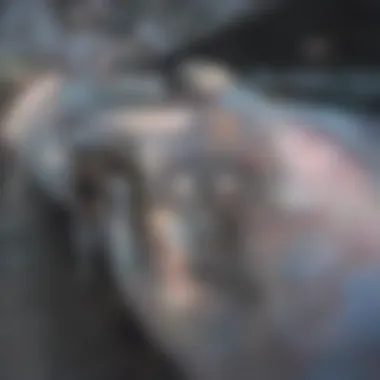
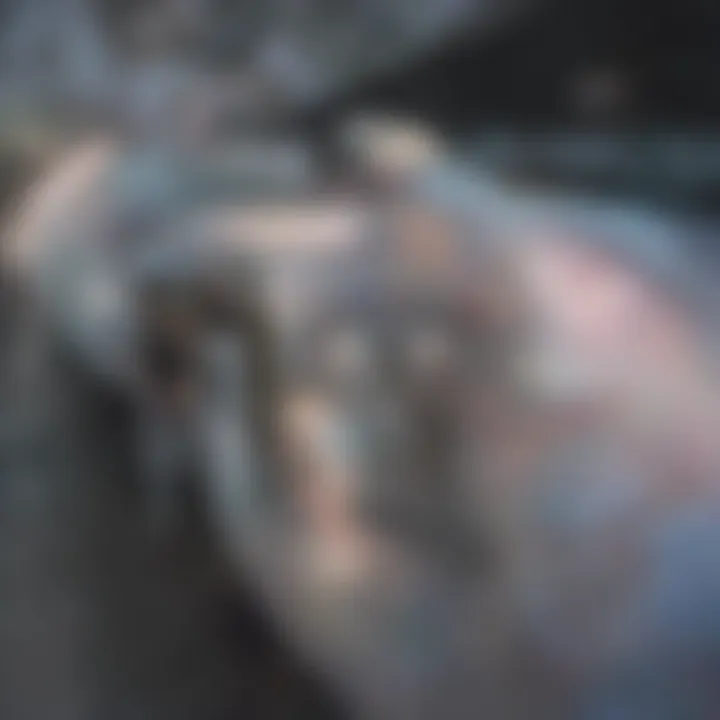
Intro
In recent years, the intersection of techology and material science has catalyzed significant advancements in various fields. One concept that arises in this discourse is metafiber. Metafiber is more than a mere material; it represents the confluence of traditional fibers and cutting-edge technologies. This innovative material has the potential to revalidate longstanding practices in textiles, biomedical engineering, and sustainability.
The journey through metafiber entails an exploration of its composition. Understanding what metafiber is made of is crucial. It encompasses fibers imbued with functionalities that were once considered the realm of electronics or specialized domains. This hybrid nature opens the door to numerous applications that can reshape industries.
In the sections that follow, we will delve deeper into the aspects of metafiber, such as its production processes and real-world applications. Key findings about its significance will be presented alongside implications for research and future advancements. Understanding metafiber is paramount for students, researchers, educators, and professionals.
Metafiber is poised to redefine the paradigm of how fibers can be utilized, and an exploration of this innovative material reveals its transformational potential in advancing multiple sectors. Many industries stand on the brink of revolution thanks to metafiber's integration. As we continue through this article, the relevance and importance of metafiber will become even clearer.
Understanding Metafiber
Metafiber stands at the crossroads of material science and advanced technology. Its importance lies in how it redefines our understanding of materials and their applications. This section aims to elucidate the concept of metafiber—what it is, how it evolved, and where it stands in contemporary research.
A foundational grasp of metafiber benefits various stakeholders. This includes students, researchers, and professionals in fields like textiles, biomedical engineering, and environmental science. Understanding its properties and applications can aid in developing innovative solutions, enhancing productivity, and fostering sustainability.
Definition and Origin
Metafiber is a term that refers to a new class of fibers. These fibers exhibit properties that are enhanced through the integration of advanced technologies. The origin of metafiber can be traced back to the early attempts to combine traditional textile materials with smart technologies. Initially, researchers experimented with fibers that could conduct electricity or change properties in response to environmental stimuli.
Historical Context
The history of metafiber can be linked to both the development of synthetic materials and advancements in nanotechnology. In the late 20th century, the demand for smarter textiles rose, prompting research into multifunctional fibers. This trend continued with significant innovations such as conductive polymers and bioresponsive materials that paved the way for metafiber. The exploration of these fibers has been fueled by industries looking for lightweight, durable, and intelligent materials.
Current Research Landscape
Today, the research landscape surrounding metafiber is dynamic and rapidly evolving. Academic institutions and research organizations are heavily involved in investigating the potential of metafibers across various disciplines. Current studies focus on:
- Optimizing Material Composition: Researchers are exploring different combinations of materials to enhance performance.
- Functionality Enhancements: Study on integrating sensors and actuators into fibers is ongoing, which enables the creation of smart textiles.
- Application Developments: Industries are experimenting with metafiber in products ranging from athletic wear to medical devices, observing how these materials can improve functionality and user experience.
"The interdisciplinary nature of metafiber research exemplifies the innovative spirit in modern material science."
In summary, the understanding of metafiber encompasses its definition, historical evolution, and current advancements in research. This knowledge serves as a cornerstone for examining its implications further in the fields of technology and material science.
Composition of Metafiber
The composition of metafiber is essential to its performance and relevance across various fields. By understanding the unique materials and structures that create metafiber, researchers and professionals can fully harness its potential. Metafiber combines advanced materials technology with traditional fiber science, offering numerous benefits in terms of performance, durability, and functionality.
Core Materials
The core materials of metafiber are fundamental to its properties. Typically, it is composed of a blend of synthetic and natural fibers. Polyester and nylon are popular choices for their strength and versatility. These materials are often combined with cellulose-based fibers to enhance biodegradability and comfort. Recent advancements have introduced biopolymers and nanomaterials into the mix. This fusion results in a material that is not only strong but also lightweight and adaptable. Researchers focus on identifying the best combinations to enhance the performance of metafiber in specific applications.
Structural Attributes
The structural attributes of metafiber are what distinguish it from traditional fibers. Unlike conventional fibers that primarily focus on tensile strength, metafiber offers a unique multi-dimensional structure. This structure enhances flexibility, making it suitable for various applications. For example, the alignment of fibers at the microscopic level contributes to both its mechanical strength and resistance to wear.
"Understanding the structural properties of metafiber is crucial for its ability to meet industry demands for more durable and efficient materials."
By optimizing the structural configuration, manufacturers can create metafiber that meets specific industry requirements, from textiles to electronics. The balance between strength, elasticity, and lightweight properties is paramount in achieving high functionality.
Functional Properties
Functional properties are where metafiber truly shines. These properties range from moisture management to thermal regulation. Because of the incorporation of specialized materials, metafiber can provide signaling capabilities, integrating well with smart technologies. The moisture-wicking ability is exceptional, allowing for greater comfort in wearables and athletic gear. Moreover, certain metafibers have antimicrobial properties, making them suitable for biomedical applications.
In essence, the functional properties of metafiber not only make it appealing for current markets but also set the stage for innovative applications in the future. Its versatility opens doors to previously unimaginable uses. As the demand for intelligent and sustainable materials increases, so does the importance of understanding and developing the composition of metafiber.
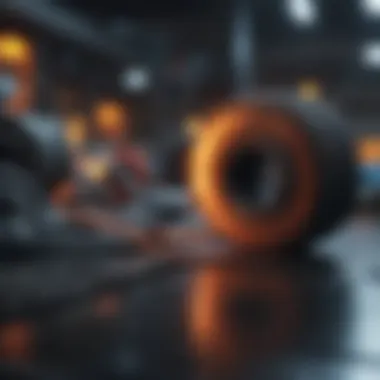
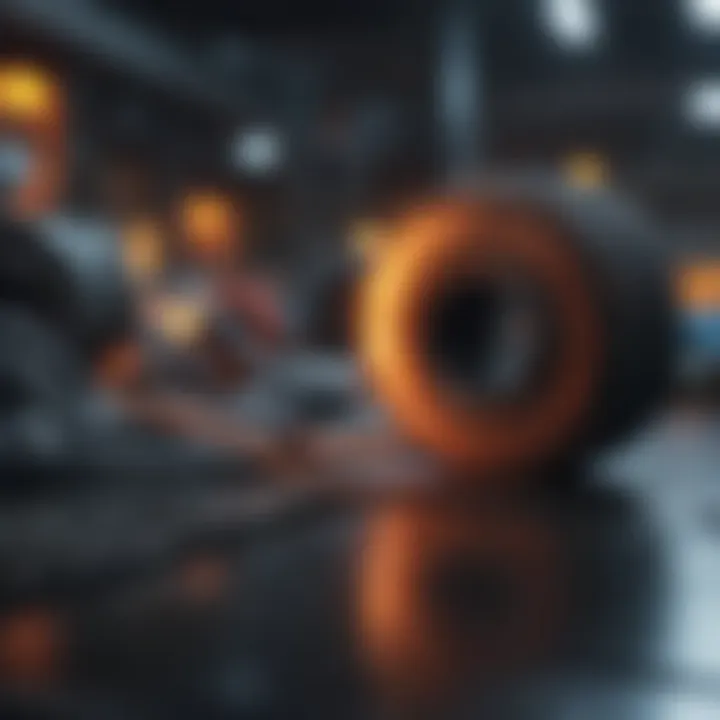
Production Techniques
Understanding the production techniques related to metafiber is crucial for appreciating its unique characteristics and potential applications. The processes involved in creating metafiber not only determine its quality but also impact its environmental sustainability and scalability in various industries. By focusing on the advancements in manufacturing, quality control, and efficiency, we can gain insight into how metafiber stands at the intersection of technology and material science.
Manufacturing Processes
Manufacturing processes for metafiber are sophisticated yet critical. These processes transform raw materials into functional fibers with enhanced properties. The most common techniques include electrospinning, melt spinning, and solution spinning. Each of these methods offers distinct advantages and limitations.
- Electrospinning involves using an electric field to draw fibers from a solution. This technique is advantageous because it produces very fine, uniform fibers that can be manipulated into various shapes and functionalities.
- Melt spinning is employed primarily for thermoplastic materials. Here, raw materials are heated until they melt and then extruded to form continuous filaments. This method favors mass production due to its speed, but it may not achieve the same level of fiber finesse as electrospinning.
- Solution spinning requires dissolving materials in a solvent before processing. This method allows for greater control over fiber properties, but the solvents must be managed carefully due to environmental concerns.
Each manufacturing process affects the mechanical and physical properties of the resulting metafiber, which can be tailored to specific applications in industries such as textiles and biomedical engineering.
Quality Control Measures
Quality control is essential in the production of metafiber. As fibers are increasingly used in critical applications, including medical devices and smart textiles, stringent measures need to be in place to ensure performance and reliability. Key measures include:
- Testing for Mechanical Properties: Tensile strength and elasticity tests help determine whether the metafiber can withstand operational stresses.
- Microscopic Analysis: Observing the fiber morphology using scanning electron microscopy ensures that the fibers meet the specified diameter and surface features.
- Chemical Composition Analysis: This includes assessing the purity of raw materials and examining any additives or treatments applied during production.
Implementing these quality control measures helps maintain consistency and reliability, which is crucial for the multifunctional applications of metafiber.
Scale and Efficiency
The scale of production directly impacts the accessibility of metafiber technologies. Companies aim to enhance efficiency not just to reduce costs but also to address sustainability concerns.
Several strategies can improve processing efficiency:
- Automation: Utilizing automated systems in manufacturing processes can reduce human error and increase output while maintaining quality.
- Material Recycling: Using recycled materials or incorporating recycling into production processes minimizes waste. This aspect aligns with broader sustainability goals.
- Research and Development: Ongoing R&D facilitates the discovery of new materials and processing techniques that can enhance fiber qualities while optimizing production.
The interplay between scale and efficiency of production impacts the overall marketability and viability of metafiber as a solution across multiple industries.
Applications in Various Industries
The significance of metafiber in various industries is profound. Its unique combination of traditional fiber characteristics and advanced technological features introduces new dimensions to sectors like textiles, medicine, environment, and electronics. These applications not only demonstrate the versatility of metafiber but also highlight its potential to drive innovation and efficiency in multiple domains.
Textiles and Fashion
Metafiber is revolutionizing the textiles and fashion industry by enhancing both functionality and aesthetics. The incorporation of metafiber into clothing can lead to fabrics that are not only lightweight and strong but also capable of moisture-wicking and antibacterial properties. For instance, brands now use metafiber to create sportswear that actively responds to the wearer's physiological needs.
Benefits
- Enhanced Performance: Fabrics made from metafiber can improve athletic performance through moisture management and breathability.
- Sustainability: The production of metafiber can utilize recycled materials, contributing to a circular fashion economy.
These innovations are crucial as consumers increasingly look for sustainable and high-performance clothing choices, making metafiber a pivotal player in the future of fashion.
Biomedical Engineering
In biomedical engineering, metafiber presents exciting possibilities. Its ability to be engineered at the molecular level allows for applications such as biosensors and drug delivery systems. Metafiber's biocompatibility makes it suitable for applications in implants and prosthetics, where it can support tissue regeneration.
Applications
- Tissue Engineering: Metafiber can be designed to resemble natural tissue structures, promoting cell growth and integration.
- Wearable Health Monitors: Due to its lightweight and flexible nature, it is ideal for creating sensors that monitor health metrics in real-time.
These enhancements not only improve patient outcomes but also reduce recovery time and increase overall healthcare quality.
Environmental Solutions
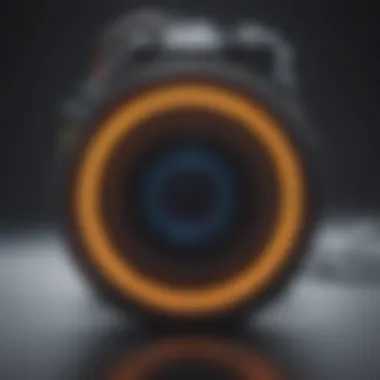
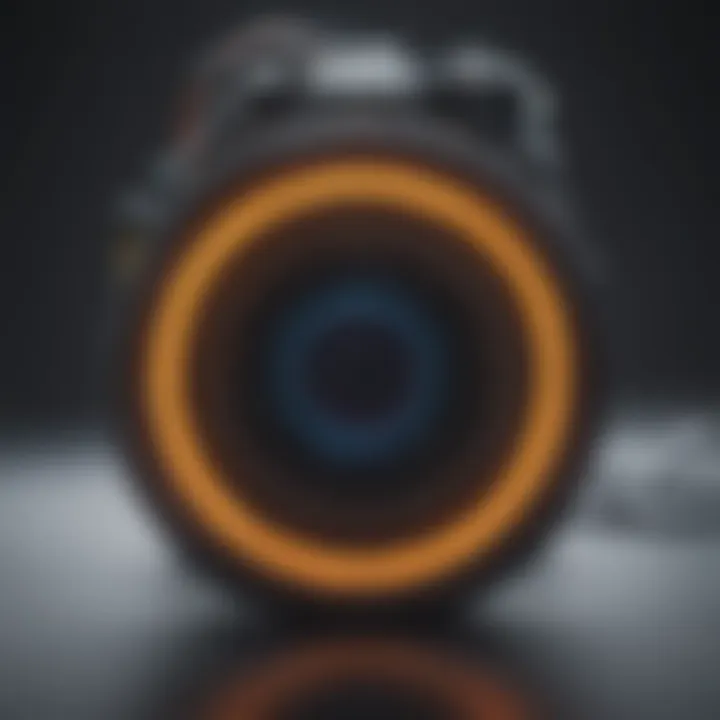
The environmental applications of metafiber are particularly noteworthy. Given the growing concerns about waste and pollution, metafiber can be developed with properties that promote ecological sustainability. For example, leveraging metafiber technology can lead to the production of biodegradable materials that break down more easily than conventional plastics.
Key Impacts
- Pollution Reduction: Metafiber-based products can decrease reliance on non-biodegradable materials, thus minimizing pollution.
- Resource Management: The utilization of renewable resources in metafiber production supports a circular economy.
Adopting metafiber solutions can greatly assist industries in meeting sustainability goals while addressing global environmental challenges.
Electronics and Smart Fabrics
In the realm of electronics, metafiber presents innovative opportunities. The development of smart fabrics woven with metafiber can integrate technology into everyday textiles. This integration allows fabrics to respond to environmental stimuli and collect data, paving the way for a more interconnected world.
Innovative Integrations
- Wearable Technology: Fabrics with embedded sensors can monitor physiological responses, fostering advancements in personal health tracking.
- Interactive Clothing: Clothing that interacts with smartphones and other devices through metafiber technology enhances user experience and functionality.
The prospects of combining electronics and textiles through metafiber are endless, positioning it as a cornerstone of the future of smart technology.
Impact on Sustainability
The concept of sustainability is critical in today's technological advancements, especially in materials science. Metafiber stands out due to its potential to significantly impact sustainability across multiple dimensions. By exploring how metafiber interacts with resource efficiency, recyclability, and overall environmental footprint, we can better understand its role in driving forward an eco-friendlier future.
Resource Efficiency
Resource efficiency refers to achieving more output from less input, a principle that is increasingly vital in material production. In the case of metafiber, the focus is on optimizing the use of raw materials. The core materials used in metafiber are often sourced sustainably, which minimizes the depletion of natural resources.
Notably, metafiber manufacturing typically employs processes that use less energy compared to traditional fiber production. Such methods reduce waste and emissions, contributing to a more sustainable lifecycle. Continuous innovation in production techniques enhances the efficiency of resource utilization. This not only benefits manufacturers but ensures that future generations have access to essential materials.
Recyclability and Circular Economy
Recyclability is a crucial factor in sustainability. Certain versions of metafiber are designed to be recycled at the end of their life cycle. This property is particularly beneficial in promoting a circular economy, where products are reused, remade, and recycled. When users can recycle metafiber, it reduces waste and the need for virgin materials, meeting both economic and ecological goals.
- Recycling Initiatives: Industry partners work to establish programs that facilitate the return and recycling of metafiber products, incentivizing such practices.
- Design for Disassembly: Metafiber can be engineered to allow easy separation of components, making recycling simpler and more efficient.
Environmental Footprint
The environmental footprint of any material includes the sum of all environmental impacts associated with its production and disposal. For metafiber, this footprint can be considerably lower than traditional materials due to its resource-efficiency attributes and recyclability. Metafiber often utilizes less water and energy, which decreases its carbon footprint during the production process.
There are opportunities for improvements yet. Systematic assessments are necessary to constantly evaluate and minimize the environmental impact. Users and manufacturers must ensure that the entire lifecycle of metafiber—from raw material sourcing, manufacturing, use, and disposal—is considered. This holistic approach will promote continual reductions in environmental footprints and foster a greater commitment to sustainability in textiles and other industries.
"Sustainability is not just a goal but a necessity for future generations; metafiber exemplifies this ethos in material science."
Through these aspects, metafiber demonstrates its importance in impacting sustainability. The dialogue around sustainability in material science must continue to evolve, aligning with these innovations to meet global demands.
Technological Innovations
Technological innovations stand at the core of metafiber's transformative potential. This section will explore advancements that redefine material science, often blurring the lines between functionality and design. The successes achieved through technological integration not only enhance the performance of metafiber but also expand its applicability across various industries. Understanding the implications of these innovations provides crucial insights into metafiber’s future and its role in shaping modern materials.
Nanotechnology and Metafiber
Nanotechnology plays a critical role in the development of metafiber. At the nanoscale, materials can exhibit unique properties that are quite different from their bulk counterparts. For metafiber, incorporating nanotechnology facilitates enhancements in strength, durability, and responsiveness.
- Increased Strength: Nanomaterials can bolster the structural integrity of fibers, making them significantly stronger without adding weight. This benefit is particularly useful in applications requiring strong, lightweight materials.
- Functionalization: Nanotechnology allows for the manipulation of surface properties, enabling features such as water resistance, UV protection, and antimicrobial functions. These attributes broaden the use cases in everyday textiles and medical applications.
- Customization: Tailoring properties at the nanoscale enables the creation of fibers suited for specific environments. This adaptability simplifies designs that would otherwise require complex engineering solutions.
The integration of nanotechnology not only elevates performance standards but also aligns with sustainability goals. Lesser material usage combined with enhanced capabilities means reduced waste and more efficient production processes.
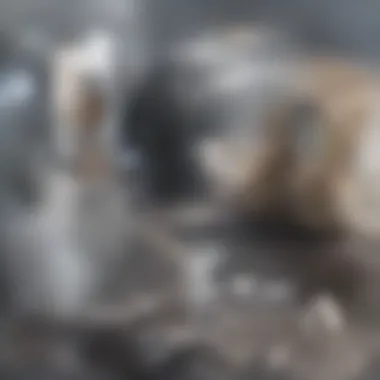
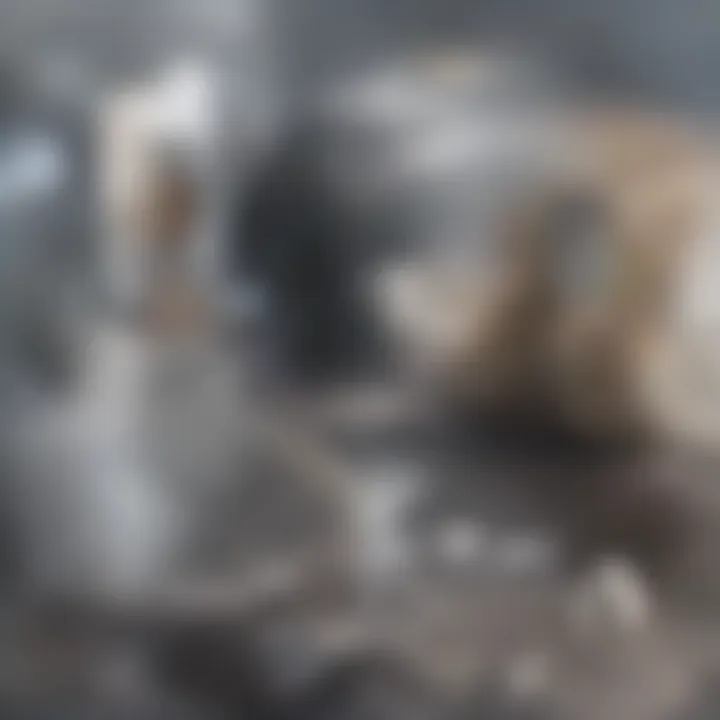
Smart Integrations
Smart features within metafiber are becoming increasingly prevalent. These integrations refer to the ability of fibers to communicate, respond, and adapt to their surroundings. Advances in sensor technology play a pivotal role in this evolution.
- Wearable Technology: Metafiber can be embedded with sensors that monitor biometric data, enabling real-time health assessments. This application has profound implications for the biomedical field, bridging the gap between fabric and personal health monitoring.
- Adaptive Textiles: Fabrics capable of reacting to environmental stimuli create novel opportunities. For instance, textiles that change color based on temperature or moisture levels can enhance user experience and functionality in various sectors.
- Energy Harvesting: Innovative fibers that can capture energy from motion or environmental sources are emerging. This characteristic is crucial for powering small devices and contributes to the push for self-sustaining technologies.
Smart integrations not only enhance user interaction with products but also foster new business models and markets, signifying a paradigm shift in material use and functionality.
Future Technological Trends
Looking ahead, several trends emerge that signify the future direction of metafiber within technological innovation. These trends suggest a landscape that is richer in possibilities while aligning with global sustainability goals.
- Biomimicry: This concept involves designing materials that imitate nature’s processes. Incorporating biological principles into the development of metafiber can lead to more efficient materials that are inherently sustainable and self-repairing.
- Advanced Manufacturing Techniques: Innovations like 3D printing and additive manufacturing are set to revolutionize how metafibers are produced. These methods allow for more complex designs, minimized waste, and on-demand production.
- Interdisciplinary Collaboration: Future advancements will increasingly depend on collaboration across disciplines, including biotechnology, robotics, and material science. Such collaboration fosters holistic approaches to problem-solving and product development.
As these trends materialize, they will drive the evolution of metafiber beyond current limitations. The influence of these innovations extends not only within technical realms but also impacts societal norms and environmental practices, reinforcing the importance of material science in addressing contemporary challenges.
Future Directions and Challenges
The exploration of metafiber is crucial for understanding how this innovative material can influence various sectors while presenting unique challenges. The future directions in metafiber development are not only about advancements in technology but also entail navigating the intricate landscape of market dynamics, regulatory frameworks, and scientific limitations. By addressing these challenges, stakeholders can harness the full potential of metafiber, ensuring it evolves to meet the demands of tomorrow's industries.
Market Opportunities
The market opportunities surrounding metafiber are broad and diverse. Industries such as textiles, healthcare, and environmental management are already recognizing the potential benefits of incorporating metafiber into their operations. As manufacturers seek to improve the performance and sustainability of their products, metafiber stands out for its unique properties.
- Textile Innovation: Clothing manufacturers can explore metafiber's application in producing lighter, stronger, and more sustainable fabrics. This represents a step forward in fashion, moving away from traditional materials.
- Biomedical Uses: In medical devices and implants, metafiber can contribute to better biocompatibility and functionality. This can lead to improved patient outcomes and revolutionary healthcare solutions.
- Environmental Solutions: Metafiber may play a significant role in developing eco-friendly materials or products. Its potential for biodegradability or recycling positions it as a vital component in efforts toward sustainability.
Exploring these opportunities requires a proactive approach from industries. Businesses that invest in research and development related to metafiber can position themselves ahead of competitors, tapping into growing market segments driven by sustainability and innovation needs.
Regulatory and Ethical Considerations
As metafiber technology progresses, understanding regulatory and ethical implications becomes increasingly important. Regulatory frameworks surrounding new materials often lag behind technological advancement, creating challenges for companies seeking to commercialize metafiber products. Important considerations include:
- Compliance with Safety Standards: Ensuring metafiber materials meet existing health and safety regulations is critical, especially in medical and consumer products.
- Consumer Transparency: Ethical production and transparency about sourcing and manufacturing processes will be integral for building trust with customers.
- Environmental Impact Assessments: As metafiber products enter the market, their environmental impact must be assessed to ensure that they contribute positively to sustainable practices.
Navigating this landscape requires collaboration between manufacturers, regulators, and stakeholders to develop appropriate regulations that protect consumers while promoting innovation.
Scientific and Practical Limitations
Scientific and practical limitations pose significant barriers as research on metafiber continues. These factors may slow down advancements, especially when transitioning from laboratory settings to real-world applications.
- Material Durability: While metafiber shows promise, questions remain regarding its long-term durability and performance under various conditions. Studies are necessary to assess how it behaves over time under stress.
- Scalability of Production: Developing scalable manufacturing processes that maintain quality and reduce costs is a challenge that needs addressing. Without cost-effective production, metafiber may struggle to compete with established materials.
- Interdisciplinary Collaboration: The advancement of metafiber demands knowledge from multiple fields, including material science, engineering, and biotechnology. Coordinating efforts among these disciplines can be complex but is essential for overcoming scientific hurdles.
End
The conclusion section holds significant weight in discussing the topic of metafiber. It serves as a defining moment to synthesize the vast topics covered throughout the article. As we explored the concepts of metafiber, from its composition to its applications, we have seen that this innovative material stands as a prime example of the fusion of technology and material science.
It is essential to recognize the key insights gathered along the way. Metafiber is not just a remarkable material but an avenue for future innovations that can potentially revolutionize several industries. The cross-disciplinary applications in textiles, biomedical engineering, and environmental initiatives highlight its versatility and commercial relevance. This material does not merely represent advancements in production techniques but also a shift in how we think about sustainability and material efficiency in manufacturing.
Additionally, considerations around metafiber propel discussions on the ethical and regulatory frameworks needed as this field evolves. The implications for industries and research communities are profound. They require not just adaptation to new technologies but also a rethinking of design, application, and environmental considerations. As we consolidate our understanding of metafiber, one cannot overlook its potential to significantly reshape manufacturing and consumption patterns across multiple sectors.
Summary of Key Insights
- Versatility: Metafiber combines the attributes of various materials, making it suitable for diverse applications.
- Sustainability: There are significant benefits to resource efficiency and the circular economy. Metafiber can offer solutions that align with global sustainability goals.
- Technological Integration: The integration of technologies such as nanotechnology enhances metafiber's functional properties, creating new opportunities in smart textiles and other areas.
Through the exploration of these insights, it is clear that research into metafiber paves the way for critical advancements in material science. The synthesis of technology with materials leads to innovations that resonate beyond mere functionality.
Implications for Future Research
Future research directions must focus on understanding the limitations and opportunities presented by metafiber. Such investigations should assess the reliability, cost-effectiveness, and scalability of metafiber production processes.
- Market Dynamics: Analysis of various markets where metafiber can be introduced or adopted to fill gaps or enhance performance.
- Regulatory Framework: Establishing standards for metafiber, ensuring safety and sustainability compliance across industries.
- Interdisciplinary Collaboration: Encouraging collaboration between material scientists, technologists, and manufacturing experts can propel future innovations beneficial for both researchers and practitioners in the field.
Ultimately, our exploration of metafiber concludes with a recognition of its complexity and potential. As the intersection between technology and material science continues to evolve, metafiber remains at the forefront, prompting valuable discussions and research initiatives that will shape the landscape of multiple sectors.