Holy Hydrogen: Exploring Its Future in Energy
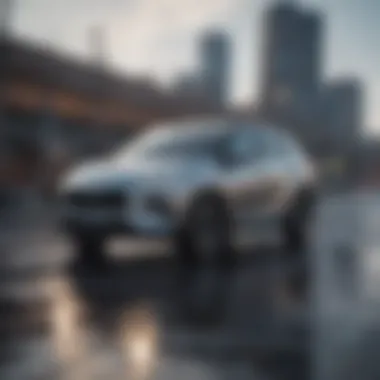
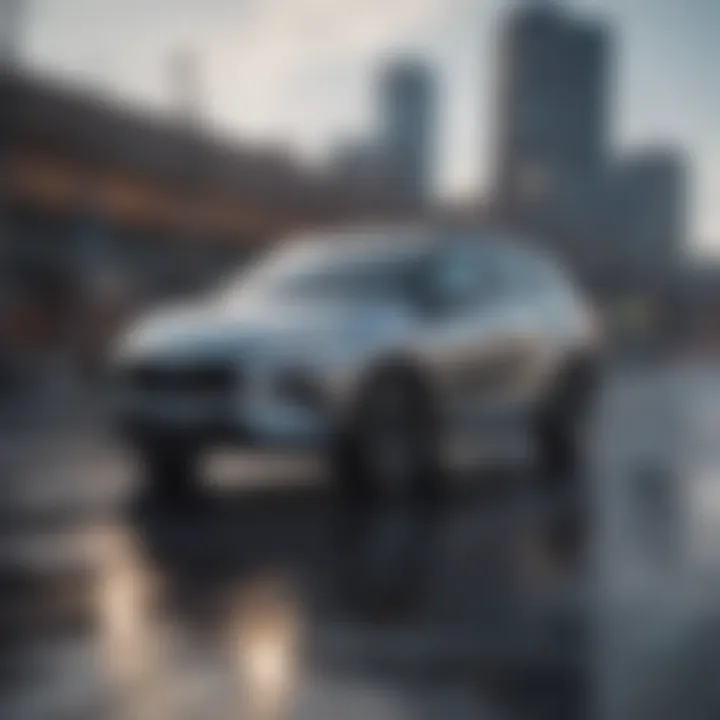
Intro
The conversation surrounding renewable energy has intensified in recent years. Various sources are analyzed for their capacity to provide sustainable solutions. Among these, hydrogen emerges as a notable candidate. The properties of hydrogen, its versatility, and the potential for implementation across many sectors offer a compelling case for its adoption. This article begins by delving into key findings regarding hydrogen as an energy source and exploring significant implications for both current applications and future developments.
Key Findings
Hydrogen stands out for several reasons. First, it is the most abundant element in the universe, making it theoretically limitless as a resource. It can be used in various forms, such as hydrogen fuel cells or burned directly for heat. Key findings include:
- Production Methods: Hydrogen can be produced through various methods including electrolysis, steam methane reforming, and even biomass gasification. Each method presents unique advantages and environmental considerations.
- Applications: In transportation, hydrogen fuel cells are being explored for efficiency and zero emissions. Industries are looking at its use in steel manufacturing and chemical production to reduce carbon footprints.
- Environmental Impacts: When generated from renewable resources, hydrogen has the potential to significantly decrease greenhouse gas emissions, thus supporting climate change mitigation efforts.
"Hydrogen is not just a fuel; it is a pathway to a sustainable future."
The significance of these findings within the scientific community cannot be understated. Researchers and institutions are focusing intensively on enhancing production techniques, exploring new applications, and understanding the long-term environmental impacts of hydrogen usage.
Implications of the Research
The implications extend beyond purely academic discussions. Hydrogen has real-world applications that are beginning to reshape industries and economies.
- Applications in Real-World Scenarios: In the automotive sector, companies like Toyota and Hyundai are investing in hydrogen-powered vehicles. Additionally, various countries are integrating hydrogen into public transportation systems.
- Impact on Future Research Directions: The push for renewable energy sources necessitates ongoing investigations into hydrogen storage technology and efficiency improvements, which could revolutionize energy systems globally.
As society transitions toward a hydrogen economy, it is crucial to assess both the opportunities and challenges that arise. This examination will provide insights that inform research, policy, and practical applications across multiple sectors.
Understanding Hydrogen
The study of hydrogen is crucial because of its potential to transform energy systems. As a lightest and simplest element, hydrogen holds the promise of sustainable energy solutions. Understanding its basic properties, reactions, and isotopes can guide future advancements in energy technology.
Hydrogen's role transcends its chemical nature; it is at the forefront of discussions surrounding renewable energy. Examining the properties of hydrogen can provide insights into their advantages over traditional fossil fuels. Moreover, its isotopes, deuterium and tritium, differ significantly in mass and behavior, affecting their practical applications, such as in fusion energy.
Hydrogen offers several benefits, which include:
- Abundant Availability: Hydrogen is the most plentiful element in the universe, making it a virtually inexhaustible resource.
- Clean Energy Production: When burned, hydrogen produces only water vapor, significantly reducing harmful emissions compared to fossil fuels.
- Versatile Applications: Hydrogen can be used across various disciplines, such as fuel cells, industrial processes, and even as a reducing agent in metallurgy.
However, certain considerations must also be accounted for in hydrogen's adoption. Safety concerns regarding its flammability and the production methods can pose challenges. Current production predominantly employs fossil fuels, which limits the environmental benefits. Thus, exploring new and greener methods of hydrogen production becomes crucial.
In sum, understanding hydrogen is fundamental for harnessing its full potential within the energy landscape, which leads to transforming our society towards a more sustainable future.
Properties of Hydrogen
Hydrogen is a unique element that has various properties making it suitable as an energy source. Its molecular structure consists of a single proton and an electron, making it the simplest and lightest element. Key properties of hydrogen include:
- State at Room Temperature: Hydrogen exists as a gas under standard conditions and has a low density, making it lighter than air.
- Reactivity: Hydrogen is highly reactive and can form compounds easily with most elements, allowing a diverse range of applications.
- Energy Content: Hydrogen has a high energy content by weight, providing an efficient means of energy storage.
These properties not only define hydrogen but also direct its role in energy systems. For example, its high energy density allows vehicles to have longer ranges when powered by hydrogen fuel cells. This capability has prompted research into hydrogen-powered vehicles.
Hydrogen Isotopes
Hydrogen has three primary isotopes: protium, deuterium, and tritium.
- Protium: This isotope has no neutrons and accounts for about 99.98% of hydrogen found in nature. It has a simple structure and is typically what is referred to in the context of hydrogen energy.
- Deuterium: Deuterium contains one neutron and is found in small quantities in natural hydrogen. This isotope has applications in nuclear reactors and other scientific processes.
- Tritium: Tritium is radioactive and has two neutrons. It is less common and is produced in nuclear reactions, often used in fusion research.
The unique properties of hydrogen isotopes open numerous potential applications, particularly in nuclear fusion technology. While tritium presents challenges due to its radioactivity, it might play a vital role in future energy systems if fusion becomes a viable energy source.
Understanding these isotopes increases the depth of knowledge about hydrogen and can help in identifying their roles in innovative energy solutions.
Hydrogen Production Methods
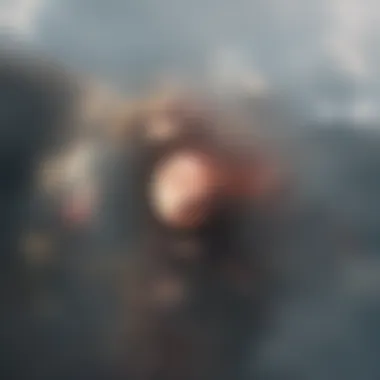
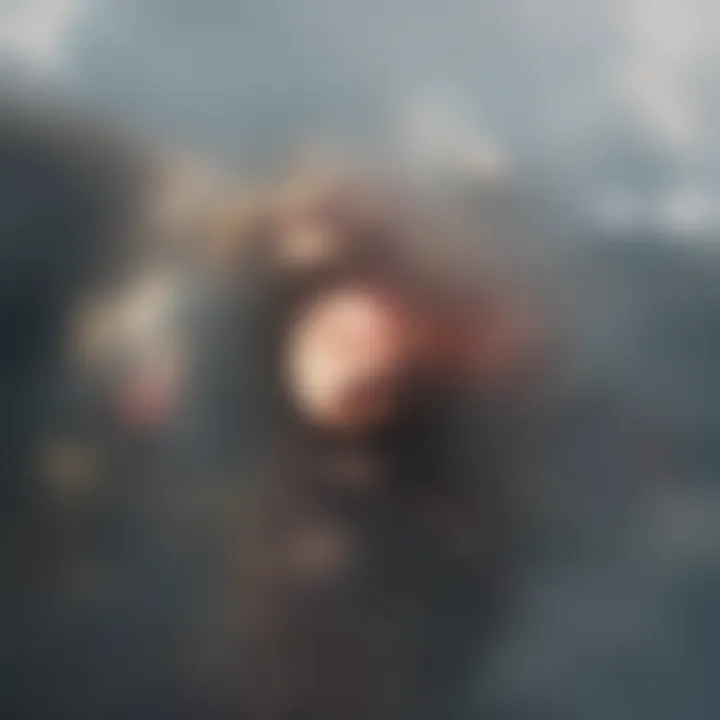
Hydrogen production methods are fundamental to understanding hydrogen as an energy source. The methods defined in this section highlight the various techniques for generating hydrogen, each with its own set of advantages and challenges. As the world moves towards a hydrogen economy, the efficiency, sustainability, and scalability of these production methods become increasingly vital. Analyzing these methods provides insights into how hydrogen can be effectively integrated into our energy systems.
Steam Methane Reforming
Steam methane reforming (SMR) is one of the most prevalent methods for hydrogen production. In this process, natural gas, primarily composed of methane, reacts with steam at high temperatures in the presence of a catalyst, producing hydrogen and carbon dioxide as by-products.
The key benefits of SMR include its established technology and high efficiency in converting natural gas to hydrogen. However, a significant drawback is the carbon emissions associated with the process, thereby raising concerns about its environmental impact. While hydrogen itself is a clean fuel, the carbon dioxide produced during SMR contributes to greenhouse gas emissions. Therefore, integrating carbon capture technologies can help mitigate these effects, but this increases operational costs.
Electrolysis
Electrolysis is considered a promising method for generating hydrogen, especially when using renewable energy sources. The process involves splitting water into hydrogen and oxygen by applying an electric current.
One of the standout benefits of electrolysis lies in its potential to produce hydrogen without carbon emissions. By utilizing solar or wind energy to provide the electricity, electrolyzers can generate green hydrogen. Despite this advantage, current challenges are high costs, particularly in the technology and energy needed. Researchers are actively working on improving the efficiency and reducing the costs of electrolyzers to make this method more commercially viable.
Biomass Gasification
Biomass gasification is another hydrogen production method that involves converting organic materials into hydrogen-rich gases through thermal processes in the absence of oxygen. This method utilizes agricultural residues, waste, and other organic materials, making it a sustainable choice for hydrogen production.
The advantages include the ability to use waste materials, thus addressing waste management issues. However, the challenges revolve around the efficiency of conversion processes and the need for advanced technologies to capture and purify the produced hydrogen. Additionally, land use and resource management are considerations that must be addressed to ensure the sustainability of biomass feedstocks.
Thermochemical Water Splitting
Thermochemical water splitting leverages high-temperature reactions, typically in a two-step process, to extract hydrogen from water. This method involves a series of chemical reactions usually driven by concentrated solar power or nuclear energy.
The potential of thermochemical water splitting lies in its capability to produce hydrogen with minimal carbon emissions, provided that the heat source is renewable. However, the complexity of the system and the need for advanced materials resistant to high temperatures and corrosive environments are significant technical challenges. Continued research and development efforts must focus on enhancing the durability and efficiency of materials used in thermochemical processes.
Overall, the understanding of hydrogen production methods is critical as society advances toward cleaner energy solutions, integrating hydrogen into various sectors.
Applications of Hydrogen
The applications of hydrogen span various sectors, demonstrating its versatility as an energy source. This section delves into how hydrogen can fulfill energy needs in innovative ways. It reveals benefits that hydrogen brings to sectors such as transportation and industry, which are critical as the world shifts towards more sustainable energy solutions. Understanding these applications is vital for evaluating hydrogen's role in the broader energy landscape.
Fuel Cells
Fuel cells are a significant application of hydrogen technology. They convert chemical energy from hydrogen directly into electricity, with water and heat as by-products. There are several types of fuel cells, each suited for different applications.
Types of Fuel Cells
Types of fuel cells include Polymer Electrolyte Membrane (PEM), Solid Oxide Fuel Cells (SOFC), and Alkaline Fuel Cells (AFC). PEM fuel cells are often regarded as efficient for transportation due to their quick start-up time and high power density. SOFCs, on the other hand, operate at much higher temperatures and are suitable for stationary applications. Each type has unique characteristics that make them beneficial choices for various strategies in sustainable energy.
However, PEM cells are commonly used in vehicles, while SOFC technology looks promising for large energy systems. The choice often depends on the specific energy requirements and environmental conditions.
Operation Mechanism
The operation mechanism of fuel cells involves an electrochemical reaction. Hydrogen molecules enter the fuel cell and split into protons and electrons across a membrane. The protons transfer through the membrane, while the electrons travel along an external circuit, creating electricity. This process is central to the effectiveness of hydrogen as a clean energy source, minimizing carbon emissions compared to combustion engines.
This mechanism showcases the unique feature of fuel cells—minimal environmental impact while generating power. However, the technology is still developing, and efficiency improvements are needed for broader adoption.
Hydrogen in Transportation
Hydrogen in transportation represents a transformative shift in how we think about energy use in vehicles. Its potential to reduce emissions and provide a clean fuel alternative makes it an attractive option for the future.
Commercial Vehicles
Commercial vehicles utilizing hydrogen fuel cells can significantly lower greenhouse gas emissions. Tesla and Nikola are among companies developing hydrogen-powered trucks. Hydrogen trucks can be refueled quickly, similar to diesel vehicles, which is a key characteristic that enhances their practicality.
Unique aspects, such as long range and the ability to carry heavy loads, distinguish hydrogen vehicles from battery electric ones. However, challenges remain, especially regarding infrastructure and the availability of hydrogen filling stations.
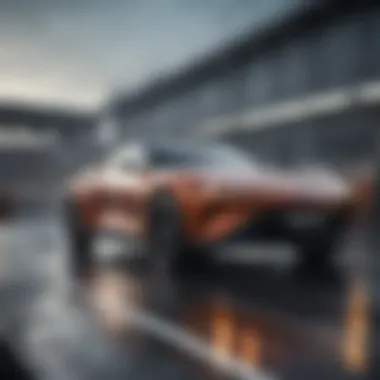
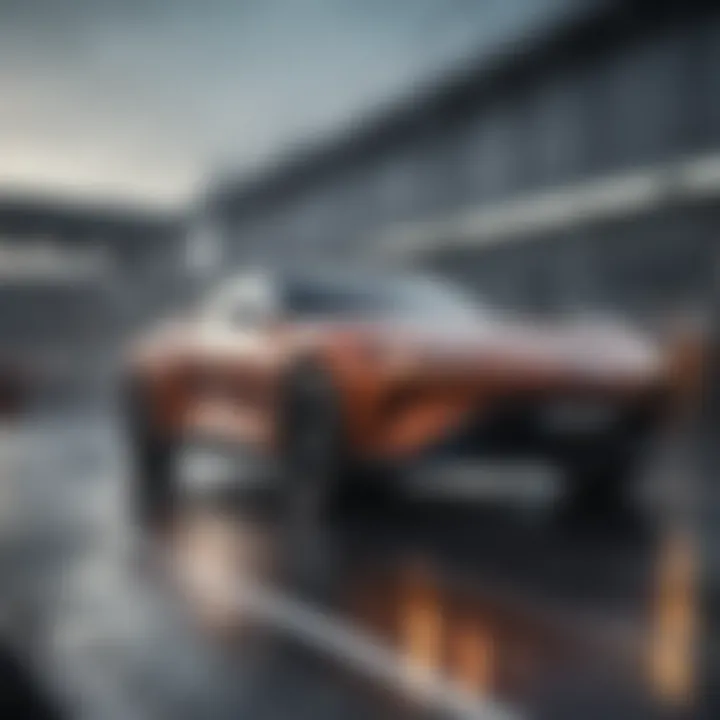
Public Transport Systems
Public transport systems could also benefit from hydrogen adoption. Buses powered by hydrogen fuel cells operate quietly and with zero emissions. This benefit not only improves air quality in cities but also aligns with urban sustainability goals.
Hydrogen buses offer unique features compared to traditional diesel buses, such as lower operating costs over time. Nevertheless, the initial investment and infrastructure requirements may present challenges to widespread implementation.
Industrial Applications
Industrial applications for hydrogen are diverse but particularly crucial in sectors that require significant energy input. Hydrogen's properties make it suitable for various processes, including steel production.
Steel Production
In steel production, hydrogen can serve as a reducing agent, replacing carbon traditionally used in the process. This shift could lead to dramatically lower carbon emissions for steel manufacturers. Hydrogen-based steelmaking processes are becoming more favored as regulatory pressures increase to reduce emissions.
This unique approach not only improves the sustainability of the industry but also showcases hydrogen's potential to enable greener manufacturing practices. Challenges include technological readiness and the need for substantial investment.
Hydrogen as a Reducing Agent
Acting as a reducing agent, hydrogen plays a pivotal role in chemical reactions that produce metal ores. Its use leads to purer metals compared to traditional methods, which still release significant amounts of CO2. This characteristic is vital for industries focused on sustainability. However, the adaptation of existing systems to incorporate hydrogen might require more time and effort as companies look for economic viability.
By examining the applications of hydrogen, we begin to see a clearer picture of how it fits into a broader strategy for renewable energy. Its potential for driving change in transportation, industry, and energy production calls for further exploration and innovation.
Environmental Impact of Hydrogen
The environmental impact of hydrogen is crucial in assessing its viability as a sustainable energy source. As the world confronts climate change and environmental degradation, hydrogen offers a potential pathway to reduce greenhouse gas emissions. However, understanding its lifecycle, water use, and resource management is essential for making informed decisions about hydrogen's role in our energy future.
Carbon Emissions and Lifecycle
Hydrogen production methods vary significantly in terms of their carbon emissions. For instance, steam methane reforming, the most common method, involves natural gas and releases considerable CO2. In contrast, methods like electrolysis when powered by renewable energy have minimal carbon footprint. It's essential to analyze not just the emissions from production but every stage in hydrogen's lifecycle.
- From Production to Use: Carbon emissions include extraction, production, transportation, and end-use. Each step can contribute to the overall carbon footprint.
- Impact of Transport: Transporting hydrogen itself can also introduce emissions, especially if pipelines or trucks use fossil fuels.
- End-of-Life Concerns: Consideration must also be given to how hydrogen systems are decommissioned and whether any waste is generated or emissions produced during this phase.
Assessing these factors leads to clearer insights into the net impact hydrogen has on global emissions.
Water Use and Resource Management
The production of hydrogen, particularly through methods like electrolysis and biomass gasification, necessitates substantial water usage, which might strain local resources. Understanding this connection illustrates the balance that must be struck between hydrogen production and water resource management.
- Electrolysis Water Demand: This method requires large quantities of purified water. Use in regions facing drought or water scarcity can present ethical and practical challenges.
- Sustainable Water Use: Strategies must be developed to ensure that water extraction for hydrogen production does not compromise local ecosystems or communities.
- Integrated Resource Management: Policymakers and researchers must work together to create frameworks that integrate water and energy production safely and sustainably. This holistic view can promote better resource management.
"The relationship between hydrogen production and water use highlights the need for sustainable practices that minimize negative impacts on local resources."
In summary, careful consideration of hydrogen's environmental impact can illuminate both its advantages and its drawbacks. By adopting a detailed approach to analyze carbon emissions and water use, it becomes possible to craft a more sustainable energy landscape.
The Role of Hydrogen in Renewable Energy Integration
The discussion of hydrogen's role in renewable energy integration is vital. Hydrogen is not just an energy carrier; it serves as a mediator between renewable energy sources like solar and wind and energy consumers. As the world increasingly seeks sustainable solutions, hydrogen presents unique benefits that align with these aspirations.
Energy Storage Solutions
One primary advantage of hydrogen lies in its ability to store energy. Energy production from renewable sources is often variable. Wind and solar power generate electricity only under certain conditions. This variability can lead to periods of excess energy production or shortages when demand is high.
Hydrogen can help mitigate this challenge through energy storage. Excess electricity from renewable sources can be used to produce hydrogen via electrolysis. This process splits water into hydrogen and oxygen using electrical energy. The hydrogen produced can be stored for long periods and converted back into electricity when necessary, for example, during times of low energy generation.
Benefits of hydrogen storage include:
- Scalability: Hydrogen systems can be scaled to meet various energy demands, from small community needs to large industrial requirements.
- Long-Term Storage: Unlike batteries, which typically have limited storage durations, hydrogen can be stored indefinitely.
- Diverse Applications: The stored hydrogen can be used not only for electricity generation but also in transportation and heating, enhancing the flexibility of energy systems.
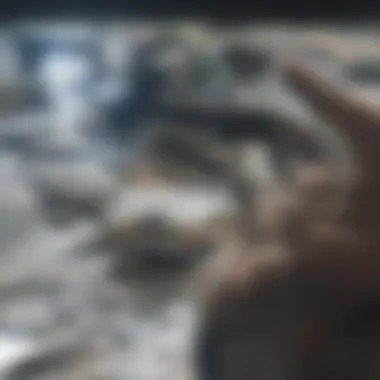
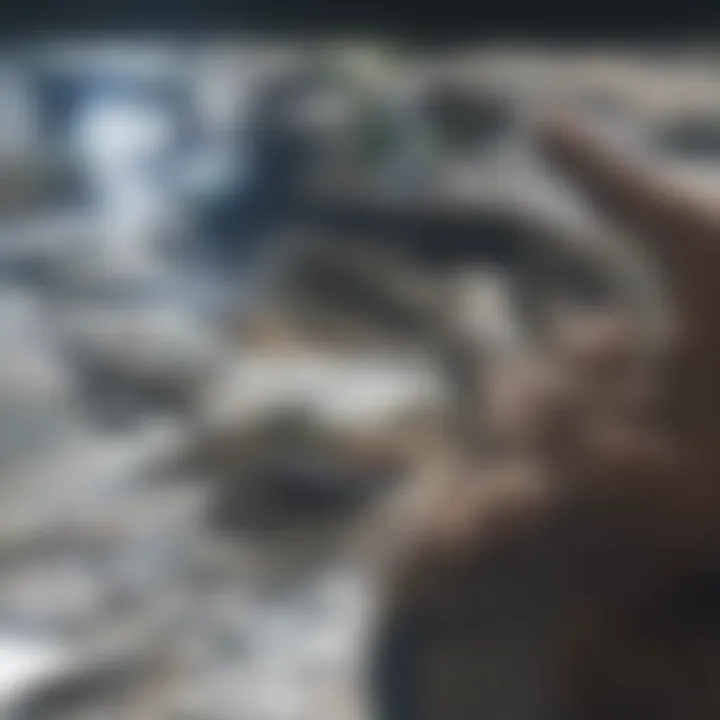
Grid Stability and Hydrogen
Hydrogen also contributes to the stability of energy grids. As renewable energy sources increase in prominence, the risk of grid instability rises. This instability can arise from the intermittent nature of renewable energy generation. Hydrogen systems can act as buffer zones, absorbing excess energy and releasing it when needed, thus maintaining a steady supply of electricity.
Moreover, the use of hydrogen fuel cells can provide a rapid response during peak demand times. By using hydrogen to generate electricity on-site, users can reduce strain on the main grid. This process allows for a more resilient energy infrastructure.
In addition, hydrogen offers the potential for resilience:
- Flexibility in Energy Sources: Hydrogen can be produced from various feedstocks, including natural gas, water, and biomass, allowing for localized energy solutions and reducing dependence on specific sources.
- Decentralization: Distributed hydrogen production can alleviate pressure on centralized energy grids, minimizing the risk of widespread outages.
By facilitating both energy storage and grid stability, hydrogen plays a critical role in seamlessly integrating renewable energy into the existing energy framework.
Challenges in Hydrogen Adoption
The adoption of hydrogen as an energy source encounters several significant challenges that must be addressed to unlock its full potential. These challenges range from the necessary infrastructure development and economic viability to public perception and awareness. Understanding these elements is crucial because overcoming these barriers is essential for transitioning to a hydrogen economy. Each of these aspects will shape how hydrogen is integrated into future energy solutions and industries.
Infrastructure Development
The infrastructure for hydrogen distribution and storage is currently underdeveloped. Unlike established energy sources such as natural gas or electricity, hydrogen requires specialized pipelines, storage tanks, and fueling stations. Developing this infrastructure demands significant investment and coordination across various sectors.
- Storage Facilities: Hydrogen is challenging to store due to its low density and small molecular size. Storage solutions must ensure safety and efficiency.
- Distribution Networks: Expanding existing gas pipelines or creating new networks poses logistical and regulatory hurdles.
- Refueling Stations: For hydrogen vehicles to thrive, a widespread network of fueling stations is essential. Presently, such facilities are sparse, limiting the growth of hydrogen-powered transportation.
These needs highlight the urgency of advancing infrastructure to facilitate broader hydrogen adoption.
Economic Viability
Hydrogen's economic feasibility is another critical concern. Even though hydrogen can offer long-term benefits, the initial costs of production, storage, and distribution can be high. Current methods such as Steam Methane Reforming, while effective, are not sustainable due to carbon emissions and dependence on fossil fuels.
- Production Costs: Methods like electrolysis are still relatively costly compared to traditional fuels.
- Investment Needs: Significant capital must be channeled into research and development, infrastructure, and market deployment to make hydrogen competitive with other energy sources.
- Market Dynamics: Fluctuations in the global energy market can impact hydrogen's attractiveness. As renewable energy costs decrease, hydrogen production from these sources will become more favorable, but careful market positioning is required.
In summary, ensuring that hydrogen can compete economically with established energy sources is vital for its successful adoption.
Public Perception and Awareness
Public perception can heavily influence the adoption of hydrogen technologies. Many individuals remain unaware of the benefits and safety of hydrogen.
- Safety Concerns: There is a general concern about the flammability and explosiveness of hydrogen. Educational initiatives must address these fears to not impede progress.
- Awareness Campaigns: Informing the public about the environmental benefits of hydrogen and its role in achieving energy transition is necessary. This can be fostered through workshops, seminars, and educational content across media platforms.
- Community Engagement: Engaging local communities in discussions about hydrogen technologies can help build trust and acceptance over time.
Future Prospects of Hydrogen Technology
The future of hydrogen technology holds great promise in addressing the challenges of sustainable energy. As the world shifts toward cleaner energy sources, hydrogen stands out as a viable alternative. It can contribute to reducing carbon emissions, enhancing energy security, and promoting economic stability. The ongoing advancements in hydrogen production methods and applications are paving the way for it to become a cornerstone of future energy systems.
Hydrogen technology excels in versatility. It can serve in diverse sectors such as transportation, heating, and industrial processes. With these roles, it can help facilitate the transition towards a low-carbon economy. Its potential in energy storage and grid management further amplifies its importance. These advantages make it necessary to explore not only the current innovations but also the policy frameworks that could support its development in the coming years.
Innovations on the Horizon
The landscape of hydrogen technology is rapidly evolving. Recent innovations are emerging in various domains, from production methods to applications. One primary area of focus is the enhancement of electrolysis systems. New catalysts are being developed to improve the efficiency and reduce the costs of hydrogen generation through water splitting. Innovations in membrane technology can also lead to significant improvements in both fuel cell performance and hydrogen storage.
Another exciting direction is the integration of hydrogen with renewable energy sources. For example, combining hydrogen production with solar or wind energy can create a system where excess energy is converted into hydrogen. This not only provides a valuable energy carrier but also buffers the intermittency of renewables.
Moreover, advancements in hydrogen transportation technologies are noteworthy. Companies are working on pipelines specifically designed for hydrogen, while improvements in liquefaction processes are making long-distance transport more feasible. This will allow hydrogen to be a globally traded commodity, similar to fossil fuels today, thus opening new economic avenues.
Hydrogen energy represents far more than mere potential; it is the key that unlocks a sustainable future.
Policy Implications and Support
The successful integration of hydrogen technology into the global energy mix requires robust policy frameworks. Governments play a crucial role in steering research and investment towards hydrogen solutions. Clear regulations, financial incentives, and streamlined permitting processes can accelerate technological adoption and commercialization.
Investment in hydrogen infrastructure is critical. Public funding can help bridge the gap for initial projects, such as hydrogen refueling stations and production facilities. Furthermore, collaboration between the public and private sectors will be essential. Public awareness campaigns will also help in shaping positive perceptions toward hydrogen as an energy source.
Another important aspect is aligning hydrogen policies with broader climate goals. By creating supportive environments for innovation, governments can facilitate the learning and scaling of hydrogen technologies. Global partnerships among nations can promote shared knowledge and resources, enhancing the collective quest for sustainable energy solutions.
In summary, the prospects for hydrogen technology are promising, driven by innovation and guided by policy support. As refining these advancements continues, the implementation of hydrogen as a mainstream energy source seems increasingly attainable.