Exploring the Fundamentals of Basic Microbiology
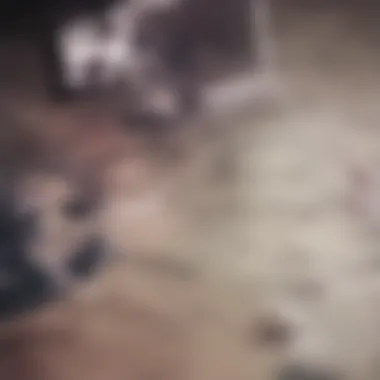
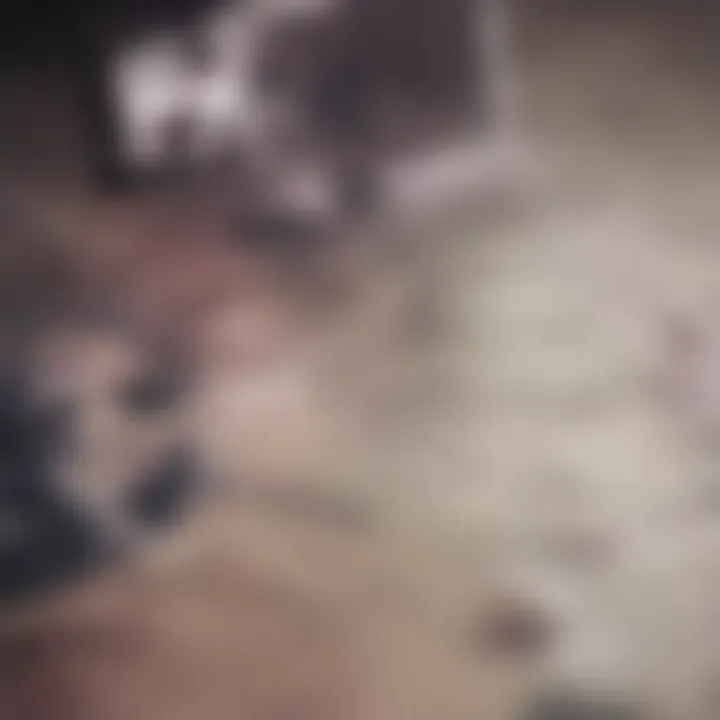
Intro
Microbiology stands as a cornerstone of biological sciences, providing an insightful lens into the world of microorganisms that inhabit virtually every environment on Earth. These tiny entities, often invisible to the naked eye, play crucial roles in health, industry, and ecosystems. Understanding their classification and significance is fundamental not just for students but also for educators, researchers, and professionals in various fields.
This article aims to outline the essential principles of basic microbiology, its methodologies, and applications. Detailed exploration of microorganisms, including bacteria, viruses, fungi, and protozoa, will be discussed, focusing on their roles within ecosystems as well as their applications in health and technology.
Because they affect everything from our health to our environment, microbiology is not merely a study of pathogens. It encapsulates all microbial life forms' interactions, proliferations, and contributions. By diving into this field, one gains a broader perspective on how life functions on a microscopic scale and how these interactions impact life at a larger scale.
Preface to Microbiology
Microbiology plays a critical role in understanding the myriad microorganisms that influence our world. From bacteria to fungi, viruses to protozoa, these tiny entities not only contribute to ecological balance but also affect health, industry, and environmental processes. This section offers insights into the importance of microbiology, and how comprehending its fundamentals elicits benefits across various fields.
Definition and Scope
Microbiology is the scientific study of microorganisms, which include bacteria, viruses, fungi, protozoa, and algae. These organisms are usually too small to be seen with the naked eye and yet have profound effects on life and the environment. The scope of microbiology extends into several domains, influencing diverse areas such as medical microbiology, environmental microbiology, and industrial microbiology.
In medicine, microbiology focuses on pathogens and their role in diseases, which is essential for diagnosis and treatment. Environmental microbiology emphasizes the role of microbes in processes like nutrient cycling and decomposition. Meanwhile, industrial microbiology applies microbial knowledge to develop products like antibiotics, enzymes, and biofuels. The broad scope of microbiology makes it a cornerstone for advancements in health, technology, and ecological sustainability.
Historical Perspective
The history of microbiology is rich and complex, beginning long before the proper understanding of microorganisms. Key advancements occurred in the 17th century with Antonie van Leeuwenhoek's discovery of bacteria through a microscope. His observations laid the groundwork for future microbiology study, although scientific exploration was limited.
The 19th century witnessed significant breakthroughs, particularly with Louis Pasteur and Robert Koch. Pasteur's work on fermentation and pasteurization demonstrated the role of microorganisms in food and disease. Koch proposed several postulates that established a connection between specific pathogens and illnesses, cementing the germ theory of disease. This theory transformed medical science and public health, saving countless lives.
As microbiology evolved, additional milestones included the discovery of antibiotics, notably by Alexander Fleming, and various techniques for culturing and studying these organisms. Understanding microbial diversity, including beneficial microorganisms, has opened new avenues in health and environmental preservation.
The historical context illustrates that microbiology is not merely a modern discipline but one deeply interwoven with human history. This foundation sets the stage for studying the various types of microorganisms, their classifications, and their complex interactions in ecosystems.
Types of Microorganisms
Understanding the different types of microorganisms is crucial for grasping the essence of microbiology. Each group of microorganisms contributes uniquely to ecosystems, human health, and industry. The knowledge of these types enhances our perspective on how life operates at a microscopic level and informs various applications in science and technology. This segment will examine the notable groups of microorganisms: bacteria, viruses, fungi, protozoa, and algae, detailing their structures, functions, and significance.
Bacteria
Structure and Function
Bacteria are single-celled organisms that exhibit a diverse range of shapes, sizes, and metabolic capabilities. Typically, they have a simple cell structure that lacks a nucleus. The cell wall, composed of peptidoglycan, provides structural support and shapes the cell. This bacterial structure allows them to survive in various environments and thrive under extreme conditions. The ease of cultivation in laboratory settings makes bacteria a popular subject in microbiological studies, as they can be rapidly grown and manipulated for research purposes. Understanding their structure is vital because it provides insights into their function and adaptability in different ecosystems, thus paving way for applications in medicine and biotechnology.
Reproduction
Bacteria primarily reproduce asexually through a process called binary fission, where a single bacterial cell divides into two identical daughter cells. This method leads to rapid population growth and is one reason bacteria can adapt swiftly to environmental changes. Their ability to exchange genetic material, such as plasmids, enhances genetic diversity, which is beneficial in evolving resistance to antibiotics. This rapid reproduction rate poses challenges in treating bacterial infections, highlighting the need for ongoing research in antimicrobial strategies.
Metabolism
Bacterial metabolism varies widely. They can be classified into anaerobic and aerobic organisms, based on oxygen requirements. Anaerobic bacteria thrive in environments devoid of oxygen, while aerobic bacteria require oxygen for growth. This metabolic versatility allows them to exploit various ecological niches. For instance, some bacteria play crucial roles in nutrient cycling and soil fertility through their metabolic processes. The knowledge of bacterial metabolism is essential for understanding their ecological roles and potential applications in waste treatment and bioremediation.
Viruses
Composition
Viruses, unlike bacteria, are acellular and consist primarily of genetic material surrounded by a protein coat. They lack the cellular structure that characterizes living organisms, relying on host cells for replication. Their composition highlights their parasitic nature, as they do not possess the machinery for independent metabolic processes. This unique composition is vital because it informs how viruses interact with host organisms and is significant in the development of antiviral therapies. The simplicity of their structure makes them intriguing subjects in both virology and biotechnology settings.
Replication Cycle
The replication cycle of viruses unfolds in several stages, including attachment, entry, replication, assembly, and release. After the virus attaches to a host cell, it injects its genetic material inside. The host's cellular machinery is then hijacked to produce new viral particles. This replication cycle is significant for understanding viral pathogenesis and developing vaccines. Knowing the details of this cycle can help researchers devise strategies to interrupt it, aiding in the fight against viral infections.
Fungi
Types of Fungi
Fungi demonstrate a remarkable variety, ranging from yeasts to mushrooms. They can be classified into four main groups: chytridiomycetes, zygomycetes, ascomycetes, and basidiomycetes. Each type plays distinct roles in the ecosystem. For example, yeasts are used extensively in food and beverage production, while some mushrooms serve as important decomposers. Understanding the various types of fungi is essential, as they offer numerous applications in industry and agriculture, demonstrating their versatility and ecological significance.
Ecological Roles
Fungi play crucial ecological roles as decomposers, breaking down dead organic material into simpler compounds, thus recycling nutrients back into the ecosystem. Mycorrhizal fungi form symbiotic relationships with plants, enhancing nutrient uptake, particularly phosphorus. Their ecological contributions underscore their importance in maintaining ecosystem health and fertility. Recognizing these roles can aid in conservation efforts and sustainable agricultural practices.
Protozoa
Classification
Protozoa are classified mainly based on their locomotion and morphological characteristics. Notable groups include amoeboids, ciliates, flagellates, and sporozoans. Each group exhibits unique attributes that influence their ecological roles. For instance, ciliates are often found in aquatic systems, playing roles in nutrient cycling. Understanding protozoan classification helps in studying their diversity and environmental significance.
Habitat
Protozoa inhabit a wide range of environments, including fresh and saltwater, soil, and as parasites in host organisms. Their adaptability to various habitats enhances their ecological importance, especially in freshwater and marine ecosystems where they serve as primary consumers. Recognizing their habitats contributes to understanding their ecological functions, including their roles in food webs and nutrient dynamics.
Algae
Photosynthesis
Algae are crucial photosynthetic organisms that contribute significantly to global primary production. They contain chlorophyll and, through photosynthesis, convert sunlight into chemical energy, producing oxygen as a byproduct. Algae's role in photosynthesis is particularly important in aquatic ecosystems, serving as the base of the food chain. Their ability to efficiently convert sunlight into energy positions them as essential contributors to both local and global ecologies.
Aquatic Ecosystems
Algae inhabit various aquatic ecosystems, from freshwater ponds to the open ocean. They support aquatic life, serve as a food source for numerous organisms, and contribute to the carbon cycle. The presence and health of algal populations can indicate the overall health of aquatic ecosystems, making their study essential for environmental monitoring and conservation efforts. Understanding the dynamics of algae helps inform approaches to managing aquatic resources and ecosystems.
Microbial Classification
Microbial classification is essential in understanding the diversity of microbial life. It provides a structured way to categorize microorganisms based on their characteristics and relationships. Knowing how microbes are classified helps researchers, students, and professionals streamline their studies and applications. This organisation aids in identifying pathogens, tracking epidemiology trends, and exploring microbial roles in various environments.
Taxonomy
Taxonomy refers to the science of naming, describing, and classifying organisms. In microbiology, taxonomy plays a pivotal role in the identification and study of microorganisms. It allows scientists to systematically group microbes according to shared traits, which can promote better understanding and communication in research and application.
Hierarchy of Classification
The hierarchy of classification consists of several levels, including domain, kingdom, phylum, class, order, family, genus, and species. This structure is advantageous because it organizes the vast diversity of microbes into manageable categories. Each level narrows down the classification, which aids in pinpointing precise characteristics.
The hierarchical system's main characteristic is its stepwise approach. Each category reflects a degree of relatedness, from broad to specific. This makes it a beneficial choice for comprehending complex relationships between different organisms.
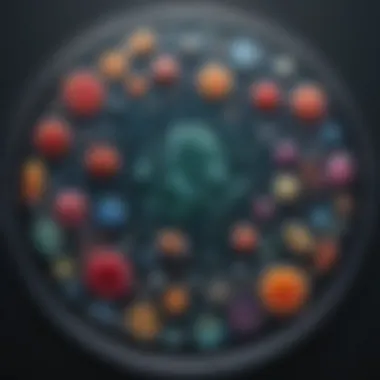
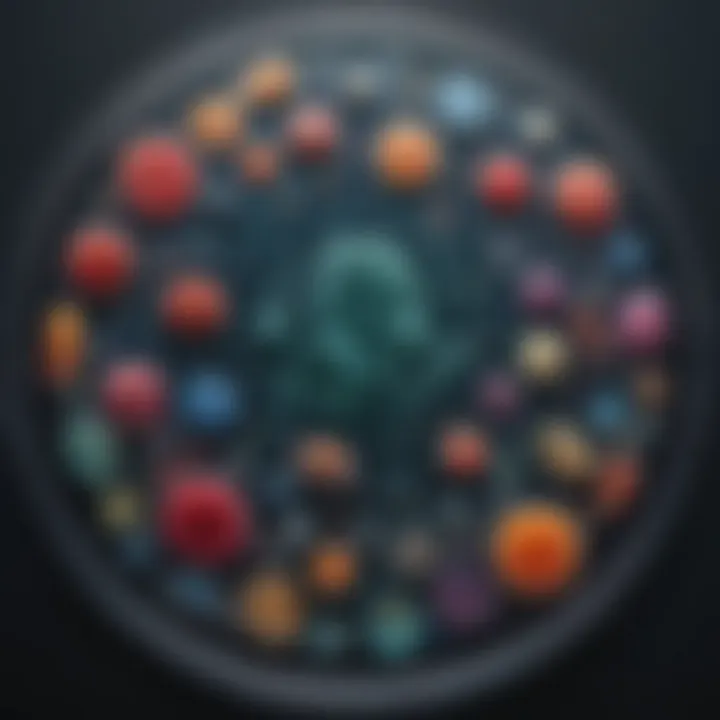
A unique feature of this hierarchy is its flexibility. For example, some organisms may change classification as new information emerges. However, the limitation lies in how rapidly changes occur in taxonomic classifications, which can create confusion in research fields.
Binomial Nomenclature
Binomial nomenclature is the formal system of naming species using two parts: the genus name and the specific epithet. This method, established by Carl Linnaeus, streamlines the identification process across languages and regions, making it a widely accepted choice in scientific literature.
The key characteristic of binomial nomenclature is precision. It eliminates ambiguity by providing a unique name to each species. This specificity facilitates better communication among researchers globally.
A unique feature is that the names are often derived from Latin or Greek roots, which adds consistency and predictability to taxonomy. However, the drawback of this system is the reliance on widespread acceptance of these names, which can vary among different scientific communities.
Phylogenetics
Phylogenetics deals with the evolutionary relationships among organisms. This field is integral to understanding how different microbes are related through common ancestry. By examining genetic data and evolutionary traits, researchers can develop a clearer picture of microbial evolution.
Evolutionary Relationships
The study of evolutionary relationships illuminates how organisms have diverged from their common ancestors over time. This understanding is beneficial for the classification and study of "
microbial diversity. "
A key feature of this approach is the use of phylogenetic trees, which visually represent these relationships. These trees help researchers identify evolutionary pathways and common traits. The trees provide insights into ecological roles and behaviors of microbes.
However, challenges exist in accurately constructing these trees. Limited genetic information can sometimes lead to misleading conclusions about relationships, affecting classification accuracy.
Molecular Techniques
Molecular techniques, such as DNA sequencing and phylogenetic analysis, are revolutionizing microbial classification. These methods offer a high level of precision and can uncover relationships that are not immediately apparent through morphological observation.
One of the key characteristics of molecular techniques is their ability to analyze genetic material directly. This approach is beneficial because it allows for classification based on actual genetic similarities and differences. It advances our ability to classify microbes that are difficult to culture or analyze by traditional methods.
A unique aspect is how molecular data can confirm or contradict previous taxonomic classifications, driving revisions to existing frameworks. However, these methods can be costly and require technical expertise, which may limit accessibility for some researchers.
Microbial Ecology
Microbial ecology is a critical component of microbiology that studies the relationships between microorganisms and their environments. It encompasses a variety of topics, including how microbes interact with one another, their ecosystems, and the overall impact they have on the Earthโs biosphere. Understanding microbial ecology helps illustrate the crucial roles microorganisms play in various processes, from nutrient cycling to disease interactions. This section will delve deeper into key aspects of microbial habitats and symbiotic relationships to demonstrate their significance.
Microbial Habitats
Microbial habitats refer to the specific environments where microorganisms thrive. These habitats can be broadly classified into two categories: terrestrial ecosystems and aquatic ecosystems. Each ecosystem supports a unique diversity of microorganisms, each adapted to their environment.
Terrestrial Ecosystems
Terrestrial ecosystems encompass all land-based environments such as forests, grasslands, and deserts. The contribution of these ecosystems to microbial ecology is profound. Microorganisms in terrestrial ecosystems play an essential role in soil fertility through organic matter decomposition, nutrient cycling, and plant growth promotion.
A key characteristic of terrestrial ecosystems is their complex structure, which includes various layers of soil and vegetation. This complexity allows for a rich diversity of microbial life. For example, mycorrhizal fungi form mutualistic relationships with plant roots, enhancing nutrient absorption.
The unique feature of terrestrial ecosystems lies in their ability to store carbon and influence global climate patterns. However, they also face threats from human activities such as deforestation and land degradation, endangering microbial habitats.
Aquatic Ecosystems
Aquatic ecosystems, which include freshwater and marine environments, are home to a significant variety of microbial species. These ecosystems contribute to global nutrient cycling, particularly in carbon and nitrogen cycles. Microorganisms such as phytoplankton form the base of the food web in aquatic systems, providing essential services.
A key characteristic of aquatic ecosystems is their dynamic nature. The nutrient availability can fluctuate greatly, influencing microbial populations and their activities. The unique feature of aquatic systems is their ability to serve as filters for pollutants, with certain microbes capable of degrading contaminants.
The advantages of studying aquatic ecosystems include understanding their role in climate regulation and nutrient management. However, they are vulnerable to pollution and climate change, which can drastically impact microbial communities.
Symbiotic Relationships
Symbiotic relationships are interactions between different species that can be mutualistic, commensal, or parasitic. Each type of relationship plays a significant role in microbial ecology by affecting the survival and productivity of microorganisms.
Mutualism
Mutualism refers to the relationship where both partners benefit. In the context of microbiology, mutualistic relationships are vital for nutrient exchange and stress resistance. For example, in the human gut, both bacteria and the host benefit from this interaction.
A key characteristic of mutualism is its ability to enhance biodiversity by promoting the growth of various microorganisms and plants. The unique feature is that it can lead to a stable ecosystem where neither party can thrive without the other. Awareness of mutualism is crucial in applications such as agriculture, where beneficial microbes can enhance crop yield and resilience.
Commensalism
Commensalism describes a relationship where one organism benefits while the other remains unaffected. In the context of microbiology, many skin bacteria have a commensal relationship with humans. They can help outcompete potential pathogens without harming the host.
The key characteristic of commensalism is its subtlety in ecosystem dynamics. The unique feature is that it demonstrates the importance of microbial presence in various environments, even when no direct benefits are exchanged. This aspect is beneficial for ecosystems, as it can contribute to maintaining balance in microbial populations.
Parasitism
Parasitism is the relationship where one organism benefits at the expense of the other. In microbial ecology, this relationship can have serious implications for hosts, often leading to diseases. Pathogenic bacteria and viruses exemplify this relationship, causing numerous infectious diseases in humans and animals.
The key characteristic of parasitism is its detrimental effects, which can range from mild to severe. The unique feature is its potential to disrupt ecosystems by negatively affecting host populations. Understanding parasitism is vital in public health and disease prevention strategies.
"Microbial ecology provides insights into the intricate interrelations of life forms, highlighting their roles in sustaining ecosystems."
Exploring microbial ecology underscores the necessity to understand microbial interactions and environments. Knowledge gained in this area informs various fields, such as agriculture, health, and environmental management, emphasizing how microorganisms shape our world.
Microbial Physiology and Genetics
Microbial physiology and genetics are crucial areas in the study of microbiology, underpinning the understanding of how microorganismsfunction and reproduce. This section explores cell structure, metabolic pathways, and genetic material, all of which are key to comprehending the behavior of these organisms. The knowledge gained from studying microbial physiology and genetics not only provides insights into the functioning of microbes but also highlights their roles in environments, health, and industry.
Cell Structure
Prokaryotic vs. Eukaryotic Cells
Prokaryotic and eukaryotic cells represent two fundamental types of cellular organization in microorganisms, which is essential for understanding their physiology. Prokaryotic cells are generally simpler and smaller in structure, lacking a nucleus. Their genetic material is found in a single circular chromosome located in a region called the nucleoid. In contrast, eukaryotic cells have a complex structure with multiple linear chromosomes housed within a defined nucleus.
This distinction is beneficial for the article as it encapsulates the core differences in cellular processes, such as metabolism and reproduction.
Key characteristics of prokaryotic cells include:
- Lack of membrane-bound organelles: This leads to simpler metabolic pathways.
- Reproduction through binary fission: This process allows for rapid population increase.
Eukaryotic cells, however, have unique features that provide advantages in complexity:
- Membrane-bound organelles: Such as mitochondria, which enables efficient energy production.
- Introns in genes: Allow for greater genetic variability through alternative splicing.
Both cell types have their strengths and weaknesses in different environments, emphasizing their adaptability to various ecological niches.
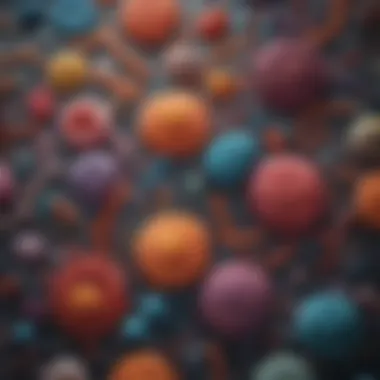
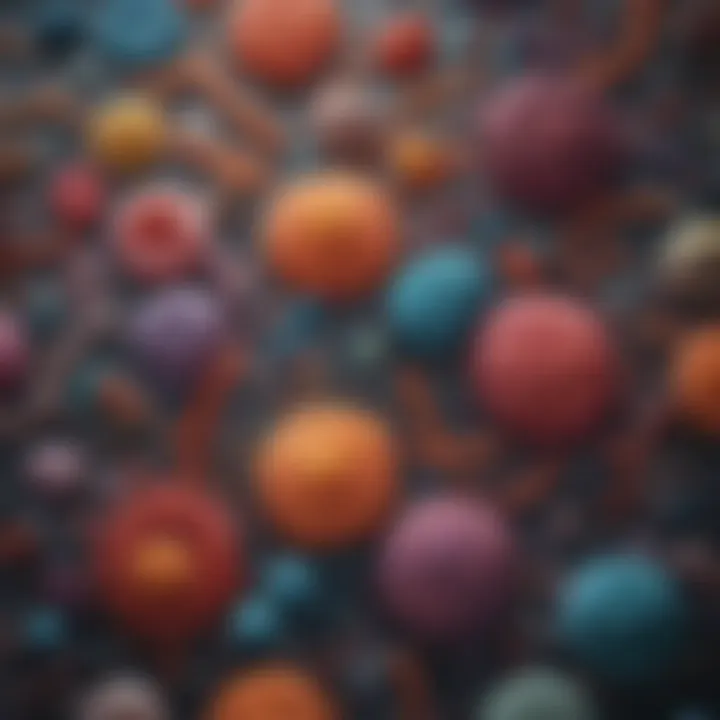
Metabolic Pathways
Anaerobic vs. Aerobic Processes
Metabolic pathways in microorganisms can be generally classified into anaerobic and aerobic processes. This distinction is critical as it influences how microbes obtain energy from their surroundings, which in turn affects ecosystem functions. Aerobic processes require oxygen and are often more efficient in energy production. In contrast, anaerobic processes do not use oxygen, relying instead on fermentation or anaerobic respiration.
This delineation adds value to the article by establishing the diverse strategies that microbes employ in various environmental contexts.
The main characteristics include:
- Aerobic processes: More ATP generation, essential for high-energy demands.
- Anaerobic processes: Functional in oxygen-limited environments, fostering unique microbial communities.
Each process has its unique features. Aerobic processes lead to complete oxidation of substrates, often yielding carbon dioxide and water, while anaerobic processes can result in byproducts such as ethanol or methane. This variation demonstrates how microbes adapt their metabolic pathways based on oxygen availability.
Genetic Material
DNA Structure
The structure of DNA is a fundamental aspect of microbial genetics. In prokaryotes, DNA exists as a single circular strand, while in eukaryotes, it is organized into multiple linear chromosomes. This structural variance has implications for gene expression and replication rates. Understanding DNA structure is important as it lays the groundwork for comprehending how genetic information is stored and transmitted.
Key features of DNA structure involved in this discussion include:
- Double helix formation: Critical for replication and repair processes.
- Base pairing: Ensures accurate transfer of genetic information during cell division.
These features underscore the reliability of DNA, making it a suitable focus for discussing genetic stability and variability in microbes.
Gene Expression
Gene expression dictates how genetic information is translated into functional proteins. The mechanisms of gene expression vary between prokaryotes and eukaryotes, reflecting their distinct cellular architectures. In prokaryotes, gene expression can occur simultaneously with transcription, leading to rapid responses to environmental changes. Eukaryotes, on the other hand, intricately regulate gene expression through various stages, including splicing and translation.
This aspect enriches the article by illustrating how microbes adapt to their environment through gene regulation.
Characteristics of gene expression relevant to the article include:
- Transcriptional control in prokaryotes: Facilitates quick responses to environmental shifts.
- Post-transcriptional modifications in eukaryotes: Allow fine-tuning of protein levels.
The diversity in gene expression mechanisms presents advantages. Rapid response to stimuli in prokaryotes is essential in fluctuating environments, while eukaryotes benefit from sophisticated regulatory networks that enable specialized functions.
Microbiological Techniques
Microbiological Techniques are fundamental to the study of microorganisms. These methods allow researchers to isolate, identify, and study various microorganisms in detail. Understanding these techniques provides crucial insight into the functional role of microbes in health, industry, and the environment. Furthermore, advancements in these techniques continuously enhance our ability to manipulate microbial activity for various applications.
Culturing Microorganisms
Culturing microorganisms is a cornerstone of microbiological techniques. It involves growing microorganisms under controlled conditions to study their characteristics and behaviors. This process can uncover various aspects of microbial life, including metabolism, reproduction, and response to environmental changes.
Media Types
Media types are specifically designed formulations that support the growth of microorganisms. They can be solid, liquid, or semi-solid and contain nutrients necessary for microbial growth. One key characteristic of media types is their composition, which varies depending on the target organism. For example, nutrient agar supports a wide range of bacteria, making it a popular choice for general culture work. The unique feature of media types is their ability to selectively encourage the growth of certain microbes while inhibiting others. This selectivity can be a significant advantage when isolating pathogens or studying specific microbial communities.
However, various media formulations may present challenges. Some microorganisms have specific growth requirements that regular media cannot satisfy. The use of specialized media can complicate the culturing process and lead to inconsistent results if not properly understood.
Incubation Conditions
Incubation conditions relate to the environment in which microorganisms are grown. These conditions include temperature, humidity, and oxygen levels, all critical for successful microbial growth. The key characteristic of incubation conditions is their ability to create an optimal environment tailored for specific microorganisms. For example, psychrophilic bacteria thrive at low temperatures, while thermophilic bacteria require high heat.
The unique aspect of incubation conditions is the way they can significantly affect microbial growth rates and behaviors. For instance, improper temperature can inhibit growth or lead to the death of sensitive organisms. It is vital for scientists to understand and control these conditions to obtain reliable and reproducible results in microbiological experiments.
Microscopy
Microscopy is another essential technique in microbiology. It allows researchers to visualize microorganisms that are not visible to the naked eye. Understanding the various types of microscopy is critical for distinguishing different microbial structures and identifying species.
Light Microscopy
Light microscopy utilizes visible light to magnify small objects. This technique is key to observing the morphology of bacteria, fungi, and other microorganisms. One of the defining characteristics of light microscopy is its accessibility and ease of use. It is a beneficial choice for educational settings and initial microbial identification due to its relatively low cost and minimal sample preparation. However, its unique feature lies in the resolving power, which limits the ability to see finer details such as the internal structures of viruses. This limitation can be a disadvantage for in-depth studies requiring higher resolution.
Electron Microscopy
Electron microscopy provides a much higher resolution than light microscopy. This technique employs electrons instead of light to view specimens, revealing detailed structures of cells and viruses. The key characteristic of electron microscopy is its capacity to visualize surfaces and biological processes at the molecular level, making it a crucial tool for advanced microbiological research. Its unique feature is the detailed imaging capability, allowing researchers to examine ultrastructures. However, it carries high costs and requires complex sample preparation, which can be your disadvantage for routine laboratory work.
Molecular Methods
Molecular methods have transformed microbiology, enabling researchers to study microbial genetics and physiology at the molecular level. These techniques are vital for understanding microbial diversity, evolution, and interactions.
PCR Techniques
Polymerase Chain Reaction (PCR) techniques amplify specific segments of DNA, making them essential for identifying microorganisms. The main characteristic of PCR is its sensitivity and specificity, allowing for the detection of even minute amounts of genetic material. This makes PCR a beneficial choice in diagnostic labs for identifying pathogens quickly and accurately. One unique aspect of PCR techniques is their speed; results can be obtained within hours. However, PCR requires precise controls to avoid contamination and false positives.
Sequencing
Sequencing determines the exact order of nucleotides in a DNA molecule, providing crucial information about gene structure and function. A primary characteristic of sequencing is its potential to reveal the genetic makeup of organisms, which can inform studies on evolutionary relationships and functional genomics. This technique is beneficial for microbiologists who seek deeper insights into microbial metabolism and pathogenicity. Its distinct feature is the broad scope of applicationsโfrom understanding microbial communities to developing novel antibiotics. However, sequencing can be expensive and requires specialized equipment and expertise, limiting its routine use in some laboratories.
Microbes and Human Health
Microbes have a profound impact on human health. Understanding how these microorganisms interact with the human body is essential for multiple fields, including medicine and public health. The role of microbes is not only about causing diseases but also about maintaining a healthy state within individuals.
Pathogenic Microorganisms
Types of Pathogens
Pathogens are organisms that can cause disease in hosts. The main types of pathogens include bacteria, viruses, fungi, and protozoa. Each type is characterized by different biological structures and mechanisms of disease.
- Bacteria: These are single-celled organisms that can reproduce independently. Some bacteria are harmful and can lead to infections, while others are beneficial and essential for processes like digestion.
- Viruses: Unlike bacteria, viruses cannot reproduce without a host. They invade host cells and can compromise immune function, leading to various diseases.
- Fungi: Some fungi can cause infections, particularly in immunocompromised individuals. However, many fungi are harmless and even useful in medicine and food production.
- Protozoa: These are more complex single-celled organisms. Some protozoa are associated with diseases like malaria.
A key characteristic of pathogens is their ability to adapt and survive in a variety of environments, making them both a concern for health care and a focal point in understanding disease spread.
Infectious Diseases
Infectious diseases are illnesses caused by pathogens entering the body. Common examples include influenza, tuberculosis, and COVID-19. Each of these diseases emphasizes the dynamic interplay between pathogens and human health.
- Consequences: Infectious diseases lead to significant morbidity and mortality worldwide.
- Public Health: Understanding infectious diseases helps inform public health policies and response strategies.
A unique feature of infectious diseases is their ability to spread from person to person, which underscores the importance of preventive measures such as vaccination and hygiene practices. By studying infectious diseases, researchers can develop better diagnostics, treatments, and preventive strategies.
Microbiomes
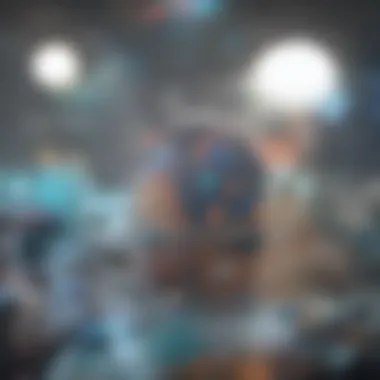
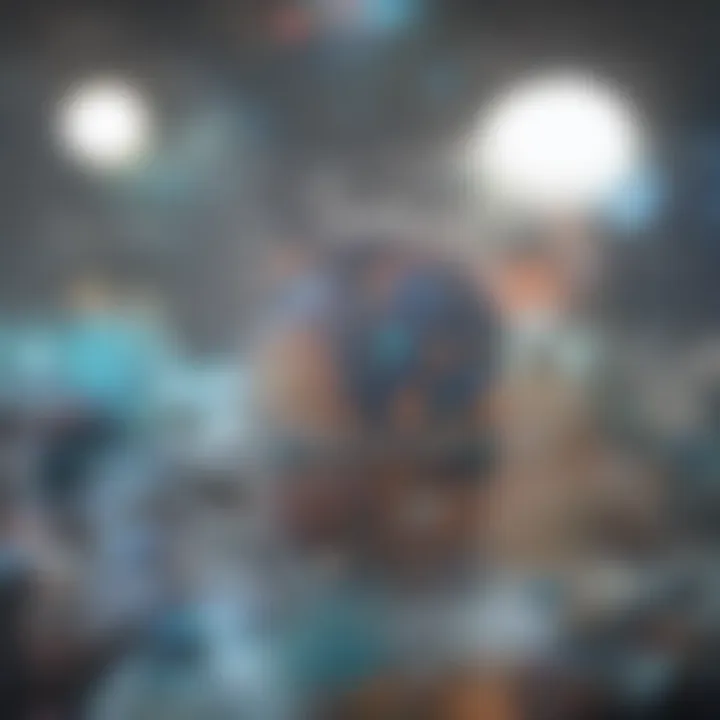
Human Microbiome
The human microbiome represents the collection of all microorganisms living in association with the human body. This complex community influences various aspects of health, including digestion, metabolism, and immune function.
The diversity of the human microbiome can vary significantly from person to person. Its composition is shaped by factors such as genetics, diet, and environment. Research into the human microbiome reveals insights into how these microorganisms contribute to health and disease.
Health Implications
The study of health implications related to the human microbiome is crucial for developing new therapeutic strategies. An imbalance in microbiota can lead to conditions like obesity, diabetes, and inflammatory bowel disease.
- Therapeutics: Understanding these implications provides avenues for treatments, such as probiotics and dietary changes.
- Research: Ongoing studies highlight the potential of microbiome modulation in improving human health.
Applications of Microbiology
Microbiology plays a crucial role in various industries and environmental processes. This application of microbiology is not merely academic; it has wide-reaching implications for health, industry, and the environment. Understanding how microbes function and their capabilities aids in leveraging them effectively for practical uses. The significance of applications of microbiology is emphasized across multiple domains, showcasing microbial contributions to technological advancements and ecological sustainability.
Industrial Microbiology
Biotechnology
Biotechnology focuses on utilizing living organisms to develop products or processes beneficial to humans. This field is a vital part of microbiology that contributes to many sectors, including medicine, agriculture, and environmental protection. The key characteristic of biotechnology is its ability to manipulate microorganisms at the genetic level, leading to innovations in producing insulin, antibiotics, and vaccines.
The reason biotechnology is often preferred in this article stems from its potential to efficiently address health and environmental challenges. In particular, genetically modified microorganisms can produce high yields of desired compounds, reducing the need for extensive resources and minimizing waste. However, it is essential to also consider the ethical implications and possible ecological impacts of such practices. The unique feature of biotechnology lies in synthetic biology, which enables designing and constructing new biological parts. This approach can accelerate discoveries but also presents challenges related to biosafety and regulatory policies.
Fermentation Processes
Fermentation processes are vital in the production of various food products, biofuels, and pharmaceuticals. This method involves the metabolic activity of microorganisms to break down substrates, resulting in energy production and by-products such as alcohol and organic acids. The noteworthy aspect of fermentation processes is their ability to occur under anaerobic conditions, which can be advantageous in specific applications.
These processes are beneficial due to their sustainability and efficiency, making them a popular choice in this article. They contribute to developing alternative energy sources, such as bioethanol, which can help reduce reliance on fossil fuels. However, the unique feature of fermentation is its sensitivity to environmental conditions, requiring careful monitoring to maintain optimal performance. The potential drawbacks include the time-consuming nature of fermentation and the possibility of contamination, which may lead to lower yields and undesirable products.
Environmental Microbiology
Bioremediation
Bioremediation refers to the use of microorganisms to remove pollutants from the environment. This process is essential for addressing contamination in soil and water, making it a significant aspect of environmental microbiology. The key characteristic of bioremediation is its natural approach, allowing microbes to transform toxic substances into harmless compounds.
Bioremediation is advantageous because it usually requires less energy than traditional cleanup methods, making it a cost-effective option for managing pollution. The unique feature of this process is its adaptability; specific microbial strains can be selected or engineered for particular contaminants, ensuring effective degradation. However, the challenges of bioremediation include the time required for microorganisms to affect significant changes and the potential unpredictability of microbial behavior in varying environments.
Waste Management
Waste management integrates various techniques to handle waste disposal efficiently, and microbiology contributes significantly through the breakdown of organic matter. In this regard, microbiology ensures optimal decomposition and recycling of resources. The critical aspect of waste management is its role in sustainability, as efficient waste treatment can lead to reduced pollution and resource recovery.
Waste management is a beneficial choice for this article because it encompasses both health and environmental considerations. Microbial processes can mitigate the impact of waste digestion, leading to by-products such as compost and biogas, which can be harnessed for energy. A unique feature of this field is the use of anaerobic digesters that utilize microbes for converting waste into renewable energy. Despite its advantages, the field faces challenges, including the infrastructure requirements for effective microbial management assays and potential public resistance to using treated waste materials.
Future Directions in Microbiology
Microbiology is a rapidly evolving field, constantly influenced by advancements in technology and research methodologies. The exploration of future directions in microbiology highlights not only the potential for innovation but also the implications for health, industry, and environmental sustainability. Understanding emerging technologies and current research frontiers can provide valuable insights into how microbial sciences will shape our future.
Emerging Technologies
CRISPR Applications
CRISPR technology is one of the most significant innovations in the field of molecular biology. It stands out for its capability to edit genes with remarkable precision. This makes CRISPR a key tool in studies related to microbial genetics and biotechnology. The primary characteristic of CRISPR is its ability to target and modify specific DNA sequences, allowing researchers to investigate gene functions and potentially correct genetic disorders.
One unique feature of CRISPR applications is their flexibility across various organisms, including bacteria, plants, and animals. This versatility allows for wide-ranging applications from agriculture to medicine.
In the realm of microbiology, CRISPR technology can be used for creating genetically modified organisms that might be more resilient to diseases or environmental stresses. However, it is not without challenges, as ethical concerns and potential off-target effects can raise questions about its implementation in research and industry.
Synthetic Biology
Synthetic biology integrates principles from biology and engineering to design and construct new biological parts. This field offers promising avenues for addressing complex problems in health, energy, and the environment. The main characteristic of synthetic biology is its focus on building new biological systems that can perform specific functions, such as producing biofuels or therapeutic compounds.
A key feature of synthetic biology is its interdisciplinary approach. It combines genomics, biotechnology, and computational science to innovate solutions for pressing global challenges. The potential benefits include sustainable production methods and creation of tailored microorganisms for specific applications.
However, synthetic biology also faces hurdles. Concerns about biosecurity and the ethical implications of creating life forms can hamper research and commercial adoption. Understanding these aspects is crucial as the field progresses.
Research Frontiers
Antimicrobials
Antimicrobials are essential for combating infectious diseases caused by bacteria, viruses, fungi, and parasites. Research in this area focuses on developing new antimicrobial agents and understanding how microbes resist treatment. The critical feature of antimicrobials is their ability to inhibit the growth of pathogens, which is vital for public health.
Antimicrobials are particularly relevant in the context of rising antibiotic resistance, which poses a significant threat to global health. Innovative approaches, such as novel compounds or alternative therapies, can lead to effective treatments. However, challenges include the need to monitor resistance patterns and ensure responsible use to preserve the effectiveness of existing antibiotics.
Vaccine Development
Vaccine development is crucial in preventing the spread of infectious diseases. Recent advancements in technology, especially mRNA vaccine platforms, have revolutionized how vaccines are developed and deployed. The standout characteristic of modern vaccine development is its speed and adaptability in response to emerging infectious threats.
The process aims to provide immunity without causing disease, making it a cornerstone of public health strategies. A unique benefit of recent methodologies is their ability to be rapidly modified in light of new viral strains, enabling quicker responses to outbreaks.
Nonetheless, disparities in vaccine access and vaccine hesitancy present significant challenges. Addressing these issues is imperative to maximize the potential benefits of vaccination in controlling infectious diseases.
The future of microbiology is significantly intertwined with technological advancements and research breakthroughs. By understanding these emerging fields, stakeholders can better navigate the challenges and opportunities ahead.
Culmination
Understanding the fundamentals of microbiology is critical for various fields. This discipline offers insights into the complex relationships between microorganisms and their environments, including human health. By studying key elements such as microbial classification and ecology, we can appreciate their roles in ecosystems and industries.
One significant element of microbiology is the ability to identify and manipulate different microbial species. This ability opens avenues in biotechnology and medicine. For instance, the development of vaccines relies on our comprehension of how pathogens interact with hosts. Additionally, the utilization of microorganisms in bioremediation highlights their potential in addressing environmental challenges.
Moreover, the relevance of monitoring and researching microbial behavior cannot be overstated. As new microbes emerge, it is essential to stay informed about their characteristics and implications for public health and safety. Hence, a structured approach in studying microbiology leads to improved outcomes and solutions.
"Microbiology bridges gaps between research and application, shaping the future of science and industry."
In summary, microbiology is not solely about microorganisms; it encompasses their vast implications on life, health, and the environment, making it imperative for continued exploration in this field.
Summary of Key Points
- Microbiology provides essential knowledge about microorganisms and their ecological roles.
- Understanding microbial classification aids in identifying and using species effectively.
- The interplay between microbes and human health has significant implications for developing treatments and vaccines.
- Research in microbiology is crucial for addressing environmental issues via techniques like bioremediation.
Importance of Microbiology
Microbiology plays a pivotal role in both science and application. Its importance can be categorized in several ways:
- Health: Microbiology informs our understanding of pathogens, leading to better health solutions and preventive measures.
- Industry: Many industries, including agriculture and pharmaceuticals, depend on microbial processes for production and innovation.
- Environment: Microbes are essential in nutrient cycling and waste decomposition, thus maintaining ecological balance.
Overall, the fundamentals of microbiology are integral to advancing knowledge and practices that can enhance life overall.