Exploring Hydrogenolysis: Mechanisms and Applications
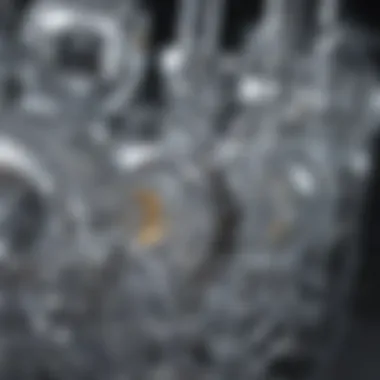
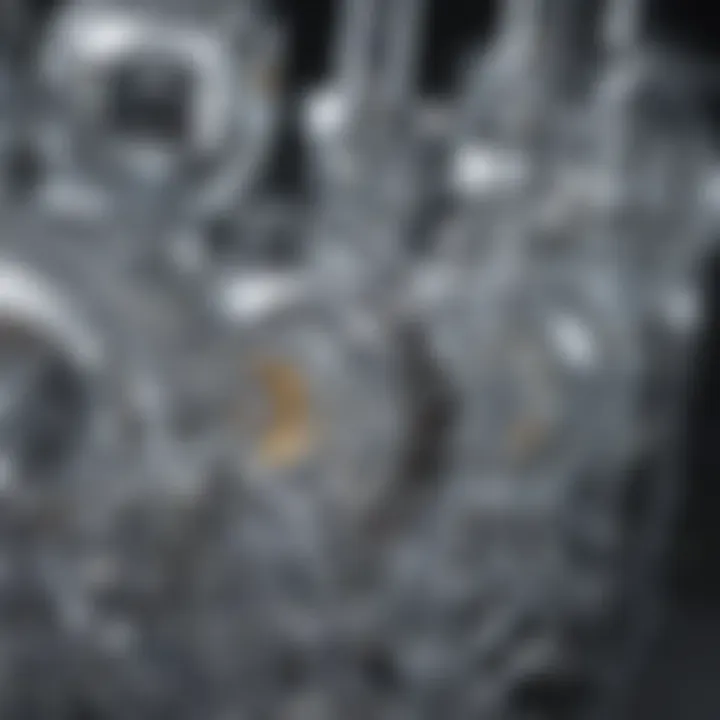
Intro
Hydrogenolysis is an essential reaction within organic chemistry, involved in breaking both carbon-to-carbon and carbon-to-heteroatom bonds in the presence of hydrogen. Understanding hydrogenolysis is critical for advancements in various fields, especially in organic synthesis and the petrochemical industry. This reaction is not just a theoretical concept; it has tangible applications that influence sustainable chemistry and environmental practices.
In this article, we will explore the intricate mechanisms of hydrogenolysis, highlighting various catalysts that facilitate the reaction. We will also discuss how different reaction conditions, such as temperature and pressure, play a vital role in its efficiency. Furthermore, we will investigate recent advancements in catalytic technologies and consider the future potential of hydrogenolysis in advancing sustainable practices within the chemical industry.
By providing a detailed analysis of the implications of this reaction and its integration with green technologies, we aim to offer valuable insights for researchers, practitioners, and anyone interested in the future of chemical processes. Ultimately, this discussion aims to illuminate the significance of hydrogenolysis in both current and future innovations in chemistry.
Prelude to Hydrogenolysis
Hydrogenolysis is fundamental in the field of organic chemistry, and its significance cannot be underestimated. It serves as both a reaction pathway and a tool for transforming various chemical structures. Through hydrogenolysis, chemists can effectively break carbon-to-carbon and carbon-to-heteroatom bonds in the presence of hydrogen. This capability makes it crucial for synthesizing a wide range of products, particularly in organic synthesis and the petrochemical industry. The article aims to unpack this intricate process, elucidating the various mechanisms, applications, and future prospects associated with hydrogenolysis.
Understanding hydrogenolysis is not just an academic pastime. Its applications extend to practical sectors, such as the production of fuels, chemical intermediates, and the conversion of biomass into more valuable chemicals. By knowing the mechanisms behind this reaction, professionals can optimize processes and develop more effective catalysts. The implications for sustainability and environmental impact further accentuate the relevance of this topic. Hence, a comprehensive analysis of hydrogenolysis stands to benefit researchers, students, and industry practitioners alike.
Definition of Hydrogenolysis
Hydrogenolysis refers to a reaction that cleaves chemical bonds using hydrogen, often facilitated by catalysts. The term itself combines the words "hydrogen" and "lysis," which signifies breaking down. During hydrogenolysis, hydrogen molecules react with a substrate, causing the bonds to break and resulting in the formation of smaller molecules. This reaction is particularly significant for converting larger, more complex molecules into simpler structures.
The underlying mechanism typically involves the heterolytic cleavage of bonds, where a nucleophile and an electrophile interact, leading to bond breaking. Given its wide array of applications, hydrogenolysis is a reaction of great importance in both industrial and academic settings. Understanding its nuances can lead to advancements in chemical synthesis methodologies.
Historical Context
The historical development of hydrogenolysis is intertwined with the evolution of catalysis as a field. Initially recognized in the late 19th century, researchers began observing that hydrogen could facilitate bond cleavage in various organic compounds. Over the decades, several landmark studies have set the foundation for this reaction, leading to significant breakthroughs in the petrochemical and organic synthesis arenas.
Key milestones include the introduction of metal catalysts, which greatly improved reaction efficiency and selectivity. For instance, the work done on nickel and palladium in the mid-20th century showcased their roles in enhancing hydrogenolysis processes. These advancements paved the way for the adoption of hydrogenolysis in industrial applications, ultimately becoming a critical tool in modern chemistry.
From these early beginnings, the study of hydrogenolysis has only expanded, with ongoing research focusing on optimizing catalytic methods and expanding applications. This context frames the discussion that follows in the article, setting the stage for an in-depth exploration of mechanisms, applications, and the future directions of hydrogenolysis.
Mechanisms of Hydrogenolysis
Understanding the mechanisms of hydrogenolysis is essential for grasping how this reaction operates in chemical processes. The ability to break carbon-to-carbon and carbon-to-heteroatom bonds with the aid of hydrogen has significant implications for both organic synthesis and the petrochemical industry. This section will detail the important aspects of hydrogenolysis mechanisms, emphasizing their relevance to various applications.
Catalytic Hydrogenolysis
Catalytic hydrogenolysis is a widely studied area as it often leads to enhanced reaction rates and improved selectivity. This mechanism involves using a catalyst to assist in breaking chemical bonds. Catalysts can lower the energy barriers for reactions, making it possible to achieve desired products while bypassing less favorable pathways.
In catalytic hydrogenolysis, palladium, platinum, and nickel are commonly employed catalysts. Their unique surface properties enable them to facilitate the adsorption of molecules, which is crucial in lowering activation energy.
The efficiency of catalytic hydrogenolysis heavily depends on the type of catalyst used. The choice of metal, for example, significantly influences both reaction rate and product distribution.
Non-Catalytic Hydrogenolysis
In some cases, hydrogenolysis can occur without the use of catalysts. This non-catalytic hydrogenolysis is typically less common due to higher energy requirements and lower reaction rates. However, it remains an interesting field of study. Factors such as temperature and pressure play a crucial role in this mechanism. Generally, higher temperatures can provide the necessary energy to break bonds directly. Despite some advantages, non-catalytic methods may lead to undesirable by-products or side reactions.
Reaction Pathways
The reaction pathways in hydrogenolysis can be complex but understanding them is vital. There are often multiple pathways that can lead to different products. The sequence of steps in these pathways varies depending on the conditions under which hydrogenolysis takes place.
For instance, there are several intermediate stages, including the adsorption of reactants onto the catalyst surface and subsequent cleavage of bonds. Length and structure of the carbon chain, as well as the presence of functional groups, can all influence which pathway is taken.
In summary, the mechanisms governing hydrogenolysis—whether catalytic or non-catalytic—are central to effectively utilizing this reaction in industrial applications and academic research. In understanding how reaction pathways develop, researchers and practitioners can better predict outcomes and craft more efficient and cost-effective processes.
Catalysts in Hydrogenolysis
Catalysts play a vital role in the efficiency and selectivity of hydrogenolysis reactions. Their presence allows the reactions to proceed at lower temperatures and pressures while increasing the rate significantly. The choice of catalyst can affect not only the yield of desired products but also the formation of unwanted by-products. Thus, understanding the types of catalysts, support materials, and recent innovations in catalytic methods is essential for optimizing hydrogenolysis processes.
Types of Catalysts
In hydrogenolysis, various types of catalysts can be used, each with its unique characteristics and functionalities. The most prominent categories include:
- Metal Catalysts: Noble metals such as palladium, platinum, and ruthenium often exhibit high activity for hydrogenolysis. These metals can facilitate bond cleavage effectively due to their electronic properties.
- Non-Metal Catalysts: Transition metal carbides and nitrides also show potential as catalysts in hydrogenolysis. They can provide similar pathways for activating hydrogen and breaking chemical bonds.
- Bimetallic Catalysts: These consist of two different metals and can lead to enhanced catalytic performance. The synergistic effects of the metals can lead to improved selectivity and activity.
The choice of catalyst will depend on the specific substrates and reaction conditions, emphasizing the need for tailor-made solutions in various applications.
Support Materials for Catalysts
The performance of catalysts in hydrogenolysis is greatly influenced by the support materials that carry them. Common support materials include:
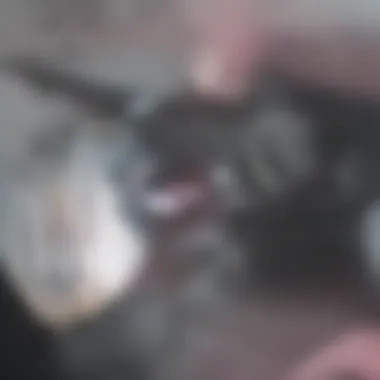
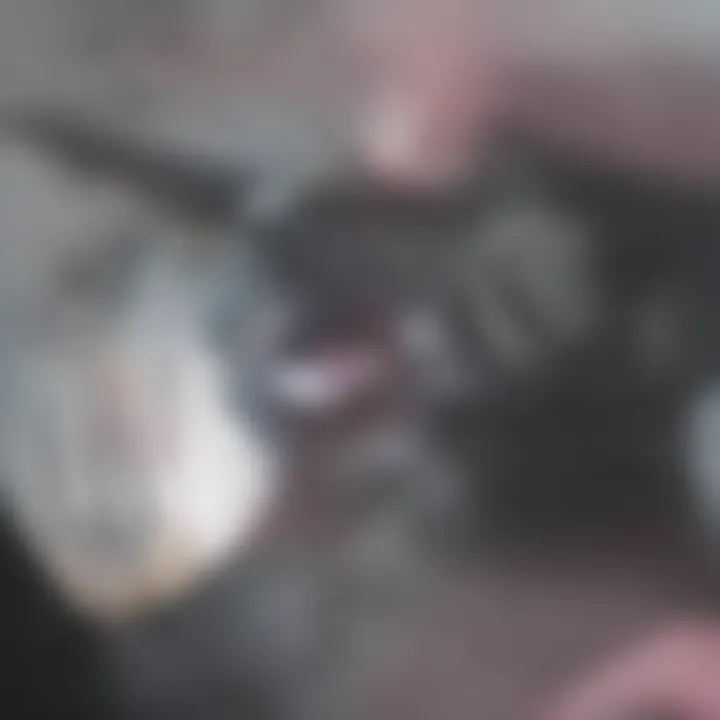
- Activated Carbon: This type of support offers a large surface area and excellent dispersion properties for metal catalysts, making it a popular choice.
- Silica and Alumina: These materials provide mechanical strength and stability, and they can affect the activity and selectivity of supported catalysts.
- Zeolites: These microporous materials can selectively absorb reactants and products, contributing to the optimal performance of catalysts.
The interaction between the catalyst and the support can significantly enhance or diminish catalytic activities, thus careful consideration of support materials is paramount in catalyst design.
Recent Advances in Catalytic Methods
Recent progress in catalytic science has led to innovative approaches in hydrogenolysis. Some notable trends include:
- Nano-Catalysts: The use of nanoparticles has been shown to enhance catalytic performance by providing a larger active surface area and improving mass transfer.
- Heterogeneous Catalysis: This method allows for easier separation of the catalyst from the reaction mixture, facilitating better recovery and reuse of catalysts.
- Computational Catalysis: Employing computational methods helps in predicting the activity and selectivity of new catalyst systems, leading to more informed catalyst design.
These advancements are crucial for improving the sustainability and efficiency of hydrogenolysis processes, paving the way for future industrial applications.
Recent developments in catalytic methods have the potential to revolutionize hydrogenolysis, enhancing its role in green chemistry.
In summary, the landscape of catalysts in hydrogenolysis involves a complex interplay between catalyst types, support materials, and innovative methods. Each element contributes significantly to the reaction's efficiency and viability in various applications.
Applications of Hydrogenolysis
Hydrogenolysis serves a crucial role in several industrial and research contexts. Its unique ability to selectively cleave carbon bonds makes it a powerful tool across various fields, especially organic synthesis and petrochemicals. The process provides not only efficiency but also selectivity in producing valuable chemical compounds. Consequently, understanding the applications of hydrogenolysis is fundamental in maximizing its potential benefits in contemporary chemistry.
Organic Synthesis
The realm of organic synthesis is where hydrogenolysis showcases its significance most strikingly. This reaction plays an essential part in the production of fine chemicals and pharmaceuticals.
For instance, many medicinal compounds require the precise modification of functional groups. Hydrogenolysis facilitates these transformations by allowing chemists to cleave specific bonds without affecting the overall integrity of the molecule.
Some of the key benefits in organic synthesis include:
- Targeted transformations: Enables selective bond cleavage.
- Higher yields: Often results in fewer side products compared to other methods.
- Versatile applications: Applicable in various chemical reactions like deoxygenation and demethylation.
Additionally, the integration of hydrogenolysis in synthetic pathways aids in the development of more sustainable processes. Traditional methods may rely on harsh conditions or toxic reagents. In contrast, hydrogenolysis typically operates under milder conditions, reducing environmental impact. This shift aligns with the principles of green chemistry, which emphasize efficiency and waste reduction.
Petrochemical Industry
Hydrogenolysis plays a vital role in the petrochemical industry, particularly in upgrading crude oil and refining processes. By converting heavier fractions of crude oil into lighter, more valuable products, hydrogenolysis supports the industry's need for efficiency and sustainability.
In this sector, notable applications include:
- Conversion of heavy hydrocarbons: Facilitates the breakdown of larger molecules into usable fuel and chemical feedstocks.
- Hydrotreating: A process that removes sulfur, nitrogen, and other impurities from petroleum products, enhancing product quality.
These processes are instrumental in addressing the growing demand for clean fuels. By improving the yield of valuable petrochemical resources while reducing impurities, hydrogenolysis contributes significantly to the overall efficiency of the petrochemical industry.
Biomass Conversion
In recent years, hydrogenolysis has gained attention in the field of biomass conversion. This application is crucial in moving towards a more sustainable future. Biobased feedstocks, such as plant materials, are rich in polysaccharides that can be converted into valuable chemicals and fuels through hydrogenolysis.
Some key points regarding biomass conversion include:
- Production of renewable fuels: Hydrogenolysis aids in the transformation of lignocellulosic biomass into liquid fuels, providing an alternative to fossil fuels.
- Chemical building blocks: It allows for the conversion of biomass into various chemicals that can serve as raw materials for further industrial applications.
Through these processes, hydrogenolysis not only aids in creating renewable energy sources but also reduces dependency on non-renewable resources. It plays a key role in addressing environmental challenges, promoting sustainable practices in chemical production.
Factors Influencing Hydrogenolysis
Hydrogenolysis is sensitive to various influencing factors that can significantly affect its efficiency and product distribution. Understanding these factors is crucial for optimizing the reaction conditions and achieving desirable outcomes, especially in industrial applications. Researchers must consider the interplay between temperature, pressure, and solvent choice when designing hydrogenolysis processes. Each of these elements plays a vital role in determining the reaction kinetics, selectivity, and overall yield, which ultimately impacts the success of hydrogenolysis in both academic and industrial contexts.
Temperature Effect
The temperature at which hydrogenolysis is conducted can greatly influence the reaction rate and product formation. As a rule, increasing the temperature tends to enhance reaction kinetics by providing the necessary energy for molecular collisions. This can lead to higher rates of bond cleavage, resulting in increased formation of hydrogenolysis products. However, there are critical considerations when adjusting temperature:
- Reaction Rate: Higher temperatures generally result in faster reactions.
- Selectivity Issues: Excessive heat may lead to side reactions, producing unwanted by-products.
- Catalyst Stability: Some catalysts might degrade at elevated temperatures, reducing their effectiveness.
Incorporating precise temperature control is essential for optimizing yields and ensuring the desired selectivity of products.
Pressure Considerations
Pressure is another significant factor in hydrogenolysis, particularly because the presence of hydrogen gas under elevated pressure can significantly alter reaction dynamics. Application of high pressure may shift equilibria and improve hydrogen solubility in the reaction mixture. Key aspects of pressure influence include:
- Increased reactant concentration: More hydrogen in the system can promote effective hydrogenation and reduce the likelihood of undesired reactions.
- Effect on Rate: Higher pressure often leads to accelerated reaction rates by favoring the dissociation of reactants.
- Safety concerns: High pressures necessitate strict controls and equipment that can withstand stress, adding to operational complexity.
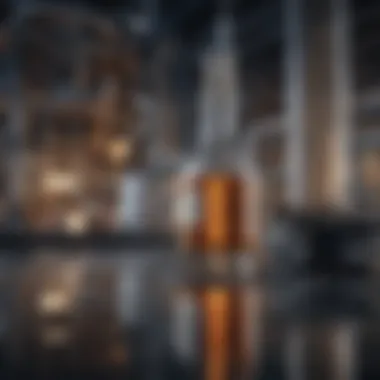
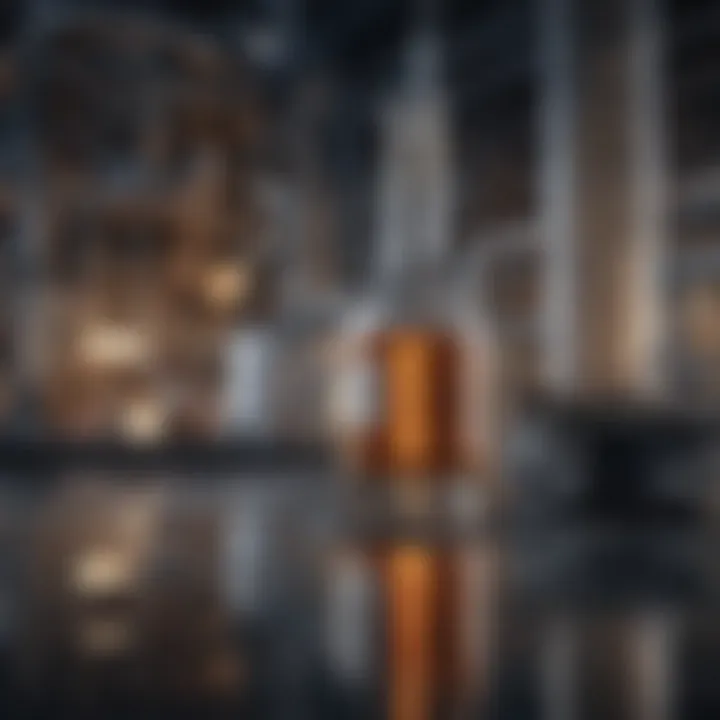
Conclusively, optimizing pressure settings is practical for enhancing reaction outcomes in industrial applications.
Solvent Impact
The choice of solvent can notably affect the hydrogenolysis process by impacting reaction rates, selectivity, and solubility of reactants. Solvents can offer a medium where reactants interact, and they may assist in stabilizing transition states or intermediates during the reaction. Some crucial considerations regarding solvent impact are:
- Polarity of Solvent: The nature of the solvent can either stabilize charged intermediates or facilitate bond cleavage.
- Protic vs. Aprotic Solvents: These can influence which products are favored, affecting overall selectivity.
- Viscosity and Solvent Properties: These factors can dictate mixing efficiency and mass transfer, thus influencing reaction outcomes.
For researchers and industries pursuing efficiency in hydrogenolysis, selecting the appropriate solvent is instrumental in optimizing processes.
Effective manipulation of temperature, pressure, and solvent choice facilitates fine-tuning of hydrogenolysis, improving its application across various sectors such as organic synthesis and petrochemicals.
Environmental Implications
The environmental ramifications of hydrogenolysis are significant in discussing its role in modern chemistry. This reaction not only provides vital pathways for organic synthesis but also aligns with the growing needs of sustainable practices. By delving into the ecological aspects, we can appreciate how hydrogenolysis contributes to reducing the environmental footprint of chemical production.
Impact on Green Chemistry
Hydrogenolysis fits well within the framework of green chemistry. This subset of chemistry emphasizes the design of chemical products and processes that minimize hazardous substances. Hydrogenolysis, in this sense, can often replace more polluting reactions. For example, traditional methods for alcohol deoxygenation produce hazardous byproducts, while hydrogenolysis mainly yields water and hydrocarbons, making it a cleaner alternative.
Some key benefits of hydrogenolysis in green chemistry include:
- Reduced waste generation
- Lower energy consumption compared to alternative processes
- Decreased use of toxic reagents
This transformation encourages researchers and industry to focus on eco-friendly processes, promoting a shift towards safer industrial practices. As these methods gain traction, hydrogenolysis serves as a model for other reactions striving for greener outcomes.
Sustainability Considerations
Beyond immediate environmental benefits, the sustainability aspects of hydrogenolysis are paramount. Its ability to utilize renewable feedstocks, such as biomass, highlights the potential for integrating this reaction into a circular economy. With the demand for sustainable practices rising, hydrogenolysis is becoming an essential technique.
Important considerations within sustainability include:
- Use of Renewable Resources: Hydrogenolysis can convert renewable biomass into valuable chemicals, reducing reliance on fossil fuels.
- Energy Efficiency: The reaction can be conducted under milder conditions, minimizing energy needs and emissions.
- Carbon Neutrality Potential: If hydrogen is derived from renewable sources, hydrogenolysis can offer pathways to carbon-neutral production cycles.
The future of hydrogenolysis is intrinsically linked to advancements in sustainability. As industries increasingly prioritize environmental responsibility, this reaction stands at the forefront of chemistry's evolution towards a sustainable future.
Hydrogenolysis is not simply a chemical transformation; it is a cornerstone of greener, more sustainable industrial practices.
Future Directions in Hydrogenolysis Research
Research in hydrogenolysis is rapidly evolving. This field presents exciting opportunities for developing new catalysts and enhancing existing methods. As researchers strive to address the challenges posed by this complex reaction, they are exploring various innovative avenues. These directions are crucial for advancing the applications of hydrogenolysis in both chemical synthesis and sustainability initiatives.
Innovative Catalysts
In hydrogenolysis, the choice of catalyst plays a decisive role. Innovative catalysts can significantly improve reaction efficiency and selectivity. Recent trends include the development of metal-organic frameworks and nanostructured catalysts. These new materials often show enhanced activity and stability compared to traditional catalysts.
Research has demonstrated that using supported metal catalysts, such as palladium and platinum, combined with unique supports like carbon nanotubes, boosts the performance of hydrogenolysis reactions. This is particularly relevant for synthesizing valuable chemicals from complex feedstocks. Moreover, a focus on earth-abundant metals instead of precious ones could lead to more sustainable and economically feasible processes.
Key aspects of innovative catalysts include:
- Increased surface area for better interaction with substrates
- Enhanced resistance to deactivation over time
- Ability to function under milder conditions
Integration with Renewable Resources
The integration of hydrogenolysis with renewable resources is an important area of research. As the world seeks greener alternatives for chemical production, utilizing biomass and waste materials presents an appealing method to produce sustainable feedstocks. Hydrogenolysis can convert lignocellulosic biomass into valuable intermediates, like alcohols and hydrocarbons, contributing to a circular economy.
Moreover, coupling hydrogenolysis with renewable hydrogen production methods, such as electrolysis or biogas reforming, can further minimize the environmental impact. This approach not only reduces CO2 emissions but also utilizes waste resources effectively.
"Innovations in hydrogenolysis not only improve process efficiency but also contribute to the vital goals of sustainability in chemistry."
Case Studies of Successful Hydrogenolysis
Case studies provide a concrete illustration of the practical application of hydrogenolysis in both industrial and academic settings. They reveal how theoretical mechanisms and catalysis research are applied to solve real-world problems, advancing fields such as organic synthesis and petrochemical processing. This section highlights notable examples, emphasizing the significance of hydrogenolysis in commercial ventures and academic pursuits.
Notable Industrial Applications
Industrial applications of hydrogenolysis showcase its versatility and effectiveness. Various sectors have utilized this reaction for efficient chemical transformations. For instance, in the petrochemical industry, hydrogenolysis processes are essential for refining crude oil. Skipping over high-reactivity bonds provides cleaner products while reducing undesirable by-products. Specifically, companies such as ExxonMobil have leveraged hydrogenolysis to upgrade residual oils, improving yields of valuable distillates.
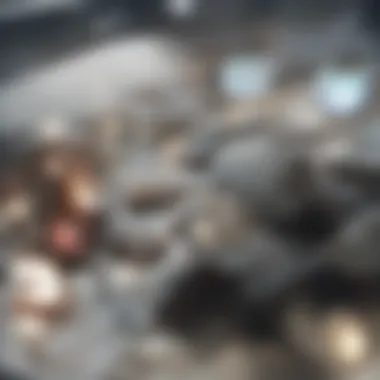
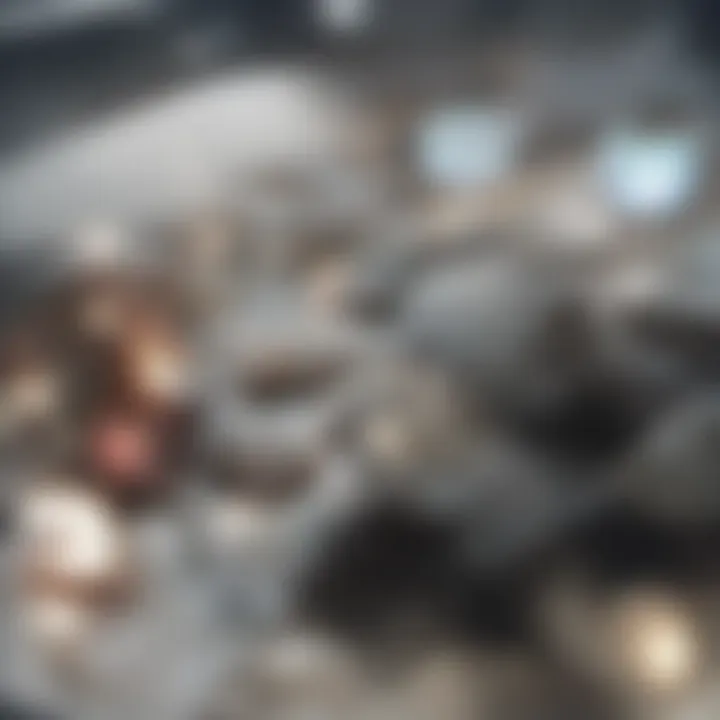
Another significant industrial use is the hydrogenolysis of complex organic molecules. This method is valuable for breaking down bio-derived feedstocks into simpler, useful components. For example, the conversion of cellulose into various alcohols has been studied extensively. This transformation serves as a promising pathway for producing renewable fuels and chemicals. Thus, the industrial applications of hydrogenolysis not only focus on economic gains but also contribute to sustainable practices in chemical manufacturing.
Academic Research Highlights
In academic research, hydrogenolysis garners attention for its potential to advance fundamental chemistry. Numerous studies have investigated the activation mechanisms of different catalysts in hydrogenolysis reactions. Research led by institutions like Massachusetts Institute of Technology has focused on understanding the interactions of catalysts with substrates at molecular levels. These investigations provide deeper insight into reaction pathways and mechanisms.
Moreover, collaborative studies between universities and industry often target specific challenges faced in commercial applications of hydrogenolysis. Here, research serves as a bridge between theory and practice, allowing academia to address real industrial needs. Notably, research articles exploring novel catalytic systems offer potential solutions to selectivity issues that commonly plague hydrogenolysis reactions. By tailoring catalyst design to specific substrates, researchers aim to enhance reaction efficiency and reduce by-products.
"Each case study exemplifies the fusion of theoretical knowledge with practical application, driving innovation in chemical processes."
In summary, the case studies discussed demonstrate how hydrogenolysis stands out in both industrial and academic sectors. These applications not only illustrate practical aspects but also motivate further exploration of hydrogenolysis mechanisms and processes.
Challenges in Hydrogenolysis
Hydrogenolysis presents several challenges that are crucial to acknowledge in understanding its overall impact and efficiency. These challenges can affect both the yield and selectivity of products, which have significant ramifications in practical applications. Grasping these aspects of hydrogenolysis is essential for researchers and practitioners striving to optimize processes and enhance outcomes.
Formation of By-Products
One notable challenge in hydrogenolysis is the formation of by-products. These by-products can complicate the purification of desired compounds, often leading to reduced overall yields. Reaction conditions greatly influence by-product formation. Increased temperature or pressure may accelerate reaction rates but also enhance side reactions. For example, during the hydrogenolysis of complex organic molecules, the creation of unwanted species such as alcohols and alkanes can detract from the main product goals.
Moreover, certain catalysts can have distinct tendencies to promote specific paths that create undesired by-products. Recognizing these dynamics is vital. Effective methods to minimize by-product formation include optimizing reaction conditions, using selective catalysts, and employing purification strategies. Indeed, understanding and controlling by-product formation remains a focus area in advancing hydrogenolysis techniques.
Selectivity Issues
Another challenge is related to selectivity issues. This area is particularly important because achieving high selectivity in hydrogenolysis reactions is necessary to direct the transformation toward intended products. Several factors can lead to poor selectivity, including catalyst choice and reaction environment.
Often, multiple reactive sites in a substrate can lead to a mixture of products unless selective catalysts are used. For instance, in converting lignocellulosic biomass, varying selectivity can determine whether the process yields desired sugars or generates mixed hydrocarbons instead. Variation in conditions such as solvent type and concentration can also shift reaction pathways, further complicating selectivity.
To address selectivity, researchers aim to develop catalysts with tailored properties. Innovations in catalyst design may improve selectivity by enhancing active site characteristics through molecular engineering. The future success of hydrogenolysis lies, in part, within solving the challenges of selectivity, ensuring that reactions yield the primary intended products rather than undesired by-products.
Addressing hydrogenolysis challenges is crucial for advancing both academic understanding and industrial applications. Optimizing conditions and innovating catalyst designs can significantly improve outcomes.
Comparative Analysis with Other Reactions
Understanding hydrogenolysis requires comparing it with similar reactions such as hydrogenation and hydrocracking. This comparative analysis sheds light on the unique characteristics and advantages of hydrogenolysis. It has crucial implications for research and industry applications. By examining these relationships, we gain deeper insights into reaction mechanisms, catalyst interactions, and the selection of conditions for optimized outcomes.
Hydrogenation vs. Hydrogenolysis
Hydrogenation and hydrogenolysis share common features as both involve hydrogen and impact carbon bonds. However, they serve distinct purposes in chemical synthesis.
- Hydrogenation refers to the addition of hydrogen across double or triple carbon bonds, converting unsaturated compounds to saturated ones. This process mainly increases the stability and saturation of organic compounds.
- Hydrogenolysis, in contrast, specifically breaks carbon-to-carbon or carbon-to-heteroatom bonds. It results in smaller fragments from larger molecules, often producing desired smaller molecules or specific functional groups in organic synthesis.
Consider the following key elements of comparison:
- Mechanistic Pathways: Hydrogenation typically follows addition mechanisms, while hydrogenolysis involves cleavage mechanisms, leading to different products.
- Catalyst Requirements: Both processes rely on catalysts but can differ in catalyst type and conditions optimal for each reaction.
- Applications: Hydrogenation is commonly used in food processing and fuel production, whereas hydrogenolysis finds its use in organic synthesis and petrochemical industries.
Thus, while both reactions utilize hydrogen, the outcomes and applications vary significantly. This distinction is critical, as it determines which process is preferable under certain conditions.
Hydrocracking Comparisons
Hydrocracking is another reaction that broadly overlaps with hydrogenolysis, often used in the oil industry. This process involves breaking larger hydrocarbons into smaller, more valuable fractions, utilizing hydrogen and catalysts. Nevertheless, the focus of hydrocracking is distinct in terms of structure and application.
- Reactivity and Conditions: Hydrocracking usually operates under harsher conditions of high pressure and temperature to facilitate the breakdown of complex hydrocarbons. Hydrogenolysis, however, may function effectively under milder conditions depending on the substrates and desired products.
- Selectivity: Hydrogenolysis tends to offer higher selectivity toward desired products compared to hydrocracking. This selectivity is critical for applications where specific outcomes are necessary, such as producing targeted chemicals in pharmaceutical synthesis.
- Catalysts: Both processes utilize catalysts, but the type and design can significantly differ. Hydrogenolysis catalysts can often be tailored for specific reactions, whereas hydrocracking catalysts are designed for bulk processing of petroleum feeds.
Comparative analysis of these reactions leads to better understanding of the hydrogenolysis reaction. Understanding their distinctions allows researchers to optimize processes for industrial applications, ultimately enhancing efficiency and product yield.
"In the world of chemistry, understanding connections between reactions enhances both theoretical knowledge and practical application."
Culmination on Hydrogenolysis
In the realm of modern chemistry, hydrogenolysis merits significant attention. This reaction, which involves cleaving various bonds in the presence of hydrogen, is integral to many industrial processes. Understanding the fundamental aspects of hydrogenolysis reinforces its relevance across multiple disciplines. This article articulately elucidates the diverse mechanisms and applications, showing how hydrogenolysis is not just a laboratory curiosity but a vital pillar in organic synthesis and the petrochemical industry.
Summary of Findings
Throughout the discussions in this article, we have established several key findings on hydrogenolysis:
- Mechanisms: The mechanisms involved in catalytic and non-catalytic hydrogenolysis are distinct, allowing for various applications and efficiencies. Catalysts play a crucial role in enhancing reaction rates and selectivity.
- Applications: Hydrogenolysis proves invaluable, especially in organic synthesis, where it aids in producing various chemical structures, and in the petrochemical industry for converting heavier fractions into lighter, more valuable components.
- Influencing Factors: Specific conditions such as temperature, pressure, and solvent can greatly influence the outcomes of hydrogenolysis reactions, which underscores the importance of optimizing these parameters.
- Environmental Considerations: The environmental implications of hydrogenolysis were addressed, emphasizing its potential role in sustainable practices within green chemistry frameworks.
These findings culminate in a clearer vision of the significance of hydrogenolysis in both academia and industry.
Implications for Future Research
As we look ahead, the implications for future research in hydrogenolysis are profound. Some critical aspects include:
- Innovative Catalysts: The development of new and more efficient catalysts can enhance reaction rates and reduce by-products. Future studies should focus on the design and discovery of catalysts that are not only effective but also sustainable.
- The Integration with Renewable Resources: Exploring the synergy between hydrogenolysis and renewable resources presents an opportunity to create more sustainable processes. Research into biomass conversion can offer a pathway to cleaner production methods.
- Mechanistic Studies: There is a continuous need for in-depth mechanistic studies to unravel the complexities of the different reaction pathways. Understanding these aspects can lead to better control over selectivity and yield during reactions.