Exploring E. coli Codon Usage Patterns and Impacts
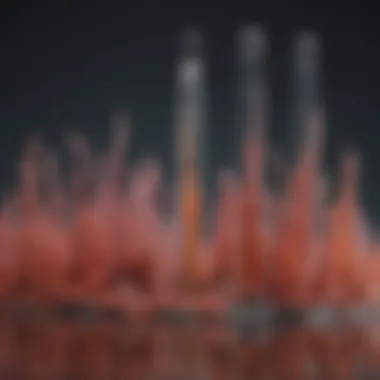
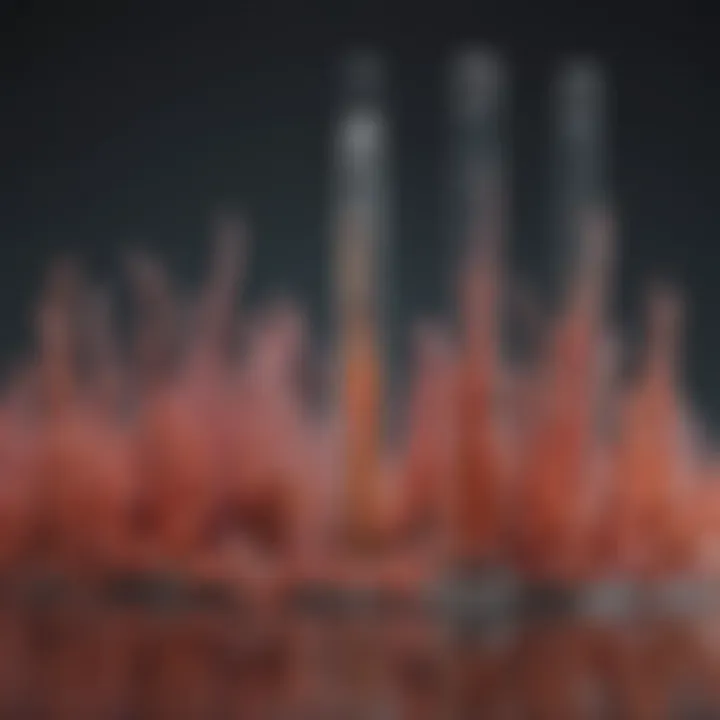
Intro
E. coli has a unique preference for certain codons over others, even though many codons can code for the same amino acid. This phenomenon is known as codon bias. The usage table showcases these preferences, and by examining it, one can uncover how these biases affect everything from translation speed to the stability of mRNA. Understanding these dynamics is important not only for fundamental biology but also for applications in biotechnology and synthetic biology, where optimizing gene expression is crucial.
In this article, we will break down the key findings, explore the implications of this research, and delve into how these insights can shape future studies and innovations in molecular biology.
Prolusion to Codon Usage
Understanding codon usage is pivotal in the realm of molecular biology. Codons, which are sequences of three nucleotides, serve as the building blocks of genetic coding. They are essential for translating the genetic code into proteins, which are crucial for cellular structure and function. This section will delve into what codons are, their significance in genetic expression, and how variations in codon usage can influence biological processes.
The implications of codon usage extend beyond mere academic curiosity. For researchers and biotechnologists, grasping the nuances of codon preferences can enhance gene expression in various organisms, notably Escherichia coli, or E. coli. The differences in how various organisms utilize codons can shed light on evolutionary trajectories, adaptation mechanisms, and even guide the optimization of synthetic genes. This understanding is invaluable for applications in areas such as genetic engineering and synthetic biology.
Definition of Codons
A codon is a triplet of nucleotides found in DNA or RNA that corresponds to a specific amino acid or stop signal during protein synthesis. Each amino acid is coded for by one or more codons, making the genetic code somewhat redundant. For example, the amino acid leucine is encoded by six different codons. This redundancy can be critical; it allows some flexibility in the coding process, providing a buffer against mutations. However, it's not just a random assortment of triplets.
Codons are categorized into two main types:
- Sense codons: These codons specify amino acids. There are 61 sense codons in total, each linked to a particular amino acid.
- Stop codons: These signal the termination of protein synthesis and do not code for any amino acid. There are three stop codons: UAA, UAG, and UGA in RNA.
The way these codons are used can vary significantly across different organisms, influenced by evolutionary history, selection pressures, and environmental factors.
Codon Usage in Different Organisms
Codon usage differs from one organism to another, greatly affected by factors such as genomic composition, evolutionary adaptations, and their respective environments. This variability is crucial for understanding how organisms express their genes effectively in their specific contexts.
For instance, bacteria like E. coli and higher organisms such as mammals exhibit distinct patterns in codon usage.
- Bacteria: They often possess a strong preference for certain synonymous codons. This preference can enhance the efficiency of protein production, allowing them to quickly respond to environmental changes.
- Eukaryotes: In contrast, multicellular organisms may exhibit a more balanced usage of codons. This reflects their complex cellular organization and the intricate regulation of gene expression.
Interestingly, even closely related species can show notable differences in codon usage. Such deviations can influence not only the rate of protein synthesis but also the folding and stability of produced proteins. For example, a codon that is more readily recognized by the translational machinery can lead to faster protein assembly, ultimately affecting the organism's growth and adaptation.
E. coli as a Model Organism
Escherichia coli, commonly referred to as E. coli, has long found its footing as a favored model organism in molecular biology and genetic research. There are multifaceted reasons why the study of E. coli is paramount, and this section aims to illuminate the significance of this bacterium. Its accessibility, rapid growth rate, and well-mapped genetics make it an invaluable asset in research fields ranging from biotechnology to medicine.
Why Study E. coli?
E. coli's status as a model organism isn’t just by chance. Here are some pressing reasons to consider:
- Genetic Simplicity: The genetic makeup of E. coli is considerably straightforward, allowing for easy manipulation. Researchers can introduce modifications to E. coli’s DNA to observe gene interactions and functions, opening pathways to understand complex genetic systems in other organisms.
- Fast Growth: With a generation time of about 20 minutes under optimal conditions, E. coli can yield results quickly. This rapid proliferation allows researchers to conduct experiments and see results in a timeframe that would be impractical with slower-growing organisms.
- Extensive Knowledge Base: E. coli is arguably one of the most studied organisms in science. Scientists have a treasure trove of resources, including genetic libraries, pathways, and functions available at their fingertips. This vast repository makes it easier to draw parallels and contrasts with other life forms.
- Safety: Certain strains of E. coli are harmless, making them suitable for use in laboratory settings. The potential for risk is minimized when handling these strains, which is essential for researchers working in public laboratories.
Additionally, E. coli has often been engineered for various applications, including the production of insulin, enzymes, and even biofuels. This versatility displays the important role that E. coli plays in both academic and practical realms, making it a cornerstone of modern biology.
Historical Context
The journey of E. coli as a model organism began in the early 20th century, when it was first isolated by Theodor Escherich in 1885. Its genetic complexity started to unfold significantly in the 1950s. Key milestones in this timeline were pivotal:
- Molecular Genetics: The development of recombinant DNA technology in the 1970s catalyzed a revolution in genetics. E. coli became a primary host for gene cloning since researchers could easily incorporate segments of DNA into its plasmids.
- Gene Expression Studies: With advancements in genetic engineering, it became increasingly clear that E. coli could serve as a scaffold for understanding more intricate gene expression systems across different organisms.
- Multinational Studies: By the 1980s, E. coli was a staple in labs globally, spurring international collaborations focusing on gene therapy and vaccine production, with breakthroughs occurring rapidly.
- Genomic Advances: In the 1990s, E. coli was one of the first organisms to have its entire genome sequenced, providing an essential resource for scientists to explore gene functions, interactions, and pathways.
"When a microbe like E. coli becomes the reference for modern molecular biology, it embodies how vital even the simplest forms of life can be in unraveling complex questions."
Through decades of research and innovation, the importance of E. coli as a model organism has grown tenfold. The ongoing exploration of its genetics and biology continues to pave the way for advancements across diverse fields, affirming E. coli's legacy as a fundamental tool in scientific investigation. From basic research to cutting-edge applications, this bacterium's influence is felt across the global scientific community.
The E. coli Codon Usage Table Explained
In the context of E. coli, codon usage becomes a language that speaks volumes about efficiency and regulation. Analyzing these patterns can drive innovations, enhance research in synthetic biology, and refine methodologies in genetic engineering.
Structure of the Codon Usage Table
At first glance, the codon usage table for E. coli may seem daunting, but understanding its structure is key to decoding the information it holds. The table is primarily organized as a grid where the rows correspond to the 64 possible codons, each representing a set of three nucleotides. These codons are classified into two groups: synonymous, which correspond to the same amino acid, and non-synonymous, which result in different amino acids.
- The first two columns typically indicate the triplet codon and its associated amino acid.
- The next columns provide the frequency of each codon used within E. coli. These frequencies usually appear as integers or percentage values, showcasing how often a particular codon appears for a given amino acid.
- An additional column or footnotes may detail the relative codon adaptiveness, helping to demonstrate preferential codon usage tied to the organism's overall fitness and efficiency in translation.
Here’s a mini layout for clarity:
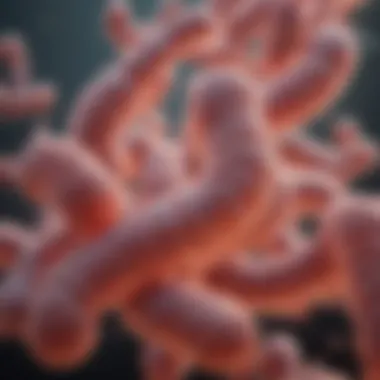
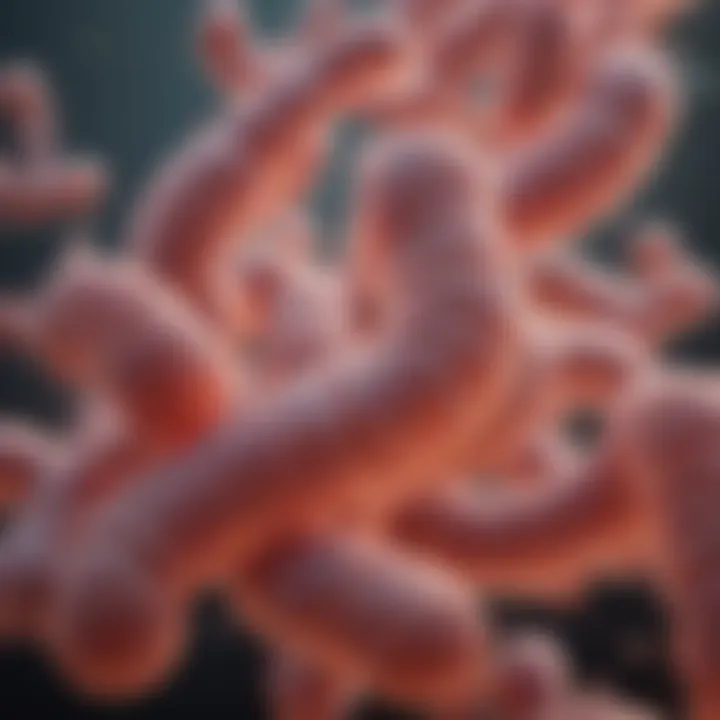
| Codon | Amino Acid | Frequency | Relative Adaptiveness |
| AUG | Methionine | 100 | High |
| CUG | Leucine | 50 | Moderate |
This structure not only conveys essential data but also serves as a foundation for further investigation into genetic expression. Each codon’s frequency can signal how optimized a sequence is for efficient translation into protein, offering a guiding light for genetic manipulations.
Interpretation of Values
When looking at values within the codon usage table, it's important to interpret them thoughtfully. Frequencies tell us a story; a high frequency of a certain codon may indicate that E. coli has adapted to utilize that codon more efficiently for rapid protein synthesis.
- High Frequency Codons: Typically preferred by the organism, they correlate with faster translation times and may relate to the prevalence of that particular codon in highly expressed genes.
- Low Frequency Codons: These may signify alternative pathways and can hint at potential bottlenecks in protein production. Genes that utilize low frequency codons might be interesting candidates for optimization when designing synthetic constructs.
Understanding relative adaptiveness is also vital. Codons that are historically favored can provide insights into which sequences might be reorganized for a more effective outcome in experimental setups, whether for research or industrial applications.
"Exploring codon frequencies not only sheds light on E. coli's history but also creates a pathway for future innovations in genetic engineering."
Decoding these values plays a fundamental role in aligning experimental designs with the natural tendencies of E. coli, effectively blending the practices of molecular biology with real-world applications.
Being detail-oriented while interpreting these values helps to grasp the fundamental aspects influencing molecular processes, which ultimately contributes to advancing research in relevant fields.
By the end of this segment, it should be clear that dissecting the structure and meaning behind the E. coli codon usage table does more than present numbers; it offers a framework for understanding the biological intricacies that govern life at the molecular level.
Codon Bias and Its Implications
Codon bias plays a pivotal role in the landscape of genetic coding, particularly when delving into the codon usage patterns of Escherichia coli. This phenomenon refers to the observation that different organisms exhibit preferences for specific synonymous codons, which can significantly impact gene expression levels as well as the resultant protein structures. In E. coli, analyzing codon bias uncovers critical insights into its biology and functional capabilities.
Understanding codon bias is not merely an academic exercise; it has tangible implications for biotechnology and synthetic biology applications. The implications of codon bias stretch across various fields, from the optimization of gene expression in recombinant protein production to the tailoring of microorganisms for biotechnological advancements. Moreover, grasping these biases illuminates potential pitfalls in genetic engineering, guiding researchers to enhance expression efficiency and reduce unwanted by-products.
Understanding Codon Bias
At its core, codon bias is linked to how frequently different codons are utilized to code for the same amino acid within an organism’s genome. This variability arises due to several factors, including mutation rates, evolutionary pressures, and translational efficiency. The degree of codon bias varies among organisms:
- Highly biased: Organisms like E. coli tend to show pronounced preferences toward certain codons, driven by natural selection for efficient translation.
- Less biased: Other organisms may display more balanced codon usage, which can hinder protein synthesis efficiency at times.
Codon bias in E. coli is particularly relevant in studies of genetic engineering, where the introduction of foreign genes can lead to unexpected outcomes if codon usage is not aligned with that of the host organism. Thus, paying heed to codon bias fosters better predictive outcomes in protein production.
Factors Influencing Codon Bias in E. coli
Genomic GC Content
Genomic GC content refers to the proportion of guanine (G) and cytosine (C) nucleotides in an organism's DNA. In E. coli, a high GC content often correlates with specific codon usage preferences, as certain amino acids may be encoded more frequently by GC-rich codons. The relevance of genomic GC content in the context of codon bias cannot be overstated:
- Characteristic: E. coli's genomic GC content is approximately 50%, which influences the stability and expression of genes.
- Why it Matters: A higher GC content can favor the stability of the secondary structures of mRNA, ultimately enhancing translation efficiency.
- Unique Feature: GC-rich environments contribute to effective base-pairing, which can boost interactions with ribosomes.
However, having high GC content can also pose challenges. For instance, some GC-rich codons may lead to slower translation due to secondary structures that form during transcription, presenting a delicate balance in expression efficiency.
Comparison with Other Organisms
When examining codon bias, it's essential to consider how E. coli stacks up against other organisms. Different species exhibit unique biases that reflect their evolutionary paths. For example, Saccharomyces cerevisiae, a model yeast organism, shows constant yet distinct codon preferences compared to E. coli:
- Characteristic: While yeast may favor certain codons disposed towards high GC content, E. coli employs a varied mixture of G and A-rich codons.
- Why it Matters: This comparison underscores how evolutionary lineage and environmental pressures shape codon preferences.
- Unique Feature: Codon biases impact gene expression significantly, which can result in functional divergences in proteins among species.
Despite these differences, interactions among codons and overall translation rates remain a common thread that links these organisms. Misalignment between gene codons and host preferences can yield low expression levels or misfolded proteins when foreign genes are introduced into E. coli or any other host organism.
Ultimately, understanding these biases equips researchers to navigate the complexities of genetic design and synthetic biology more effectively. As genetic engineering continues to evolve, the implications of codon bias on experimental outcomes will only deepen, demanding a nuanced approach to designing functional genes.
Synonymous Codons in E. coli
The investigation of synonymous codons in E. coli is pivotal in understanding the genetic language by which proteins are synthesized. To clarify, synonymous codons are different codons that code for the same amino acid. For example, the amino acid leucine can be codified by the codons CUU, CUC, CUA, and CUG. With the knowledge of synonymous codons, researchers can grasp not just the primary translation process, but also the nuances that influence protein stability and expression.
Role of Synonymous Codons
Synonymous codons fulfill several essential functions within the cell. They are not mere substitutes; their usage can have a profound impact on gene expression levels and protein functionality. An important aspect to note is the codon adaptability, which refers to how frequently a particular synonymous codon occurs in the organism's overall genome. Higher frequency of certain codons aligns with abundant tRNA availability, which can accelerate the translation process.
Additionally, codon choice may influence the initiation and termination rates during protein synthesis. When a specific codon dominates, it could lower the risk of ribosome stalling, thus promoting efficient protein production. Moreover, the variation in synonymous codons can help in fine-tuning the expression of transgenes when engineered for various applications, particularly in synthetic biology and recombinant protein production.
Effects on Protein Folding and Stability
The implications of synonymous codon choice extend to protein folding and stability. When proteins are synthesized, the rate at which amino acids are added can impact the way a protein folds. Faster translation due to favored synonymous codons can result in correct folding pathways, while slower rates may lead to misfolding due to accumulation of intermediate states.
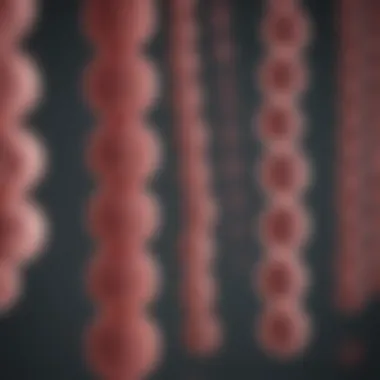
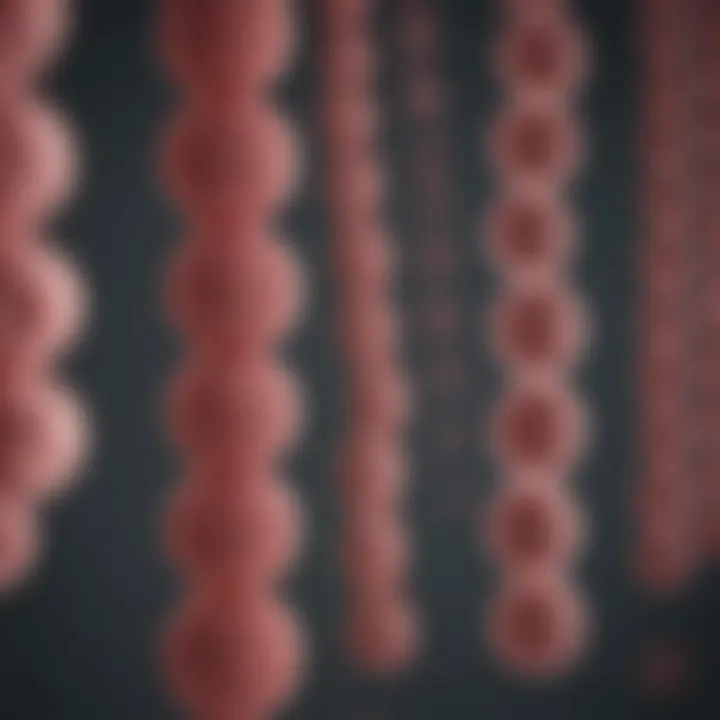
"It is essential to recognize that synonymous changes can significantly affect protein structure and function, which may not be immediately clear from the nucleotide sequence alone."
For instance, a study showed that certain synonymous codon pairs caused variations in the folding kinetics of proteins, leading to differences in the final structure and, consequently, their biological activity. Thus, understanding synonymous codons is not simply an exercise in semantics but critical for unraveling the complexities of gene expression and the functional attributes of produced proteins.
In summary, synonymous codons play a fundamental role in shaping how proteins are created in E. coli. Their influence extends beyond coding; they modulate translation dynamics, affect protein stability, and can dictate interactions within the cellular environment. Recognizing these facets helps to clarify why codon usage must be considered carefully in genetic engineering and metabolic pathway design.
Applications in Biotechnology
Biotechnology relies heavily on a precise understanding of genetic coding to address various challenges in medicine, agriculture, and environmental sustainability. Within this landscape, the E. coli codon usage table emerges as a critical tool that allows researchers to optimize gene expression. This has implications not only for laboratory experiments but also for real-world applications that can lead to innovative solutions in numerous fields. Understanding how to utilize this table means harnessing the potential of biological systems more effectively and efficiently.
Optimizing Codon Usage for Gene Expression
When designing genes for expression in E. coli, one must consider the codon usage within the organism's genetic makeup. Each organism exhibits a preference for certain codons over others, making it essential to align the codon usage of the foreign gene with that of E. coli. This alignment can significantly boost the levels of expressed protein.
For instance, a gene introduced into E. coli that features a high concentration of rarely-used codons may lead to lower protein yields. Such inefficiency often occurs because the ribosome struggles to find the necessary tRNAs to translate the less common codons. By tweaking the genetic code to use preferred codons, the efficiency of translation enhances, thus maximizing protein production.
To optimize codon usage effectively, consider these steps:
- Identify the codon usage bias in E. coli compared to the source organism.
- Modify the gene to replace non-preferred codons with synonymous preferred codons, a process known as codon optimization.
- Use codon optimization tools or software that can analyze exponential data on codon usage and provide recommendations.
By following these approaches, researchers improve gene expression dramatically, enabling the production of necessary proteins for various applications, from the creation of vaccines to the development of enzymes used in biofuels.
Recombinant Protein Production
Recombinant protein production stands as one of biotechnology's significant milestones, allowing researchers to generate large quantities of proteins that are otherwise difficult, if not impossible, to produce directly from natural sources. E. coli, due to its rapid growth rate and well-characterized genetics, becomes the organism of choice for recombinant DNA technology.
In the context of recombinant protein production, the E. coli codon usage table plays a substantial role. Once again, aligning the introduced gene's codon usage with that of E. coli simplifies the translation process and minimizes unintended outcomes, such as poor protein folding or inclusion body formation.
Key considerations for successful recombinant protein production in E. coli include:
- Choice of expression vector: Vectors carry the gene and must be compatible with E. coli.
- Host strain selection: Specific E. coli strains are engineered to enhance protein yield, stability, or post-translational modifications.
- Growth conditions: The temperature, nutrient availability, and method of induction can impact the protein yield.
"The success of recombinant protein production is not just about inserting a gene; it's about creating the right environment for that gene to express optimally."
In summary, by utilizing the E. coli codon usage table effectively, researchers can enhance their efforts towards maximization of gene expression and the successful production of recombinant proteins. The innumerable applications in fields such as therapeutic development or industrial enzyme production highlight the ongoing importance of understanding and manipulating codon usage in this model organism.
Synthetic Biology and Codon Optimization
Synthetic biology is a rapidly evolving field that combines principles from biology, engineering, and computer science to create new biological parts and systems. One of the pivotal elements within this discipline is the concept of codon optimization. Understanding this relationship is essential for enhancing the efficiency of gene expression, particularly in organisms like Escherichia coli, which serves as a workhorse for synthetic biology applications.
Codon optimization involves modifying the codons in a given DNA sequence to match the preferred codon usage of the host organism. This can significantly improve protein yields during gene expression. By adjusting these sequences, researchers can ensure that the translated proteins fold correctly and function properly. The advantages of codon optimization include improved translation speed, enhanced protein folding, and increased stability of the final product. Moreover, tailored codon usage minimizes the risk of misfolded proteins, which can lead to aggregation or inactivity.
In the broader context, codon optimization is not merely an academic exercise; it has profound implications in biotechnology. This tailoring of genetic sequences can lead to better yields in vaccine production, biopharmaceuticals, and enzyme development. Here are some of the specific benefits of codon optimization:
- Increased Protein Production: Enhanced translation efficiency allows cells to produce more proteins than would ordinarily be the case.
- Cost Efficiency: Higher yields lead to reduced production costs, making the whole process more economically viable.
- Enhanced Activity: Optimized proteins often show greater activity, contributing to the overall success of the engineered system.
However, the journey of codon optimization is not without its challenges. Each organism has a unique codon bias, influenced by its evolutionary history and environmental factors. Thus, a one-size-fits-all approach doesn’t cut it. Tailoring codon sequences to specific conditions or host characteristics pertains to the art and science of synthetic biology.
"Optimizing codon usage is like customizing a recipe; you must understand the taste preferences of your target audience to achieve the best results."
By engaging deeply with codon optimization, scientists can unlock new frontiers in genetic engineering. This enhances not only our understanding of genetic codes but also paves the way for tailored solutions in microbial production systems, addressing a wide array of scientific and industrial challenges.
Designing Artificial Genes
The process of designing artificial genes involves incorporating synthetic elements into a genome to create novel functionalities. This practice is a key aspect of synthetic biology, where the accurate understanding of codon usage tables facilitates the development of these artificial constructs. By employing codon optimization techniques, researchers can engineer genes that perform specific functions more efficiently in their chosen host organisms.
Every aspect of gene design must consider the target organism's codon usage preferences. The challenge lies in achieving the right balance between novel gene function and compatibility with the host’s translational machinery. When artificial genes are incorporated, using synonymous codons that the organism favors can dramatically enhance expression levels. This is particularly important when aiming to produce complex proteins or enzymes for industrial processes.
In the endeavor of designing artificial genes, a software-driven approach can be taken. Algorithms can aid in selecting the optimal codon sequence based on detailed analysis of existing codon usage tables. This data-backed approach helps in ensuring that the artificial gene not only encodes the desired protein but also does so efficiently.
Impact on Microbial Engineering
Microbial engineering harnesses the capabilities of microorganisms to create products or solve problems. The role of codon optimization in this area cannot be overstated. Changing a microbial strain’s genetic makeup through the design and insertion of artificial genes allows for the exploration of new biochemical pathways. It broadens the scope of what can be achieved with synthetic biology.
By utilizing optimized codon usage in microbial strains, researchers can spur the production of metabolites that are otherwise challenging or inefficient to obtain. For instance:
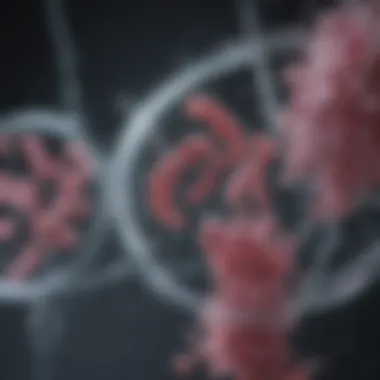
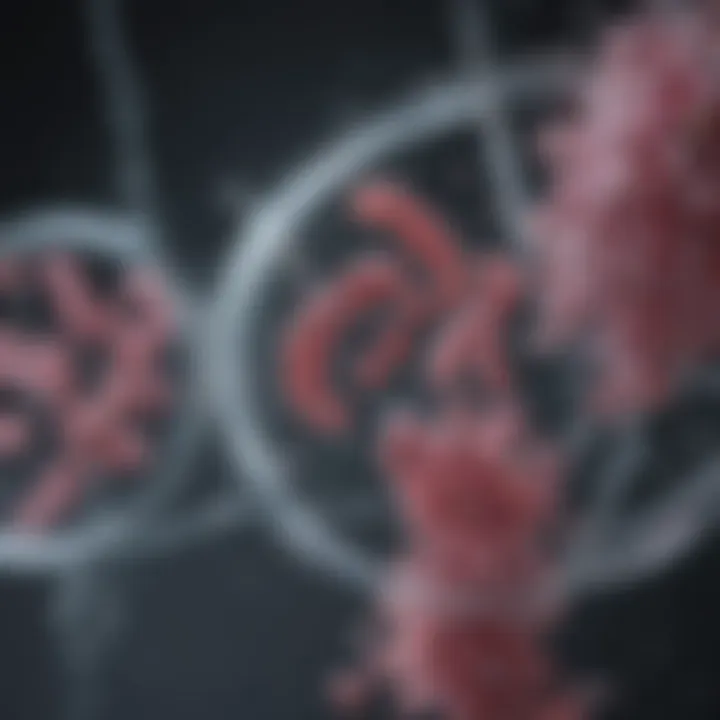
- Biofuel Production: Engineered microbes can be optimized to yield higher amounts of biofuels, contributing to sustainable energy solutions.
- Bioremediation Efforts: Optimized microbial strains can effectively degrade pollutants, proving useful in environmental cleanup efforts.
- Pharmaceutical Development: Engineered microbes can be guided to synthesize complex drugs, making the production process more efficient.
However, researchers must remain vigilant regarding the stability and reproducibility of these engineered systems, ensuring that the changes bring about consistent results across various environments.
In summary, codon optimization stands as a foundational strategy in synthetic biology and microbial engineering, propelling advancements in diverse fields, from medical therapeutics to sustainable environmental solutions.
Current Research and Developments
The field of codon usage is rapidly evolving, shedding light on the complexities of genetic expression in microorganisms. Understanding the nuances of codon usage in E. coli is not just an academic endeavor; it has practical implications in various sectors, particularly in biotechnology and synthetic biology. Recent studies have started to focus on the intricate patterns of codon usage, revealing that these nuances can affect everything from protein production to the stability of genetic constructs.
Recent Findings in Codon Usage Patterns
In the past few years, researchers have discovered that codon usage in E. coli varies significantly under different environmental conditions. For example, some studies observed that cells adaptively shift their codon preferences based on nutrient availability and temperature. These shifts can lead to optimizing protein synthesis pathways. Notably, researchers found that the codon usage can also correlate with the expression levels of different genes, affecting overall cellular performance.
Moreover, the concept of "codon deoptimization" has emerged as a fascinating area of research. This strategy intentionally alters codon usage patterns to reduce the expression of specific proteins, which could be beneficial in engineering attenuated strains of bacteria for vaccine production.
Codon usage patterns are a key element in the genetic toolkit of E. coli, influencing everything from basic metabolic functions to advanced applications in biotechnology.
Future Directions in Codon Research
Looking ahead, there are several promising directions for future research in codon usage patterns for E. coli. One significant avenue is the integration of machine learning techniques into codon optimization. By employing AI algorithms, researchers can predict optimal codon usage for specific genes in a variety of contexts, enhancing the efficiency of gene expression.
Another potential area is the investigation into non-canonical codons. Understanding how these less common codons impact translation efficiency could lead to new insights in protein engineering and synthetic biology, opening doors to innovative applications. As the methods for gene editing continue to mature, researchers may find ways to incorporate these insights into comprehensive genetic engineering strategies.
In addition, collaborations between computational biologists and experimentalists can foster the development of tools that allow for real-time tracking of codon performance in living cells. Such interdisciplinary research could lead to better prediction of gene expression outcomes and refined methodologies for synthetic gene design.
Finally, ethical considerations surrounding codon optimization must also be explored, especially as the field advances into more complex genetic manipulations. Striking a balance between innovation and ethical responsibility will be crucial as we harness the power of codon optimization for the benefit of mankind.
Challenges and Limitations
The study of codon usage in E. coli and other organisms is not without its hurdles. Understanding these challenges is crucial for researchers, as it dictates the efficacy of various applications in genetic engineering and synthetic biology. The implications of codon usage are nuanced, and appreciating the limitations can lead to more informed approaches in biotechnological endeavors.
Limitations of the Codon Usage Table
When delving into the codon usage table of E. coli, one must recognize that this tool, while beneficial, is fundamentally limited in scope. Here are some of the key limitations that bear consideration:
- Static Representation: The codon usage table tends to depict a snapshot in time. This means that, while it can provide insights based on existing data, it may not encompass the full range of codon biases present in different E. coli strains or under varying environmental conditions.
- Contextual Variability: The efficiency of codon usage does not operate in a vacuum. Factors such as the presence of specific transcription factors or ribosomal conditions can vastly influence which codon is preferentially used during translation. Such nuances may escape the simplicity of a standard table.
- Evolutionary Insight: Codon usage among organisms evolves over time. Consequently, a codon usage table may reflect an organism's natural history more than its current ecological context. This can hinder efforts to apply findings across different strains or species.
- Limited Predictive Power: While the table sheds light on historical trends, it does not predict how an introduced gene will perform in a new environment or host. This can lead to unforeseen challenges during gene expression experiments.
"The true test of a codon usage table lies not just in its data but in its application. Without a nuanced understanding, researchers may find themselves adrift in their projects."
Despite these constraints, the codon usage table remains an integral component in molecular biology, serving as a guide rather than an infallible manual.
Ethical Considerations in Genetic Engineering
The intersection of codon usage research and genetic engineering invites a wave of ethical considerations that cannot be overlooked. As researchers tread into the contentious waters of genetic manipulation, various issues arise:
- Modification of Life Forms: When we alter the genetic code of an organism, even one as simple as E. coli, we engage in a complex dialogue about the morality of such actions. What defines an organism’s identity? Is it ethical to change that?
- Biodiversity Risks: Introducing engineered models into the environment holds the potential to disrupt existing ecosystems. This raises the question of whether we are willing to gamble biodiversity for the sake of the advancements in biotechnology that might arise.
- Dual-Use Dilemma: Codon optimization can be harnessed for beneficial purposes, such as vaccine development, yet the same techniques could also be misappropriated for harmful agendas. The knowledge gained from codon usage studies, if misused, poses threats that require strict controls and responsible practices.
- Informed Consent: As genetic manipulation becomes increasingly prevalent, there is a pressing need for transparency and informed consent, especially when research involves bioengineering that may impact human health or agriculture.
Navigating these ethical waters requires a balance between scientific innovation and responsible stewardship of the living world. It is within this critical framework that the study of codon usage finds its fuller meaning—not merely as a scientific endeavor, but as a societal one.
Epilogue
The conclusion of this article ties together the various themes woven throughout the examination of the E. coli codon usage table. This section serves not just to summarize the findings but also to underscore the broader implications of understanding codon usage in the realms of genetics and biotechnology. As we’ve explored, codons play a crucial role in determining how genes are expressed in E. coli, shaping both the efficiency and accuracy of protein synthesis. The nuances of codon bias and synonymous codon roles have far-reaching consequences, not only for microbial engineering but also for the design of synthetic genes that are essential in research and industrial applications.
Studying E. coli's codon usage is akin to paving the way for advancements in genetic engineering. It allows scientists and researchers to tailor gene expression according to specific needs, thereby enhancing the viability of recombinant protein production and the development of novel therapies. By analyzing the intrinsic patterns of codon translation, researchers can develop predictive models, translating these insights into practical solutions that could revolutionize various fields—ranging from medicine to agriculture.
"Understanding codon usage is art and science intertwined, a road map that guides genetic manipulation to foster innovation and discovery."
Recognizing and applying the principles covered throughout this article can lead to more efficient methodologies, thereby maximizing resource use and reducing costs. As we shift towards increasingly intricate genetic applications, the synthesis of knowledge gained from the codon usage table becomes more significant. It's not merely a chart; it’s a vital tool that aids researchers in navigating the complex genetic landscape, shaping tomorrow’s breakthroughs.
Summary of Key Points
Reflecting on the important insights drawn from the exploration of E. coli's codon usage, several key points emerge:
- Role of Codons: Codons are essential units in genetic coding, influencing how proteins are synthesized in E. coli and other organisms.
- Codon Bias: There exists a preference among certain codons; understanding this bias can lead to improvements in gene expression optimization.
- Synonymous Codons: These codons, while coding for the same amino acid, can exhibit varying efficiencies in translation, impacting protein yield and folding.
- Biotechnological Implications: The applications of this knowledge extend to recombinant protein production, where codon optimization plays a pivotal role in enhancing yield and stability.
- Future Directions: Continuous research into codon usage patterns is essential to stay ahead in the rapidly evolving field of genetic engineering.
Implications for Future Research
Looking ahead, the insights gained from the E. coli codon usage table lay a strong foundation for further inquiry and innovation:
- Refinement of Gene Design Approaches: Future research can focus on refining gene design techniques, utilizing codon optimization to ensure higher expression levels in diverse organisms.
- Exploring Codon Usage in Other Organisms: Investigating how codon bias differs across various species can lead to a deeper understanding of evolutionary adaptations and help in cross-species gene expression.
- Ethical Considerations: The ethical implications of genetic manipulation must be a focal point, as advancements in biotechnology can lead to profound societal impacts.
- Integration with AI and Bioinformatics: Utilizing AI to analyze codon usage data could open new avenues for predictive modeling, enabling the design of synthetic biology applications more efficiently.
The remarkable insights from this brief exploration highlight not only the complexity of genetic coding but also the promising avenues available for future research. As our techniques advance and our understanding deepens, the implications of codon usage will no doubt play a significant role in shaping the future landscape of molecular biology.