Decoding DNA: Understanding Genetic Information
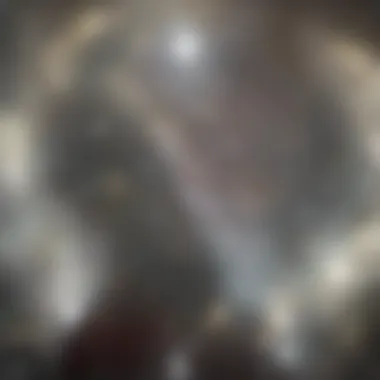
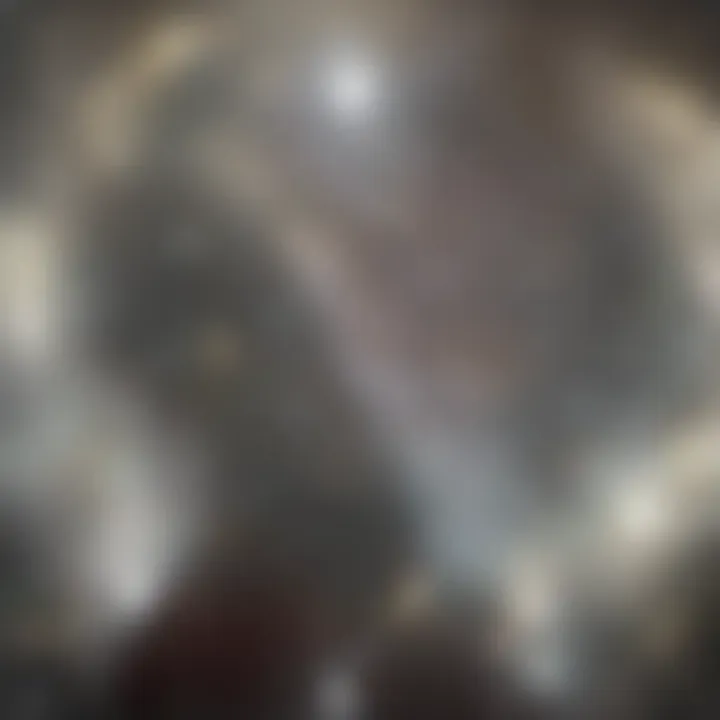
Intro
Understanding DNA is fundamental to many fields, including genetics, biology, and medicine. This guide aims to equip you with essential knowledge to read and interpret DNA code effectively. Over the coming sections, we will explore the structure of DNA, the significance of genetic sequences, and advances in technology that enhance our capability to analyze genetic information.
DNA, or deoxyribonucleic acid, consists of two intertwined strands forming a double helix. Each strand comprises smaller units known as nucleotides. There are four types of nucleotides in DNA represented by the letters A, T, C, and G. These letters stand for the nitrogenous bases adenine, thymine, cytosine, and guanine. The specific arrangements of these bases encode genetic information.
The knowledge of how to read this code can lead to breakthroughs in various areas, such as understanding hereditary diseases, developing personalized medicine, and advancing biotechnology. This guide provides a pathway through the often-convoluted landscape of DNA analysis, making it accessible and understandable.
Key Findings
In the process of reading DNA, several key elements emerge:
- Nucleotide Patterns: The sequences in which nucleotides appear play a critical role in determining genetic traits.
- Structural Elements: Genes, composed of DNA segments, are the fundamental units of heredity.
- Technological Advances: Innovations like CRISPR and DNA sequencing technologies have revolutionized our understanding of genetic codes.
The significance of these findings cannot be overstated. The ability to decode DNA sequences has profound implications within the scientific community. It supports more precise genetic engineering, enhances the understanding of evolutionary biology, and offers insights into pathogen genomic structures.
"The understanding of DNA has opened new frontiers in biomedical research and genetic therapy, paving the way for innovations that were once thought impossible."
Implications of the Research
The applications of DNA decoding extend far beyond the lab:
- Medical Diagnostics: Genetic testing can identify predisposition to diseases, enabling early intervention.
- Agriculture: Genetically modified organisms can be designed for resilience and yield improvement.
- Criminal Justice: DNA analysis plays a crucial role in forensic science to solve crimes or identify victims.
The potential impacts on future research directions are equally significant. As our tools become more advanced, the precision with which we can manipulate and understand DNA enhances. The ethical implications also arise, requiring careful consideration in areas like gene therapy and human enhancement.
Overall, becoming adept at reading DNA code offers a valuable skill set in many domains. As research advances, the understanding of DNA will continue to be at the forefront of scientific inquiry.
Prolusion to DNA
The exploration of DNA is fundamental for understanding the intricacies of life. DNA serves as the blueprint for living organisms, guiding the formation and functioning of every biological system. Its role reaches far beyond mere heredity, encompassing aspects of evolution, disease, and even forensic science. This section aims to lay the groundwork for your comprehension of DNA, allowing for a deeper dive into the code that underpins genetic material.
Definition of DNA
Deoxyribonucleic acid, commonly known as DNA, is a molecule that contains the instructions necessary for the development and functioning of all living organisms. Structurally, DNA is composed of two long strands forming a double helix. Each strand consists of a sequence of nucleotides, which are the building blocks of DNA. Each nucleotide, in turn, includes three components: a phosphate group, a sugar molecule, and a nitrogenous base. The sequence of these nitrogenous bases—adenine, thymine, cytosine, and guanine—encodes genetic information.
DNA not only carries genetic instructions but also plays a vital role in the transmission of these instructions from one generation to the next. The unique combinations of nitrogenous bases create the specific genetic code that defines an organism’s traits and characteristics.
Importance of DNA in Biology
The significance of DNA in biology cannot be overstated. It serves numerous essential functions:
- Genetic Information Storage: DNA is largely responsible for storing and transmitting genetic information across generations. Mutations and variations occur in this code, leading to diversity within species.
- Role in Protein Synthesis: DNA provides the instructions needed for synthesizing proteins, which are crucial for the structure, function, and regulation of the body's tissues and organs. This synthesis involves processes like transcription and translation, which translate the DNA code into functional proteins.
- Mechanism of Evolution: The changes and variations in DNA sequences contribute to the evolution of species. Natural selection acts on these changes, aiding in adaptation to changing environments.
- Medical Relevance: Understanding DNA is essential for advancements in medicine. Genetic research has led to breakthroughs in diagnosing and treating genetic disorders, understanding cancers, and developing targeted therapies.
"DNA is the molecule that encodes the genetic instructions. Understanding it is key to unlocking the mysteries of biology and medicine."
In summary, DNA is not just a molecular structure. It is the foundational element that supports life itself, making its study crucial for scientific progress across various domains.
The Structure of DNA
Understanding the structure of DNA is crucial in comprehending how genetic information is stored and transmitted in living organisms. The architecture of DNA is not just a physical formation; it plays a fundamental role in processes such as replication, transcription, and translation. Deciphering how DNA is organized helps researchers identify genetic disorders and develop biotechnological applications.
Components of DNA
Deoxyribonucleic acid
Deoxyribonucleic acid, commonly known as DNA, serves as the blueprint for all living organisms. Its double helical structure is a key characteristic. The main function of DNA is to store and pass along genetic information. This attribute makes it a popular subject in genetics studies. DNA's unique feature is its capacity to replicate itself, ensuring the continuity of genetic information from one generation to the next. However, it can also be a disadvantage, as errors during replication can lead to mutations.
Nucleotides
Nucleotides are the building blocks of DNA. Each nucleotide consists of three components: a phosphate group, a sugar molecule, and a nitrogenous base. This structural simplicity is its core advantage. The four types of nucleotides—adenine, thymine, cytosine, and guanine—combine in various sequences to form genes, contributing precisely to the overall genetic coding system. However, while nucleotides are vital for DNA structure, they are susceptible to damage from environmental factors, which can compromise genetic integrity.
Base pairs
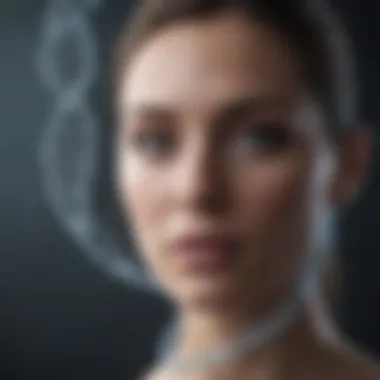
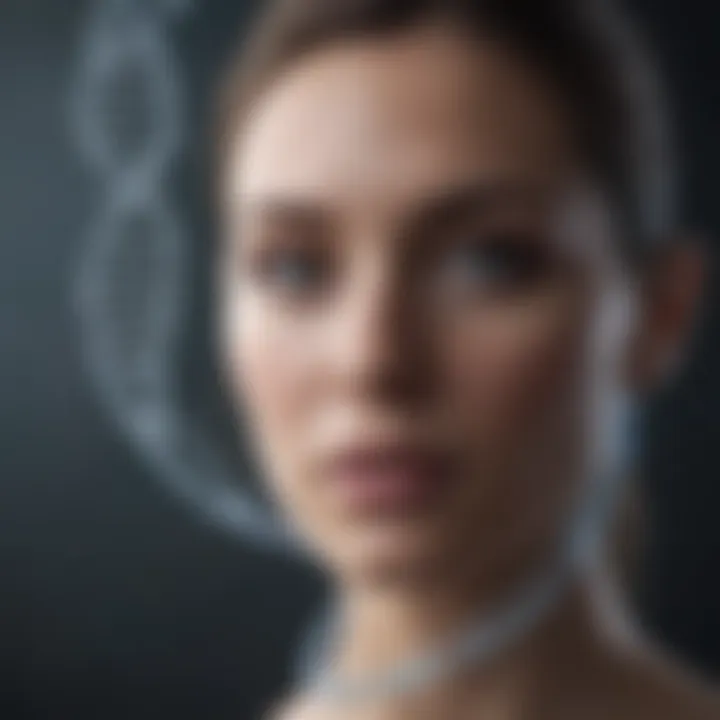
Base pairs form the rungs of the DNA ladder, connecting the two strands of the double helix. The specific pairing of adenine with thymine and cytosine with guanine is essential for maintaining the stability and integrity of genetic information. This characteristic is beneficial for evolutionary processes, as it allows for genetic variability while preserving essential functions. Yet, their specificity can also limit adaptability if environmental changes require swift genetic shifts.
Double Helix Formation
The double helix formation represents one of the most significant discoveries in molecular biology. It illustrates how two strands of DNA twist around each other, with the base pairs positioned in the center. This structure not only protects the genetic code but also facilitates easy access for replication and transcription processes. The design of the double helix is energy efficient, helping to minimize the space needed to store DNA within the cell. Such structural considerations reflect the elegance of biological systems and their efficiency.
Understanding Genetic Codes
Understanding genetic codes is crucial for anyone exploring the field of genetics. This section delves into fundamental concepts that are essential for interpreting DNA sequences. Genetic codes serve as blueprints for biological organisms, dictating the production of proteins that carry out various functions within cells. A thorough understanding of genetic codes allows researchers and practitioners to comprehend how traits are inherited and expressed, which has implications in medicine, ecology, and biotechnology.
What is a Genome?
A genome encompasses the entire set of genetic material in an organism. It contains all the instructions necessary for growth, development, and function. The studying of genomes, therefore, provides insights into the unique traits of species, as well as the shared characteristics that underscore the concept of life itself. The sequencing of genomes has proven vital for many scientific advancements, including personalized medicine and understanding genetic diseases.
The human genome, for instance, consists of approximately 3 billion base pairs of DNA. Each organism has its distinct genome, which can vary significantly between species. Understanding an organism's genome is important for identifying specific genes that are responsible for health and disease, paving the way for targeted therapies and interventions.
Codons and Amino Acids
Codons are triplet sequences of nucleotides in DNA that dictate which amino acids are added during protein synthesis. Each set of three nucleotides corresponds to a specific amino acid or a stop signal in the process of translation. This relationship between codons and amino acids underlines the genetic code's universality across living organisms.
Translation process
The translation process is where the genetic code is converted into the language of proteins. It occurs in the ribosome, where messenger RNA (mRNA) is read, and corresponding amino acids are linked to form a protein chain. This process is vital because proteins are key players in nearly every biological function, serving as enzymes, structural components, and signaling molecules.
One key characteristic of the translation process is its precision. Each codon binding to its respective amino acid ensures that proteins are produced accurately. The benefits of understanding this process are immense. Researchers can manipulate translation to alter protein synthesis, leading to advancements in therapeutics targeting specific diseases. However, translation also has complexities that can lead to errors in protein synthesis, impacting cellular function.
Significance of codon sequences
The significance of codon sequences lies in their role as the framework for protein synthesis. This relationship influences how genetic information is expressed in phenotypes. Codon sequences can carry variations that result in differences in protein function and, consequently, the traits exhibited by organisms.
The key aspect of codon significance is its impact on genetic diversity. A single change in a codon can have profound effects on an organism’s characteristics. Understanding these sequences allows scientists to trace hereditary properties and to explore evolutionary relationships between species. Additionally, a clear grasp of codon implications is beneficial for genetic engineering efforts, where precise modifications can yield desired outcomes in crops or therapeutic agents.
Understanding codons is critical for shedding light on the complex mechanisms of heredity and diversity in the biological world.
Methods for Reading DNA
Understanding the methods for reading DNA is crucial for interpreting the genetic information encoded within. This section explores the techniques that enable researchers to decode DNA sequences, providing insights into biological processes and facilitating advancements in various scientific fields.
Sequencing Technologies
Methods of sequencing DNA allow for the determination of its exact nucleotide sequence, which is fundamental for genetic analysis. Two prominent sequencing technologies are Sanger sequencing and Next-generation sequencing.
Sanger sequencing
Sanger sequencing, developed by Frederick Sanger in the 1970s, has been a cornerstone of genetic research. This method is based on the incorporation of labeled dideoxynucleotides during DNA synthesis, causing termination of the DNA strand. One of its key characteristics is accuracy, making it reliable for smaller sequences.
This method is beneficial for projects requiring high precision, such as determining mutations in a given gene. Its unique feature includes the ability to sequence directly from an existing DNA template. However, Sanger sequencing can be time-consuming and might be cost-inefficient for large-scale genomic projects.
Next-generation sequencing
In contrast, Next-generation sequencing (NGS) revolutionized genomics by enabling massively parallel sequencing. This technique allows millions of fragments to be sequenced at once, drastically reducing the time required for genome sequencing. A vital characteristic of NGS is its throughput, making it suitable for large genomic studies.
NGS's unique feature lies in its ability to produce a vast amount of data quickly and at a lower cost compared to Sanger sequencing. Though it offers numerous advantages, it does come with challenges, such as data analysis complexity and relatively lower accuracy for individual readings compared to Sanger sequencing.
Bioinformatics Approaches
Bioinformatics is necessary to manage and analyze the massive datasets generated by sequencing technologies. Key components of these approaches include various data analysis tools and genome mapping techniques.
Data analysis tools
Data analysis tools play a pivotal role in interpreting sequencing data. These programs help researchers extract meaningful information from the raw genomic information. Their remarkable characteristic is their ability to handle diverse data types and formats, making them essential for genomic research.
These tools are valuable because they streamline the process of variant calling, allowing researchers to identify potential genetic anomalies easily. One unique feature is the integration of machine learning algorithms that enhance predictive capabilities. However, they may require a steep learning curve and extensive computational resources, which can be barriers to usage for some researchers.
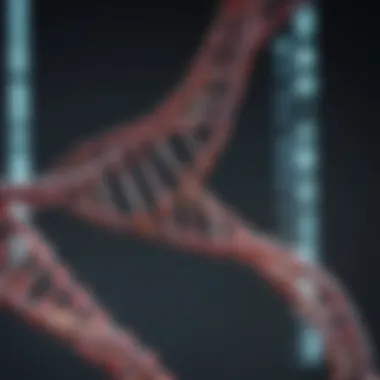
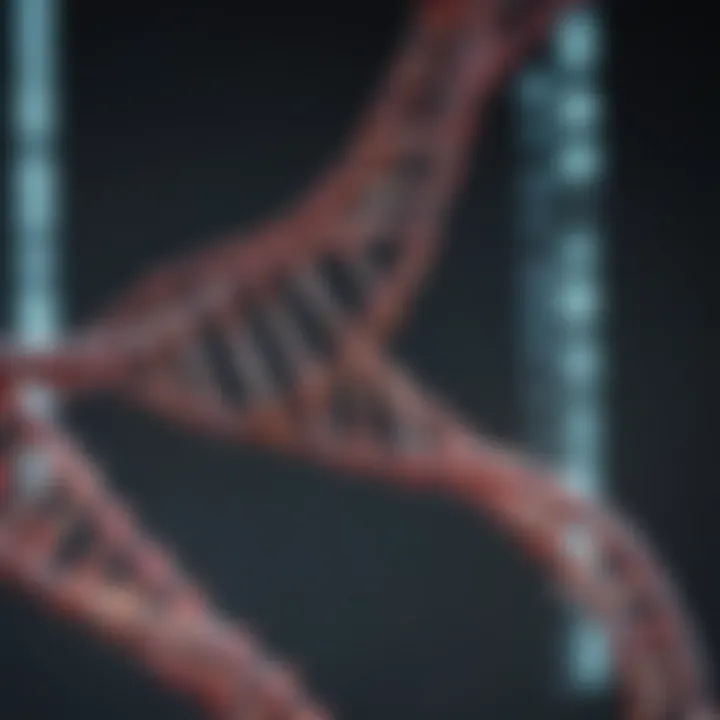
Genome mapping techniques
Genome mapping techniques involve locating genes within a genome and defining their relations. These methods help in constructing genetic maps that are crucial for genetic studies. A distinct aspect of these techniques is their ability to provide a spatial context for genetic data.
These mapping methods are beneficial as they facilitate the understanding of genetic architectures and pathways in organisms. One unique feature includes the use of linkage mapping and physical mapping. Despite their advantages, it is important to note that these techniques can be resource-intensive and may involve complex experimental design.
"Genome mapping is akin to creating a detailed road map that guides researchers through the complexities of genetic relationships."
In summary, the methods for reading DNA are not just technicalities but form the basis of contemporary genetics. They enable researchers to unlock the secrets encoded in our genomes, paving the way for innovations in medicine, agriculture, and many other fields.
Interpreting Genetic Information
Interpreting genetic information is a crucial element in understanding DNA analysis. This process allows scientists and researchers to extract meaningful insights from the complex sequences of nucleotides within the DNA. By addressing the significance of functional genomics and genetic variation analysis, we can grasp how genetic information shapes biological functions and contributes to individual differences in organisms.
Functional Genomics
Functional genomics aims to understand the roles of genes in cellular processes. This field examines how genes interact with each other and how environmental factors influence gene expression. The insights gained from functional genomics help researchers comprehend not only the underlying mechanisms of diseases but also the potential for targeted therapies. For example, identifying gene functions can lead to the development of drugs that specifically target mutated or malfunctioning genes.
Moreover, functional genomics helps predict how organisms respond to various treatments based on their genetic profile, thus enhancing personalized medicine approaches. While powerful, this area of research also raises questions about ethical implications, particularly when it comes to genetic testing and data usage.
Genetic Variation Analysis
Analyzing genetic variation is essential for understanding evolutionary biology and individual traits. There are two main types: single nucleotide polymorphisms and structural variations.
Single nucleotide polymorphisms
Single nucleotide polymorphisms, or SNPs, are variations at a single position in a DNA sequence among individuals. They are the most common type of genetic variation found in humans.
These variations contribute significantly to individual diversity, affecting everything from susceptibility to diseases to responses to medications. The key characteristic of SNPs is their abundance; their widespread presence makes them easier to study and more reliable for genetic association studies.
Since far more SNPs can be identified compared to other types of variations, they are a beneficial choice for many researchers aiming to map genes associated with complex traits. However, analyzing SNPs may have its limitations, particularly in complex diseases where multiple SNPs interact in unpredictable ways.
Structural variations
Structural variations refer to larger genomic alterations, including duplications, deletions, and rearrangements of large DNA segments. These variations can have profound effects on health, as they may disrupt gene function or lead to the formation of oncogenes, which are implicated in cancer development.
The key characteristic of structural variations lies in their potential impact on large regions of the genome, making them critical for understanding genetic diseases. Their ability to encompass multiple genes provides a unique perspective compared to SNPs, offering valuable insights into how changes can lead to phenotypic diversity. In this article, structural variations highlight the necessity for comprehensive genomic analyses, as they can be more challenging to detect and interpret.
"Understanding genetic information requires a careful interpretation of both functional genomics and genetic variation analysis, aligning with advancements in technology."
Ultimately, each of these aspects contributes uniquely to the broader goal of interpreting genetic information. Together, they form the foundation upon which we build our understanding of genetics, ensuring that scientists can more effectively investigate the complexities of life.
Applications of DNA Reading
The applications of DNA reading extend far beyond the realm of theoretical science. They present tangible solutions and insights across various fields. Understanding how we utilize DNA analysis is crucial, given its impact on medicine, forensics, and agriculture. This section outlines the key areas where DNA reading has significant implications, benefits, and necessary considerations.
Medical Diagnostics
DNA reading plays an essential role in medical diagnostics. By examining genetic information, healthcare professionals can identify predispositions to certain diseases. This identification process can lead to early interventions, personalized treatment plans, and more effective management of chronic conditions. Advancements in whole-genome sequencing have made it possible to uncover genetic variations linked to diseases such as cancer, cardiovascular conditions, and rare genetic disorders.
For example, identifying single nucleotide polymorphisms can help predict patient responses to medications. The field of pharmacogenomics is therefore rapidly evolving, allowing clinicians to tailor treatments based on the patient’s genetic makeup. This ultimately enhances treatment efficacy and minimizes adverse drug reactions.
Additionally, DNA analysis furthers research in hereditary diseases, enabling families to make informed health decisions.
Forensic Science
In forensic science, DNA reading is invaluable for criminal investigations. The ability to analyze genetic material found at crime scenes allows for the identification of suspects and victims. Forensic DNA profiling has led to numerous convictions and exonerations, highlighting its necessity in the justice system.
Techniques such as short tandem repeat (STR) analysis enable forensic scientists to compare DNA samples with great precision. Law enforcement agencies increasingly rely on DNA databases—for both solving existing cases and preventing future crimes. This helps to connect seemingly unrelated cases and establish patterns of criminal behavior.
There are ethical implications with DNA use in forensics, particularly concerning privacy and the potential for misuse of genetic information. However, the potential for ensuring justice remains a strong argument for DNA's use in this field.
Agricultural Biotechnology
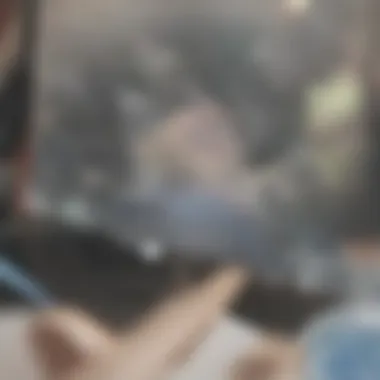
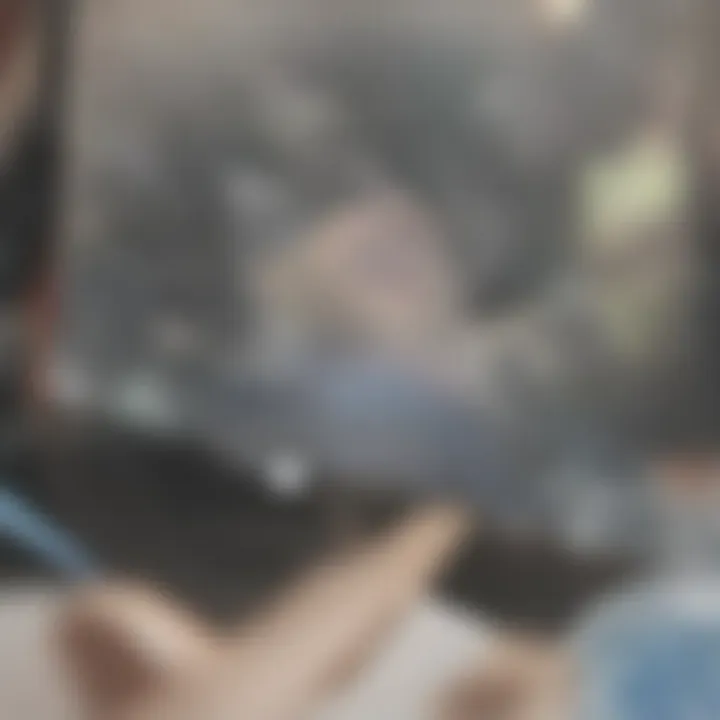
The agricultural sector also benefits from DNA reading, particularly in biotechnology. Genetic analysis aids in the development of genetically modified organisms (GMOs) that are more resistant to pests, diseases, and environmental stress. By manipulating specific genes, scientists can enhance crop yield and nutritional content, addressing food security challenges.
Furthermore, DNA reading paves the way for accurate plant breeding. With knowledge of genetic markers, breeders can select desirable traits more effectively, speeding up the crop development process. This benefits both farmers and consumers alike, providing healthier and more sustainable agricultural practices.
"DNA applications in agriculture not only promise improved crop performance but also offer environmental benefits by reducing pesticide use."
Ethical Considerations
The discussion surrounding the ethics of DNA reading is increasingly significant, especially as advances in genetic technology continue to evolve. Ethical considerations encompass a wide array of issues that arise from the ability to analyze, modify, and understand genetic information. These considerations are crucial not only for researchers but also for the broader community affected by genetic research and applications. They help guide responsible practices and protect individual rights while maximizing the benefits that such technological breakthroughs can offer.
Privacy Concerns
Privacy is one of the foremost ethical considerations in the realm of DNA research. The ability to read and interpret genetic code brings with it the potential for significant invasions of personal privacy. Genetic information is intrinsically sensitive, often holding detailed insights into an individual's health, ancestry, and identity. Thus, with advancements in DNA analysis, there arises a pressing need to protect this information.
Individuals may have differing perspectives on how their data should be handled. Some may advocate for full disclosure, seeing the benefits of sharing their genetic information for research and medical advancements. Others may fear the misuse of their data. Genetic data can be vulnerable to breaches, and the potential for discrimination based on genetic predispositions adds layers of concern to the discourse.
Here are key points regarding privacy concerns related to genetic data:
- Informed Consent: Individuals must be made aware of how their genetic data will be used and have the option to consent.
- Data Security: Protecting genetic data from unauthorized access and breaches is paramount.
- Disclosure Policies: Clear guidelines must be established regarding who has access to genetic information and under what conditions.
"Privacy is not just about data; it is about the dignity of individuals and their right to control their own genetic narrative."
Implications of Genetic Editing
Genetic editing represents both a powerful tool for advancing science and a source of ethical dilemmas. Technologies like CRISPR-Cas9 have opened new frontiers in gene alteration, allowing for precise modifications in the DNA sequence. This capability raises profound ethical questions about the nature of genetic intervention.
While genetic editing holds promise for correcting genetic disorders and improving agricultural yields, it also invites caution. The potential for unintended consequences, such as off-target mutations, calls for rigorous ethical scrutiny. Here are some important implications:
- Designer Genes: The possibility of creating "designer babies" raises moral questions about accessibility and the definition of normality.
- Ecosystem Impact: Genetic modifications in organisms can influence ecosystems in unpredictable ways, possibly leading to ecological imbalances.
- Equity and Access: How these technologies are distributed could exacerbate existing inequalities in health care and technology access.
In summary, as we advance in our ability to read and edit DNA, it is critical to engage in ethical considerations. Addressing privacy concerns and the implications of genetic editing ensures that scientific progress does not infringe upon individual rights or societal values.
Future Directions in DNA Research
The field of DNA research is perpetually evolving, driven by advances in technology, a growing understanding of genetics, and the application of innovative methodologies. Emphasizing future directions in this area is vital for various reasons. Understanding these trends allows researchers and students to grasp what approaches might dominate the field, shaping both laboratory practices and theoretical knowledge. Moreover, this awareness enables them to contribute effectively to ongoing discussions and explorations in genomics and molecular biology.
Emerging Technologies
Emerging technologies play a pivotal role in the future of DNA research. Several advancements are set to revolutionize how scientists analyze genetic material. Significant developments include:
- CRISPR-Cas9: This gene editing tool allows for precise modifications of the DNA sequence. Its potential application in treating genetic disorders continues to expand. As researchers develop safer and more effective versions of this technology, its implications for medicine and agriculture are profound.
- Third-generation sequencing: Unlike traditional methods, this technology enables real-time sequencing and the analysis of longer DNA fragments. Consequently, it enhances the accuracy of genomic data, which is crucial for understanding complex genetic traits.
- Nanopore sequencing: Another remarkable advancement is the development of nanopore sequencing, which allows DNA to be sequenced as it passes through a tiny pore. This method offers speed and portability, vital for large-scale genomic studies.
In addition to these specific technologies, there is a movement toward integrating machine learning and artificial intelligence within DNA research. This integration aids in processing vast amounts of genetic data—enabling more precise predictions and insights into genetic diseases.
Trends in Computational Biology
As DNA analysis becomes more sophisticated, the role of computational biology is increasingly significant. Trends in this area reveal a growing reliance on computational methods for interpreting genetic data. Key aspects include:
- Data analysis: With high-throughput sequencing, vast datasets are generated. Computational methods are necessary for organizing, analyzing, and deriving meaningful insights from this data. For instance, bioinformatics tools are essential for identifying patterns and variations that correlate with phenotypic traits.
- Genome mapping: Advanced computational techniques simplify the process of mapping genomes. These methods facilitate the identification of genetic markers linked to diseases. In turn, this understanding can lead to personalized medicine approaches where treatments are tailored based on an individual’s genetic profile.
- Predictive modeling: Researchers increasingly use computational models to predict how genetic variations influence biological processes. This can assist in drug development and the understanding of disease mechanisms.
Adapting to these trends requires robust training in both biological sciences and computational techniques. Educational programs are evolving to meet this need, preparing future generations of researchers to harness technology effectively in DNA research.
"The integration of computational biology and advanced technologies is vital for modeling complex biological interactions that define life itself."
Epilogue
The conclusion of this article underscores the significance of understanding how to read DNA code. This understanding is crucial as it encapsulates the essence of genetic information, influencing various fields such as medicine, forensics, and agriculture. Readers should recognize that delving into DNA analysis not only expands scientific knowledge but also raises essential discussions around ethics and privacy.
Here are some key reasons why this conclusion matters:
- Integration of Knowledge: It ties together various concepts covered in the article, enabling readers to see the full picture of DNA analysis.
- Practical Applications: The information provided underscores real-world applications, showing how these techniques can lead to advancements in treatments for diseases or improvements in crop yields.
- Ethical Considerations: This section also highlights the importance of addressing ethical concerns raised by advances in genetic technology, ensuring the responsible conduct of research.
Understanding DNA is not only about the science; it is also about our responsibilities as stewards of this knowledge.
Ultimately, the insights offered here empower students, researchers, educators, and professionals to navigate the complexities of genetic data responsibly. It is essential that ongoing research continues to be conducted with transparency, aiming for a balance between innovation and ethical considerations.
Summary of Key Points
- The article provides a comprehensive overview of DNA structure and function.
- It explores sequencing technologies and bioinformatics approaches that facilitate DNA reading.
- Applications of DNA analysis are diverse, impacting health, law, and agriculture significantly.
- Ethical concerns are crucial as genetic technologies evolve, requiring careful consideration and dialogue.
Readers are encouraged to stay informed and engage critically with developments in DNA research.