Mastering CRISPR-Cas9 Primer Design Techniques
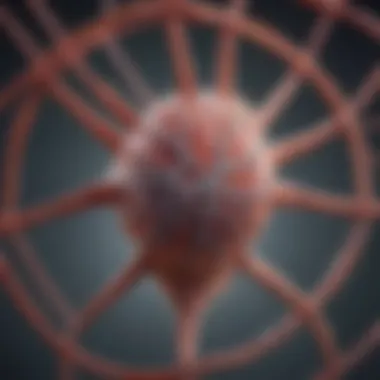
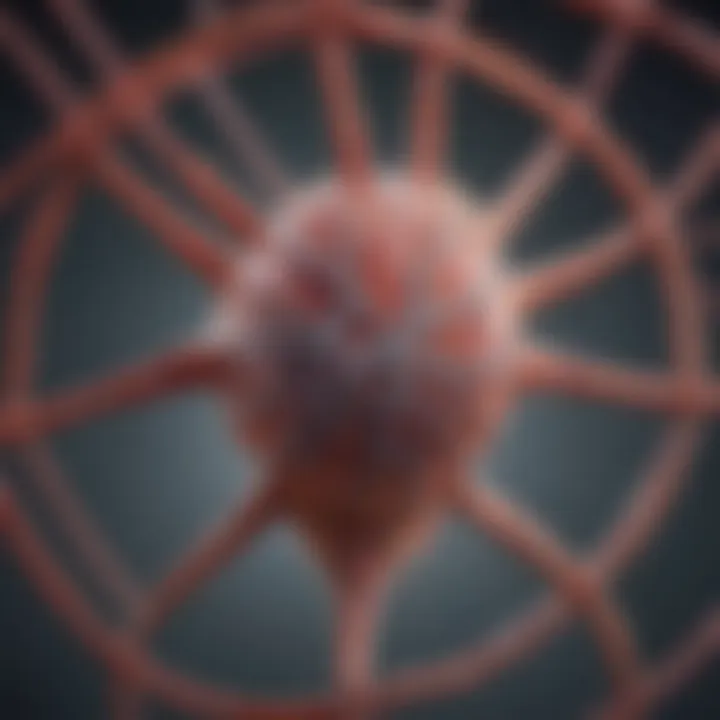
Intro
CRISPR-Cas9 technology has taken the research world by storm, and one of its pivotal components is primer design. For those diving into gene editing, understanding the nuances of primers can mean the difference between a successful experiment and a whole lot of wasted time. This guide is crafted for students, researchers, educators, and professionals with a keen interest in molecular biology and genomics. Whether you're dabbling in genetic modifications or looking to heal hereditary diseases, the primer design process is a critical step that needs attention.
By breaking down the specific steps involved in designing effective primers for CRISPR-Cas9 applications, this article provides a comprehensive overview that caters to both novice and seasoned practitioners. From grasping the theoretical foundations of CRISPR technology to honing in on practical tips for efficiency and specificity, we’ll cover essential strategies for ensuring that researchers enter the laboratory equipped with robust designs that contribute to precise gene editing efforts.
Let’s delve deeper.
Key Findings
Primer design is an often-overlooked aspect of CRISPR experiments that carries significant weight in the overall success of gene editing. Here are the primary points to consider:
- Primer specificity is pivotal: The ability of primers to match target sequences without binding to non-target areas significantly affects the efficiency of off-target effects and overall results.
- Length matters: The optimal length for primers typically falls between 18 to 25 nucleotides, balancing between specificity and binding affinity.
- GC content influences stability: Aim for a GC content of 40-60% to achieve the right balance of melting temperature (Tm) and stability; too high or too low can lead to undesired binding characteristics.
"Effective primer design is like the cornerstone of a solid foundation; without it, even the most advanced CRISPR techniques might crumble under scrutiny."
Implications of the Research
The implications of thoughtful primer design stretch far beyond the lab. Here’s how it can impact real-world applications:
- Gene therapies and biotechnology: Advanced understanding of primer design can enhance therapeutic interventions that target genetic disorders, thereby improving patient outcomes.
- Agricultural advances: Precision in gene editing has vast potential in crop improvement, providing traits like disease resistance or enhanced nutritional profiles for food security.
- Future research: As CRISPR techniques evolve, so does the need for more refined primer design protocols. Investments in this area can lead to breakthroughs in understanding gene functions and the development of sophisticated genetic engineering tools.
With this foundation laid, let’s explore every aspect involved in CRISPR-Cas9 primer design, enriching your approach to gene editing endeavors.
Preface to CRISPR-Cas9 Technology
CRISPR-Cas9 technology stands as a landmark in gene editing, representing a breakthrough that has reshaped the life sciences landscape. Its significance cannot be overstated, as it provides not just a method for precise genetic modifications but opens a Pandora’s box of possibilities in medicine, agriculture, and beyond. By understanding the ins and outs of CRISPR-Cas9, researchers and practitioners can harness its power to address complex biological questions and tackle genetic disorders.
The CRISPR-Cas9 system derived from the adaptive immune system of bacteria. This technology allows scientists to target specific DNA sequences with unprecedented accuracy, leading to developments that previously existed only within the realm of science fiction. But this is no mere techno-babble; the implications for genetic engineering, including the potential to treat genetic diseases and enhance crop resilience, are staggering.
In this section, we will explore key historical milestones and fundamental mechanisms that underpin the CRISPR-Cas9 technology. Each aspect builds upon the other, painting a complete picture of what this technology offers and the responsibilities it brings along.
Historical Context
The journey of CRISPR technology began in the late 1980s when researchers stumbled upon unusual sequences in the DNA of bacteria. Initially, it was treated as an oddity until in 2005, the function of these sequences was deciphered. Scientists identified them as part of a bacterial immune system that protects against phages. Fast forward to 2012, and Jennifer Doudna and Emmanuelle Charpentier successfully adapted this system into a tool for gene editing. This marked a watershed moment.
Now, scientists from various fields began experimenting with the tool, leading to swift advancements. Notably, a plethora of research has been published since then, ranging from genetically modified organisms to clinical trials aimed at eradicating hereditary diseases. This rapid development demonstrates how important CRISPR-Cas9 is to modern biology and the research community. Here, innovation is the coin of the realm.
Mechanism of Action
At its core, the CRISPR-Cas9 system operates with remarkable efficiency. It employs two key components: the CRISPR RNA (crRNA) that guides the Cas9 protein to the target DNA and the Cas9 enzyme that acts as molecular scissors to cut the DNA at a precise location. This dual-action system allows for targeted editing by either inserting or deleting specific genetic sequences.
Here's how it goes down in simpler terms:
- Guide RNA Design: The user designs a piece of RNA that matches the sequence of the target DNA. This is critical, as the effectiveness of editing hinges on the accuracy of this sequence.
- Binding: Once introduced into the cell, the guide RNA binds to the complementary DNA sequence, latching on like a savvy lock and key.
- DNA Cutting: The Cas9 enzyme comes into play, cutting the DNA at the specified location. This double-strand break triggers natural repair mechanisms in cells, which can lead to the desired genetic modifications.
The beauty of this mechanism is its versatility. Researchers can induce various changes, such as knocking out genes or inserting new genetic material. Nevertheless, such powerful tools also demand careful handling to avoid unintended consequences.
In summary, understanding the historical context and mechanism of action for CRISPR-Cas9 technology lays the groundwork for effective primer design, an essential aspect covered in the subsequent sections of this article.
Understanding Primer Design
Understanding primer design is a foundational aspect of CRISPR-Cas9 technology. It may seem straightforward at first glance but the details are crucial to ensuring successful editing. Think of primers as the artists' brushes; without the right tool, the masterpiece becomes a mere doodle.
Primers are short sequences of nucleotides that bind to a specific DNA template, guiding the enzymatic processes necessary for gene editing. By crafting precise primers, researchers can lead the CRISPR machinery to the exact location on the genome where changes are desired. This specificity is not just a bonus, but a necessity; off-target modifications can lead to unintended consequences.
Here are some key reasons why understanding primer design is vital:
- Specificity: A well-designed primer will only bind to the intended target site, minimizing off-target activity. This is where it can mean the difference between a successful experiment and a costly mistake.
- Efficiency: Higher efficiency in primer design can enhance the overall outcome of gene editing projects. Subpar primers can lead to decreased gene editing efficacy, wasting time and resources.
- Versatility: Different applications, whether in medicine or agriculture, require tailored approaches to primer design. Grasping these nuances allows for adaptable strategies across diverse fields.
Like a seasoned chef who knows which spices to blend for an exquisite dish, researchers who master primer design can produce remarkable results with CRISPR-Cas9 technology. Hence, focusing on this aspect can elevate one's understanding and effectiveness in gene editing.

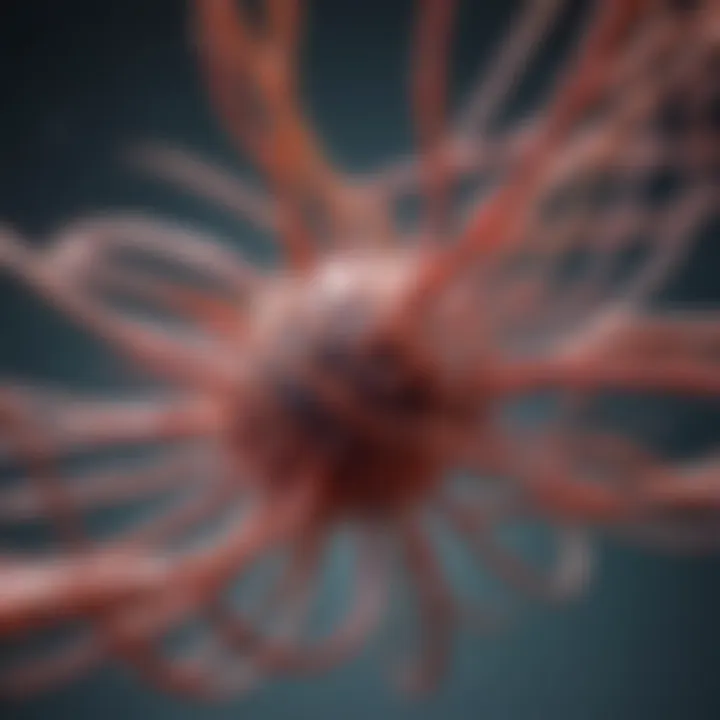
The Role of Primers in CRISPR
Primers play a pivotal role in the functionality of the CRISPR-Cas9 system. They support the entire procedure by providing the necessary sequence context that the Cas9 protein requires. Cas9, the molecular scissors of this technology, relies on these short sequences to locate its target efficiently. Once a suitable primer binds to the DNA, the Cas9 enzyme can then slip into action and create a double-strand break.
Moreover, primers also influence the efficiency of homologous recombination, which is a critical mechanism that repairs DNA breaks. Through the repair process, the desired genetic changes are introduced. If the primers are poorly designed, the chances of successful recombination drop significantly, possibly leaving the desired edits on the cutting room floor.
Types of Primers for CRISPR
When it comes to CRISPR primer design, it’s essential to understand the different types available. Each serves specific functions and applications:
- Forward Primers: These initiate the synthesis of new DNA strands during PCR or other amplification techniques.
- Reverse Primers: Typically paired with forward primers, they help create the double-stranded DNA needed for editing.
- Universal Primers: They are designed to anneal with multiple sequences; useful when working in a context requiring flexibility.
- Nested Primers: A pair of primers designed to bind within the product of a previous PCR round, increasing specificity and sensitivity.
In summary, combining various primer types judiciously can lead to enhanced detection and modification capabilities in CRISPR applications. Like choosing the right tools for a task, selecting the correct primer types can dramatically influence outcomes.
Considerations for Effective Primer Design
When it comes to CRISPR-Cas9 technology, effective primer design is like laying a solid foundation for a skyscraper. Without it, the whole structure can come tumbling down. The aim here isn’t just to throw together a couple of sequences and call it a day; it requires a methodical approach that takes several intricate factors into account.
Understanding the nuances of primer design can drastically influence the outcome of gene editing experiments. Not only does the design affect specificity in targeting genes, but it also has implications for the overall efficiency of the CRISPR process. By paying close attention to certain considerations, researchers can enhance the likelihood of successful edits, thus making their efforts far more productive.
Target Region Selection
Selecting the right target region might feel like searching for a needle in a haystack. However, it is crucial for success. The target site on the genome must be accessible and present a favorable environment for CRISPR components to function.
For instance, choosing a region rich in accessible DNA sequences can improve binding efficiency. Traditionally, researchers often consider selecting exons over introns, as exons code for proteins which can sometimes yield clearer results in downstream analysis. Furthermore, it's essential to verify that the target region is unique across the genome to avoid unwanted off-target effects. This means consulting various databases for homology searches and utilizing tools like BLAST for alignment checks.
Keeping these factors in mind will pave the way for a smooth editing process.
Length and Sequence Composition
When it comes to a primer's length and sequence composition, there’s no one-size-fits-all answer. Generally, primers should be between 18 to 25 nucleotides. This range strikes a balance between specificity and binding strength. Too short may lead to non-specific bindings, while too long could complicate synthesis and annealing.
The composition of the primer’s bases is equally important. A good rule of thumb is to have a balanced mix of GC and AT percentages. A higher GC content can increase stability, but too high a percentage can lead to secondary structures like hairpins, which are generally the last thing you want. Aim for a composition that avoids repetitive sequences or regions with high homology to other genes, thus minimizing the risk of off-target activity.
Key Considerations for Sequence Composition:
- Avoid homopolymeric runs: Sequences with a stretch of identical bases can lead to mispairings.
- Using restriction sites: If integrating with vectors, consider including restriction enzyme sites at the ends.
- Consider the placement: A good primer often has a G or C at the 3’ end to increase binding stability.
Melting Temperature Calculations
The melting temperature (Tm) is pivotal in primer design. It’s essentially the temperature at which half of the DNA is in the double-stranded form and half is in the single-stranded form. If the Tm is too low, the primer may not bind effectively; too high, and it might lead to issues during the PCR amplification stages.
To get a handle on these calculations, you can use formulas, online calculators, or software tools specifically tailored for primer design.
Tm can be estimated by:
- Adding 2°C for each A or T in the primer
- Adding 4°C for each G or C
By examining the Tm closely enough, you can ensure that the primers work in harmony with one another if you're using multiple sets, keeping you clear of complications in the lab work.
In closing, focusing on these core considerations allows researchers to tailor their primer design to their specific needs, aiding in the accurate and effective application of CRISPR technology. This careful planning ultimately creates a ripple effect that enhances overall research productivity and efficacy.
Specificity and Off-Target Effects
In the realm of CRISPR-Cas9 applications, ensuring specificity is non-negotiable. This ensures that the gene-editing tool only targets the desired sequences within the genome, which is paramount for successful outcomes. The implications of off-target effects can be profound. If the editing tool mistakenly modifies unintended regions of the genome, the implications can range from harmless to severely deleterious. Given that gene editing holds the potential for groundbreaking advances in treatment and agriculture, the stakes couldn’t be higher.
Importance of Specificity
Specificity serves as the bedrock of any CRISPR experimentation. When designing a guide RNA, the primary goal is to ensure it binds exclusively to the target site. Here are a few reasons why specificity can't be overlooked:
- Precision: Targeting the right gene means better outcomes, especially in therapeutic applications.
- Safety: Minimizing unintended alterations guards against potential side effects, such as activation of oncogenes or silencing of crucial genes.
- Efficacy: High specificity increases the chances of the desired edit taking place while limiting unwanted modifications, thereby enhancing overall success rates.

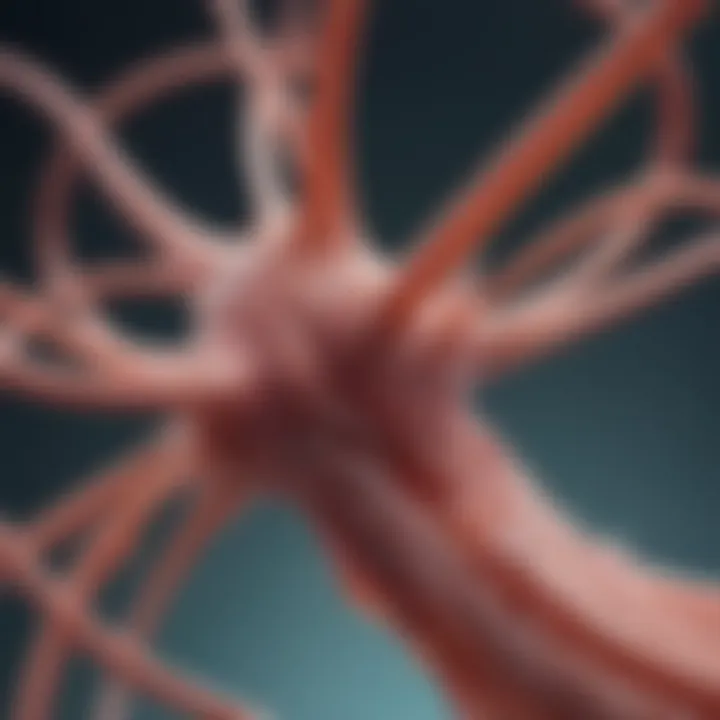
Studies have shown that off-target activity tends to correlate with the homology of the guide RNA to unintended sites. If the guide RNA differs from the target sequence by as little as one nucleotide, it could lead to unintentional editing. This sensitive balance calls for meticulous attention during design phases.
Strategies to Minimize Off-Targets
Preventing off-target effects requires strategic planning and execution. Here are several tactics that researchers can apply:
- Use of High-Fidelity Cas9 Variants: Using engineered variants of Cas9, such as SpCas9-HF1 or eSpCas9, can contribute to reduced off-target activity.
- Designing Optimal Guide RNA: In-depth analysis for the selection of guide RNAs can significantly help. Software tools that evaluate potential off-target sites during design phases can streamline this process.
- Shortening the Length of Guide RNAs: Shorter guide RNAs can reduce the risk of off-target binding, although this approach can come with trade-offs regarding binding efficiency.
- Confirming Off-Target Effects through Sequencing: Post-editing, employing high-throughput sequencing techniques can help in identifying any off-target sites that may have been altered inadvertently.
- Focus on Target-Dedicated Delivery Methods: Carefully selecting the method of delivery for the CRISPR components can ensure a more localized action thus preventing unintended genomic interactions.
Engaging in rigorous pre-design analysis and adopting the newest technologies aids significantly in minimizing off-target effects.
The complexity and precision required in the design of CRISPR-Cas9 experiments underscore the necessity for researchers to remain vigilant about specificity to create reliable and effective outcomes. By addressing off-target effects head-on, one can not only enhance the integrity of each gene-editing action but also pave the way for safer applications in biomedicine and agriculture.
Tools and Software for Primer Design
Primer design is critical when utilizing CRISPR-Cas9 technology, and the right tools can make or break a project. Advanced software and an arsenal of online tools simplify the often-complex process of primer creation, optimizing the chances of successful gene editing. With a multitude of options available, understanding the various tools can enhance primer specificity, minimize off-target effects, and ultimately contribute to more precise outcomes in gene editing applications.
Overview of Available Tools
Numerous tools exist to aid in designing primers specifically tailored for CRISPR-Cas9 experiments. Popular ones include:
- Primer3: A widely used design tool that allows customization of primers based on various parameters such as length and GC content. This flexibility makes it suitable for a variety of applications.
- CRISPRdirect: Geared specifically for CRISPR, it designs sgRNA and recommends primers while also allowing researchers to check for off-target sites.
- Benchling: Offering a suite of tools, Benchling simplifies the entire molecular biology process from primer design to experimental documentation.
- eCRISP: An online platform that helps in designing sgRNA sequences along with primers, making off-target assessments equally straightforward.
Each tool has its unique benefits, and the choice may depend largely on specific project needs, interface preferences, and integration capabilities with other bioinformatic tools.
Comparison of User Interfaces
User interface plays a pivotal role in choosing the right tool for primer design. A well-designed interface can streamline the workflow, making the process of primer design more efficient. Here’s a brief comparison:
- User-Friendliness: Tools like Benchling provide an intuitive layout, allowing users to quickly navigate through options without a steep learning curve. Conversely, some tools can be packed with features that might overwhelm new users.
- Feature Accessibility: In CRISPRdirect, users can easily input gene sequences and receive immediate feedback, whereas other tools may require a deeper understanding of the program before realizing their full potential.
- Output Clarity: Clear, well-organized outputs are vital. Primer3 excels by providing structured feedback on primer characteristics, while eCRISP also includes off-target information but may present it in a more complex format.
"The right tool not only simplifies the primer design process but also enhances the quality of experimental outcomes."
Case Studies of Tool Utilization
Examining real-world applications of these tools highlights their impact on successful primer design:
- CRISPRdirect in Gene Knockout:
- Benchmarking with Primer3:
- Integrating Benchling for Documentation:
- A research team utilized CRISPRdirect to design primers for a gene knockout project in mice. They reported a significant improvement in specificity, with minimal off-target activity.
- Another group employed Primer3 for designing primers for a synthetic biology project, allowing them to customize primer sequences to fit their requirements, resulting in efficient amplification of desired sequences.
- A study employed Benchling not only for primer design but also for keeping track of experimental results, which aided in the seamless collaboration among researchers, enhancing overall project efficacy.
These case studies underscore the viability of these tools in practical applications, emphasizing their critical role in contemporary CRISPR research. Clearly, choosing the right software can significantly influence the outcome of gene editing projects, and incorporating these tools effectively can lead to more successful endeavors.
Experimental Validation of Primer Efficacy
Experimental validation of primer efficacy is a cornerstone in the field of CRISPR-Cas9 research. This process assesses how well designed primers perform in actual experiments. When working with gene editing applications, having confidence in the effectiveness of primers is vital. It essentially ensures that the intended genetic alterations can be achieved with precision, minimizing the chances of unwanted outcomes. Without proper validation, researchers risk pursuing paths that do not yield the desired effects, ultimately wasting time and resources.
The significance of this validation process spans numerous elements. First and foremost, it allows researchers to confirm the specificity of their primers. This is crucial because non-specific interactions can lead to unwanted off-target effects. By confirming efficacy through experimental testing, one can affirm that the primer binds only to the intended target sequence.
Additionally, validating effects helps in gauging the efficiency of primers in leading to successful edits. It’s not simply about binding; it’s about effectively facilitating the CRISPR-Cas9 mechanism to introduce the desired changes in the genome.
Lastly, experimental validation fosters trust within the scientific community. Publishing validated results increases reproducibility, thus promoting confidence in the findings that can lead to subsequent research developments.
Methods for Testing Primers
There are several methodologies to assess the efficacy of primers in CRISPR applications. Below are a few of the commonly utilized approaches:
- Polymerase Chain Reaction (PCR): This is a fundamental technique for amplifying DNA. By employing PCR with the primers in question, researchers can determine whether the primers can efficiently amplify the target sequences under the conditions tested.
- Sanger Sequencing: After running PCR, Sanger sequencing can be a valuable confirmatory step. It helps in verifying that the amplified products correspond to the intended targets, providing a deeper layer of confidence in the experimental outcomes.
- qPCR (Quantitative PCR): This technique not only confirms that amplification occurred but also quantifies it. It allows for insights into the efficiency of the primer, which is essential for understanding its performance in various experimental conditions.
- Gel Electrophoresis: By analyzing PCR products using gel electrophoresis, researchers can visualize the results. Clear bands indicate successful amplification, while extra bands may hint at non-specific binding or primer-dimer formation, which can impair the intended outcomes.
- CRISPR Activity Assessment: Using methods such as sequencing of edited alleles or functional assays, the actual outcome of the CRISPR-Cas9 system can be evaluated. This helps correlating the primer performance directly with gene editing activity.
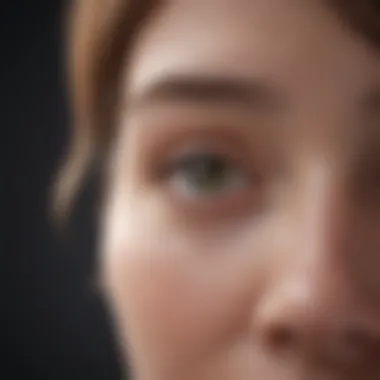
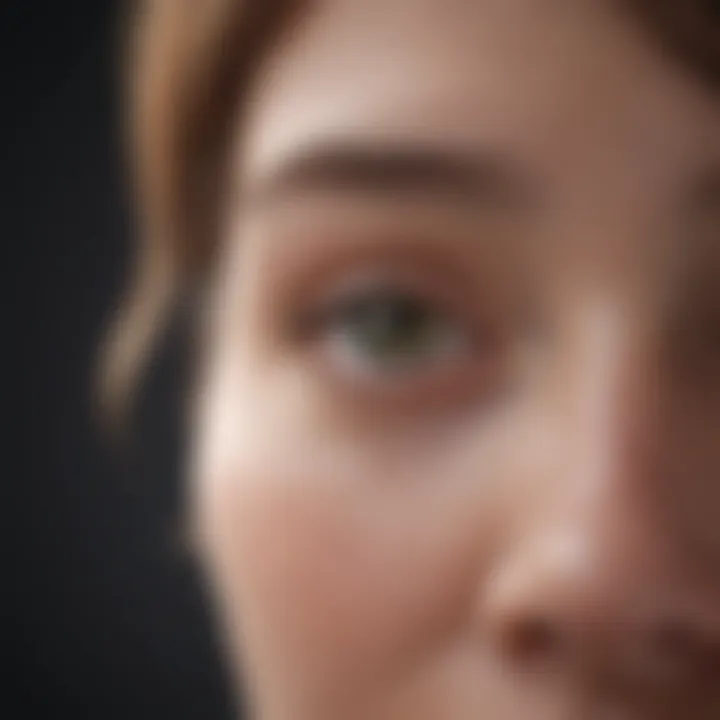
Interpreting Primer Performance
Once the methods for testing primers are employed, the next step involves interpreting their performance. This interpretation is multifaceted and requires careful analysis.
Firstly, the success rate of the amplification must be evaluated. A high success rate typically indicates that the primers are functioning correctly in their designed role. However, if the success rate is low, researchers must investigate further; it may require redesigning the primers or modifying conditions.
Next, the specificity of the amplification must be analyzed. Primers that exhibit non-specific binding can lead to a host of complications, including undesirable off-target edits. It is critical to compare the results obtained from the test against expected outcomes. If unexpected products are generated, one may consider adjusting the primer design or the experimental setup.
Quantitative data from qPCR can also provide insight into how efficiently the primers function. Performance metrics from this data should be compared to thresholds established during initial design stages. This can help in differentiating robust primers from those that struggle under experimental conditions.
Interpreting the outcomes should not disregard assay reproducibility. Consistency across separate experiments underscores the reliability of primer performance. It is often beneficial to conduct multiple replications to solidify findings.
In essence, interpreting primer performance is not merely about observing outcomes but understanding them to improve the design and application of CRISPR technologies.
Through these insights into methods and interpretation, researchers can further their work in a confident and informed manner, ultimately enhancing the CRISPR-Cas9 editing strategies. Proper validation and understanding of primer efficacy lead to successful gene editing efforts, thus paving the way for advancements in fields like medicine and agriculture.
Ethical Considerations in CRISPR Use
The rapid advancements in CRISPR-Cas9 technology have ushered in a new era of possibilities for gene editing. As the scientific community embraces these innovations, it becomes paramount to engage with the accompanying ethical considerations. This discussion is not merely academic; it is essential for guiding responsible research practices and ensuring that the benefits of CRISPR technology do not come at an unintended cost to society.
Central to the ethical landscape surrounding CRISPR are the regulatory frameworks. These frameworks play a critical role in governing how gene editing can be applied. The existence of robust regulations ensures that research does not stray into murky waters. Different countries have established their own set of rules, reflecting their values and societal norms. For instance, in the United States, the Food and Drug Administration (FDA) oversees the use of gene editing for therapeutic purposes, while in the European Union, stricter regulations apply to genetic modifications, emphasizing precaution. These frameworks often reflect a balancing act—facilitating innovation while protecting public welfare.
Conversely, there is an ongoing conversation regarding public perception and ethical debates. Gene editing, particularly when it comes to potentially modifying human embryos, ignites passionate discussions. Opinions vary widely; some view these advancements as a pathway to eradicating genetic disorders, enhancing human capabilities, and even improving agriculture. Others, however, raise alarms about the unforeseen consequences of 'playing God,' the potential for eugenics, or inadvertent ecological disruptions. The apprehension about gene editing technology often stems from a lack of understanding among the general populace, making public education a crucial piece of the puzzle. Addressing these concerns head-on, through open, transparent dialogue, is essential. An informed society is better equipped to discuss the implications, weigh benefits against risks, and engage with the ethical complexities of CRISPR technology.
"The greatest danger in times of turbulence is not the turbulence—it is to act with yesterday's logic." — Peter Drucker
Future Directions and Applications
As the field of gene editing continues to make waves, understanding the future directions and applications of CRISPR technology becomes imperative. Development in this area isn’t just about perfecting techniques; it’s about exploring uncharted territories that could reshape medicine, agriculture, and other sectors. Here, we’ll discuss recent advancements and innovative applications, which not only promise to increase the efficiency and safety of CRISPR-Cas9 but also highlight its vast potential.
Advancements in CRISPR Technology
Recent advancements in CRISPR technology are paving the way for unprecedented capabilities in gene editing. One notable trend is the improvement of Cas proteins. For instance, researchers are continuously working to develop next-generation Cas proteins, like Cas12 and Cas13, which offer unique features such as increased specificity and reduced off-target effects. These proteins also have applications beyond traditional gene editing, including diagnostic tools that can identify viral infections rapidly.
Moreover, the introduction of base-editing technologies allows for more precise modifications without double-strand breaks in DNA. This means correcting point mutations without the potential chaos that full gene edits could bring. Another major focus is the development of delivery methods, like liposome-based systems, which enhance how effectively CRISPR components are delivered inside cells. By improving delivery vehicles, scientists hope to see CRISPR’s therapeutic potential truly realized in a clinical setting, such as in the treatment of genetic disorders.
The importance of these advancements can’t be overstated. They could lead to broader accessibility of gene editing technology and practical solutions to previously unsolvable genetic issues.
Innovative Applications in Medicine and Agriculture
The applications for CRISPR technology are vast. In medicine, possibilities include treating genetic conditions such as sickle cell anemia and cystic fibrosis. A particularly exciting development is CRISPR’s role in CAR-T cell therapy. Researchers are employing CRISPR to modify T cells so they can more effectively attack cancer cells. The flexibility of CRISPR allows for personalizing treatments, tailoring them precisely according to a patient's unique genetic makeup, which could revolutionize cancer care.
In agriculture, CRISPR is being used to develop crops that are resistant to diseases or environmental stress. For example, scientists are creating drought-resistant varieties of crops, which is essential as climate change alters weather patterns unpredictably. This aspect appeals directly to food security concerns, enabling farmers to maintain yields even in less-than-ideal conditions. By cutting down the time traditionally needed for crop breeding, CRISPR technology can lead to faster production of vital food sources.
"The innovative applications of CRISPR not only hold tremendous potential but also invite essential dialogues surrounding ethical implications of gene editing in living organisms."
The blend of advancements in CRISPR technology and its applications in both medicine and agriculture suggests a promising horizon. However, careful consideration of ethical implications is also necessary, especially as society grapples with the realities of gene editing. As these technologies unfold, they could indeed redefine many aspects of our lives, shifting how we understand genetics and interaction with the biological world.
End
The conclusion serves as more than just a wrapping up of the article; it highlights the significance of CRISPR-Cas9 primer design in the broader scope of genetic engineering. In the context of gene editing technologies, the role of precisely designed primers cannot be overstated. They are the linchpin that often determines the success or failure of CRISPR applications. This article has emphasized that effective primer design is integral to achieving specificity and reducing off-target effects, which are crucial for advancing research in various fields including medicine, agriculture, and biotechnology.
Summarizing Key Points
In reviewing the essence of primer design for CRISPR-Cas9, a few key points emerge as central to the discussion:
- Importance of Specificity: Precision in binding reduces unintended consequences in gene editing, which can lead to harmful effects in cellular contexts.
- Strategic Selection of Targets: Identifying the correct regions to target enhances the efficacy of the CRISPR system.
- Understanding Primer Structure: Awareness of primer length, composition, and melting temperature are vital considerations in their design.
- Utilization of Software Tools: Resources are available that fortify the primer design process, giving researchers a sharper edge in their endeavors.
- Ethical Awareness: Considerations surrounding the usage of CRISPR are paramount, and researchers need to navigate these waters carefully to ensure responsible application.
By reinforcing these points, readers can better appreciate how the careful crafting of primers aligns with successful CRISPR outcomes.
Implications for Future Research
As we propel forward in the genomic arena, the implications of robust primer design methodologies are multifaceted. Future research is likely to benefit greatly from enhanced computational tools that incorporate machine learning and predictive algorithms. Such advancements may provide even more accurate modeling of primer behavior under various biological conditions.
Moreover, a deeper understanding of off-target effects will likely be pursued, leading to innovations that minimize these occurrences further. Researchers are likely to explore the intersection of CRISPR technology and synthetic biology, potentially enabling more nuanced applications in fields such as tissue engineering and therapeutic development.
Lastly, the societal impacts of CRISPR technology encourage a continuing dialogue about the ethical landscape. As applications of CRISPR evolve, building frameworks that promote responsible research practices will be essential.
In summary, the journey of CRISPR primer design is not just a technical exercise; it is a broader commentary on the evolution of biotechnology and its role in addressing significant challenges in medicine and agriculture.