Characterization of Cells: A Comprehensive Analysis
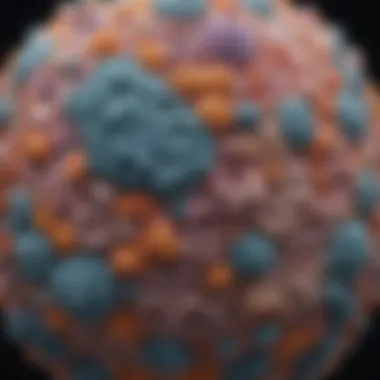
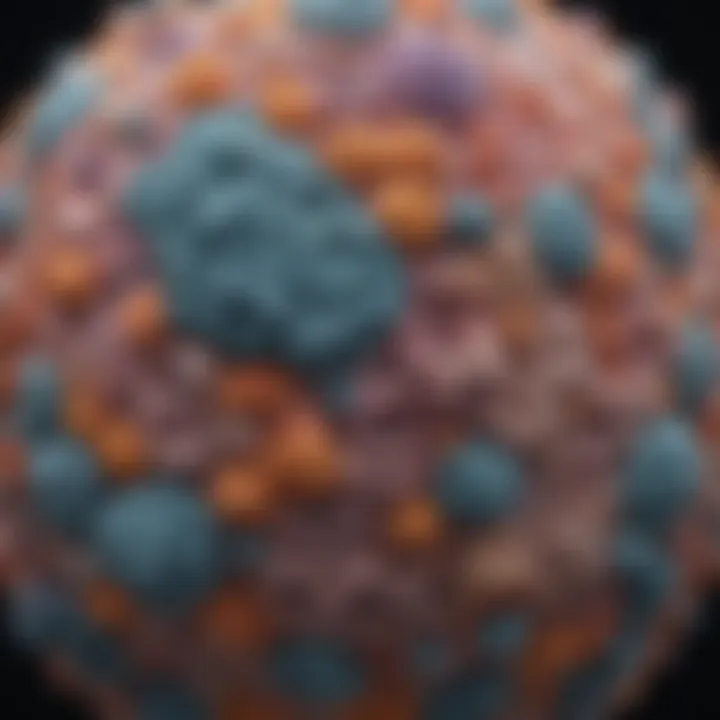
Intro
Characterizing cells is fundamental for understanding biological systems. The complex and diverse nature of cells demands a thorough exploration of their characteristics. Cells are the basic units of life, and their differentiation is crucial in multiple scientific domains. Assuring accurate characterization allows for advancements in health sciences, biotechnology, and pharmaceuticals.
In this section, we will outline critical methodologies for analyzing cells. These approaches will reveal how we measure structural features and functional roles. Furthermore, a focus on modern technologies employed in cellular analysis is essential. The link between these characterization methods and potential advancements in medical research will be examined. By understanding these relationships, we can appreciate the significance of accurate cell identification and classification.
As we progress through the article, we will highlight key findings that impact the scientific community. Implications of research in real-world applications and future directions will also be discussed. This exploration will provide valuable insights for students, researchers, educators, and professionals involved in cell biology.
Prolusion to Cell Characterization
Cell characterization plays a pivotal role in understanding biological systems. It provides insights into the intricate structure and function of cells, the basic units of life. This section sets the stage for delving into various methodologies and their relevance in scientific research. The importance of cell characterization is underscored by its implications across a multitude of fields, from biotechnology to health sciences. Accurate cell identification and classification are essential for advancements in medicine, particularly in the development of targeted therapies and personalized medicine.
Definition and Importance
Cell characterization refers to the extensive analysis of cell properties and behaviors. This encompasses a wide range of aspects, including morphology, functionality, and genetic profiles. The significance of this process cannot be overstated. For instance, understanding the characteristics of various cell types aids in identifying their roles within tissues and organs.
The implications extend to diagnostics, disease modeling, and therapy development. By comprehending cell types such as stem cells or cancerous cells, researchers can innovate treatment methods that are more efficient. Additionally, cell characterization is crucial for the validation of experimental models used in research, ensuring that findings can be accurately interpreted and generalized.
Historical Perspectives
The journey of cell characterization has evolved dramatically over the years. Early microscopes in the 17th century facilitated the initial exploration of cellular structure. Scientists like Robert Hooke and Antonie van Leeuwenhoek laid the foundation for cellular biology by discovering and documenting cells. Over time, advancements in technology have propelled this field forward.
The introduction of staining techniques allowed for better visualization, while the development of electron microscopy provided unprecedented detail about cell ultrastructure. In recent years, high-throughput technologies like single-cell sequencing have emerged. These innovations enable scientists to analyze individual cells, revealing heterogeneity within populations that was previously obscure. This historical context highlights how cell characterization has transformed from simplistic observations to complex, multifaceted analyses, crucial in modern biological research.
"Cell characterization is not just a process; it is a journey through which we unravel the mysteries of life at the cellular level."
Through this evolution in understanding and technique, cell characterization continues to shape the future of biology, paving the way for new discoveries and therapies.
Cell Types and Their Distinct Features
Understanding cell types is essential for the thorough comprehension of cell characterization. Each type exhibits unique attributes and functions, offering insights into biological diversity as well as specialization in multicellular organisms. The implications of these distinctions play a critical role in health sciences, biotechnology, and other fields. Knowing the cellular differentiations aids researchers and practitioners in manipulating and utilizing cells for various applications, thus strengthening the overall understanding of complex biological systems.
Prokaryotic Cells
Prokaryotic cells are distinguished primarily by their simple structure. They lack a membrane-bound nucleus and organelles, making them fundamentally different from eukaryotic cells. Their DNA is typically a single circular chromosome located in the nucleoid. Prokaryotes, such as bacteria and archaea, are vital to ecosystems, contributing to nutrient cycling and energy flows. Understanding prokaryotic cells can lead to advancements in microbiology and biotechnology, including applications in waste treatment, fermentation, and antibiotic production.
Eukaryotic Cells
Eukaryotic cells showcase greater complexity with a defined nucleus and membrane-bound organelles. This group includes a vast array of cell types, each with specific characteristics.
Epithelial Cells
Epithelial cells form protective layers on surfaces throughout the body. They are critical for absorption, secretion, and sensation. The key characteristic of epithelial cells is their ability to form tight junctions, which create barriers that regulate the exchange of substances. Their regenerative capacity makes them a popular subject in regenerative medicine. However, their rapid growth can also lead to issues such as cancer when regulation fails.
Muscle Cells
Muscle cells, or myocytes, are essential for movement and force generation in organisms. They are categorized into three types: skeletal, cardiac, and smooth muscle cells. Each type has distinct structures and functions. The unique feature of muscle cells is their contractile ability, which comes from specialized proteins, actin and myosin. This characteristic promotes their use in studies related to muscle regeneration and repair atrophy. However, challenges in targeting specific muscle types can complicate research.
Nerve Cells
Nerve cells, or neurons, are fundamental units of the nervous system. They transmit electrical signals, which are critical for communication within the body. Neurons possess unique features, such as axons and dendrites, that facilitate signal transmission. Their ability to adapt and form new connections writes the narrative of neural plasticity. For this article, neuron studies offer insights into neurodegenerative diseases and potential therapies. However, the complexity of neural networks can pose challenges in understanding their functions fully.
Stem Cells
Stem cells are remarkable for their ability to differentiate into various cell types. They hold significant potential in regenerative medicine due to their capacity to replace damaged tissues. The key characteristic of stem cells is their pluripotency, especially in embryonic stem cells, which allows them to turn into nearly any cell type in the body. This versatility contributes to their popularity in therapeutic research. Nevertheless, ethical concerns and challenges related to controlled differentiation have garnered ongoing debate in the scientific community.
Understanding cell types is a cornerstone of cellular biology, influencing advances in multiple scientific disciplines.
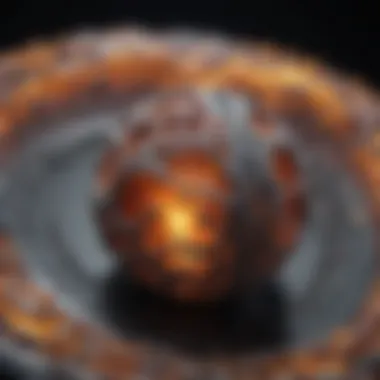
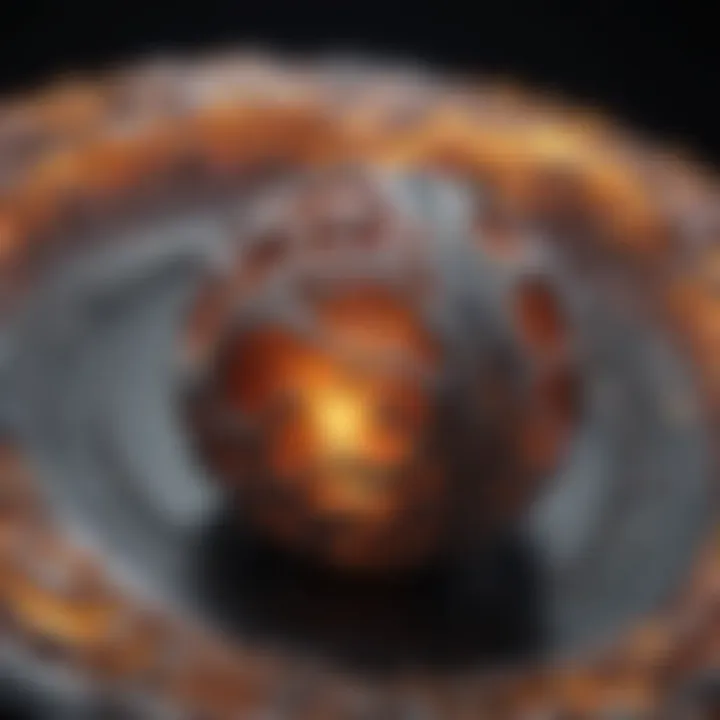
In summary, the examination of cell types enhances our insight into the roles and functions of different cells in living organisms. Acknowledging the diversity and unique traits of prokaryotic and eukaryotic cells emphasizes their significance in various biological processes, making this exploration pivotal in the realm of cell characterization.
Key Techniques for Cell Characterization
Cell characterization is essential in understanding the complex biology of cells. The techniques used for this purpose enable scientists to obtain a broad range of information regarding cellular behavior, structure, and function. In this section, we will explore various key techniques that provide insights into cell characterization. We examine how these methodologies not only support research but also have wide applications in different fields such as medicine and biotechnology.
Microscopy
Microscopy is one of the foundational techniques in cell characterization. It allows researchers to visualize cells and their components, making it possible to analyze morphology and structure in detail. The advancements in microscopy have significantly enhanced our understanding of cellular processes.
Light Microscopy
Light microscopy is perhaps the most commonly used technique for cell visualization. This method uses visible light and optical lenses to magnify cellular structures. A key characteristic of light microscopy is its ability to provide a relatively quick and easily accessible approach for examining cells. This method is beneficial for routine assessments of cell shape and arrangement. One unique feature of light microscopy is its capacity to allow live-cell imaging. This capability lets researchers observe dynamic processes in real time.
However, the resolution of light microscopy is limited compared to other techniques, making it challenging to resolve structures smaller than approximately 200 nanometers.
Electron Microscopy
Electron microscopy offers a significantly higher resolution than light microscopy by using a beam of electrons instead of light. A key aspect of this technique is its ability to visualize ultra-structural details at the nanometer scale. This feature is particularly valuable for distinguishing sub-cellular components. Due to its high resolution, electron microscopy becomes a critical tool in fields such as nanobiotechnology and viral studies.
Despite these advantages, the electron microscopy process is time-consuming and requires sample preparation that may alter the specimen being observed. This potential artifact generation can be a drawback for certain applications.
Fluorescence Microscopy
Fluorescence microscopy employs fluorescent dyes to label specific proteins or cellular components, allowing for targeted visualization. This technique is a powerful option because it can provide information about the location and dynamics of molecules inside the cells. It allows for multiplexing, meaning that different markers can be detected simultaneously in the same sample. A unique characteristic of fluorescence microscopy is its capability to provide data on cellular interactions and processes.
However, fluorescence microscopy can sometimes suffer from photobleaching, where fluorescent signals diminish over time with exposure to light. This limitation may affect the duration and accuracy of observations.
Flow Cytometry
Flow cytometry is a highly quantitative technique used to analyze the physical and chemical characteristics of cells in a mixed population. Cells are forced in a single-file line through a laser, and as they pass, the system measures their properties such as size, granularity, and fluorescence. This technique allows for rapid analysis of thousands of cells per second. One significant advantage of flow cytometry is its capacity for multiparametric analysis, which provides detailed information about individual cells within a heterogeneous population. Nevertheless, the complexity of the equipment and the need for specific reagents can be a barrier for some laboratories.
Genomic and Proteomic Analysis
Genomic and proteomic analyses are integral for cellular characterization, aiding in the comprehensive understanding of cellular function and behavior.
DNA Sequencing
DNA sequencing offers the ability to determine the nucleotide order in a cell's genome. This information is fundamental to understanding genetic variations and anomalies tied to different cultures or disease states. One remarkable aspect of DNA sequencing is the advent of high-throughput techniques, which allow for rapid sequencing of multiple samples. However, challenges remain in interpreting vast amounts of sequence data and linking them to biological significance.
RNA Analysis
RNA analysis is vital to understanding gene expression. By measuring RNA levels, researchers can gain insights into cellular responses to varying stimuli. A key characteristic of RNA analysis is its ability to reflect dynamic processes in real time. Technologies such as RNA-Seq have made this process quicker and more efficient. However, challenges include the stability of RNA samples and achieving accurate quantification across different cellular contexts.
Protein Profiling
Protein profiling provides information about the types and quantities of proteins within a given cell type. It allows researchers to explore cellular functions, signaling pathways, and interactions. Mass spectrometry is a key technology in protein profiling, known for its sensitivity and coverage. However, the complexity and variability in protein expression can make this analysis challenging, necessitating advanced statistical methods to derive meaningful conclusions.
In summary, mastering the key techniques for cell characterization is crucial for advancing our understanding of cellular biology, with each technique providing unique insights and facing its own specific challenges.
Biophysical and Biochemical Properties of Cells
Understanding the biophysical and biochemical properties of cells is essential for fully characterizing their behavior and functionality. These properties influence how cells interact with their environment, communicate with each other, and respond to various stimuli. By examining these characteristics, researchers can gain insights into cellular mechanisms, which is crucial for advances in biomedical research and therapeutic development.
Cell Membrane Characteristics
The cell membrane is a critical structure that defines the boundary between the internal cellular environment and the external surroundings. It is primarily composed of a lipid bilayer with embedded proteins. This unique arrangement provides both structure and fluidity, allowing for selective permeability.
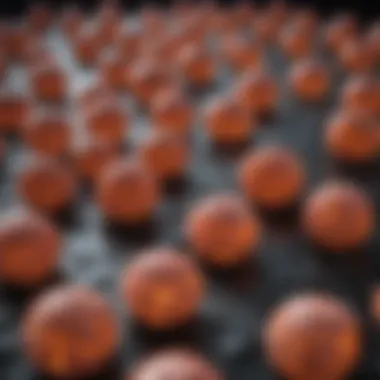
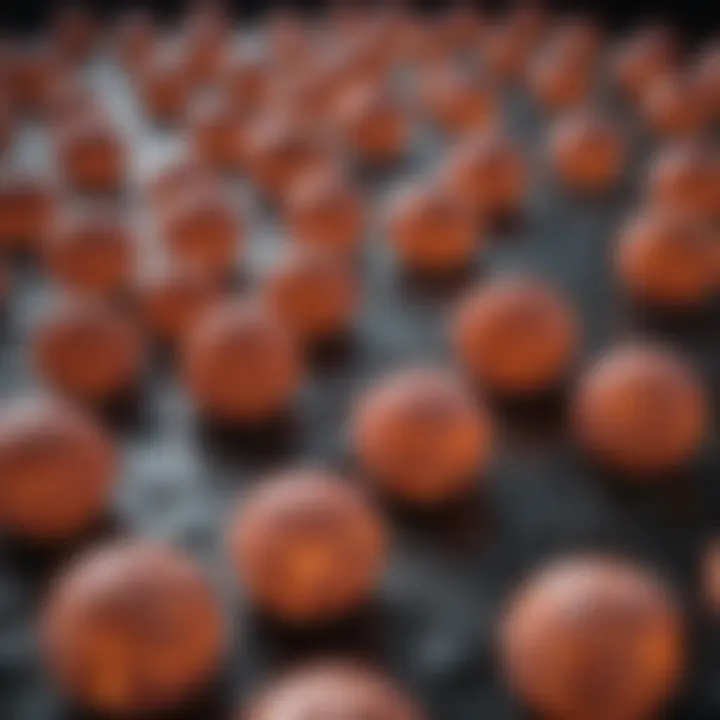
- Phospholipids: The fundamental building blocks of the membrane are phospholipids. Their hydrophilic heads face outward, while the hydrophobic tails face inward, creating a barrier that separates the cell from its environment.
- Proteins: Integral proteins play vital roles in transport, signaling, and structural support. They facilitate various functions, such as the transport of ions and nutrients.
The fluid mosaic model describes how components of the membrane can move laterally within the lipid bilayer. This movement is crucial for processes like endocytosis and exocytosis. Understanding membrane dynamics helps in various areas, including drug development and understanding disease mechanisms.
Cytoplasmic Composition
Cytoplasmic composition encompasses a range of biomolecules and organelles that contribute to cellular functions. The cytoplasm is a jelly-like substance that fills the cell and houses various cellular components.
Key elements of cytoplasmic composition include:
- Organelles: Organelles like mitochondria, ribosomes, and endoplasmic reticulum are essential for metabolic processes.
- Cytoskeleton: This network of fibers provides structural support and enables cellular movement. It plays important roles in cell division and intracellular transport.
- Metabolites and Ions: The cytoplasm contains a range of small molecules, such as glucose and ions, crucial for energy production and maintaining osmotic balance.
The composition of the cytoplasm can vary between cell types. For instance, muscle cells exhibit more mitochondria due to their high energy demands, while neurons may have specialized organelles for neurotransmitter release.
Overall, the biophysical and biochemical properties of cells provide a foundation for understanding their functional roles in health and disease. Advances in this area are likely to influence future research directions as scientists seek to unravel more complex biological processes.
Applications of Cell Characterization
Understanding the applications of cell characterization is crucial for various scientific domains. The processes involved in characterizing cells provide insights that enable advances in multiple fields. This section will explore how these applications shape biomedical research, drug development, and biotechnology. Each application reveals unique benefits and considerations, underscoring the importance of accurate cellular analysis.
Biomedical Research
Cell characterization plays a fundamental role in biomedical research. By examining the properties and functions of different cell types, researchers can gain insights into disease mechanisms. For instance, characterizing cancer cells allows scientists to understand tumor biology and the interactions between cancer and immune cells. This knowledge is vital for developing targeted therapies.
Moreover, characterizing stem cells offers avenues for regenerative medicine. Identifying specific markers can assist in distinguishing stem cells from other cell types, allowing for more reliable applications in cell therapies.
In summary, the implications of cell characterization in biomedical research extend to:
- Understanding disease mechanisms
- Identifying therapeutic targets
- Enhancing regenerative medicine efforts
Drug Development
In drug development, cell characterization is essential for screening potential drug candidates. By studying how cells respond to various compounds, researchers can evaluate drug efficacy and safety. High-throughput screening, which involves testing many compounds simultaneously, heavily relies on accurate cellular characterization to generate meaningful data.
Furthermore, understanding cell biology facilitates the identification of biomarkers that can predict drug responses. This leads to more personalized medicine approaches. Accurate characterization of cells ensures that therapies are tailored to individual patient profiles, increasing treatment success.
Key benefits in drug development include:
- Tenability of testing processes
- Predicting patient responses
- Reducing time in drug discovery
Biotechnology and Synthetic Biology
Cell characterization is also a cornerstone of biotechnology and synthetic biology. In these fields, understanding the functional aspects of cells enables innovations in product development and industrial applications. For example, microorganisms are often engineered for bioproduction, where precise cellular attributes determine their viability for producing biofuels, pharmaceuticals, or bio-based materials.
Additionally, synthetic biology combines biological science and engineering. Comprehensive cell characterization leads to the development of new synthetic constructs that perform specific functions. This paves the way for advances in environmental sustainability and resource management.
Considerations in this context include:
- Effective optimization of cellular systems
- Innovating sustainable solutions
- Addressing global challenges through biotechnological advances
Accurate cell characterization is not just about understanding cells; it is about translating that understanding into practical applications that benefit numerous fields.
Challenges in Cell Characterization
The process of cell characterization encompasses several obstacles that can imped the accuracy and reliability of analysis. Understanding these challenges is crucial, as they have diverse implications across various scientific domains. Whether in biomedical research, biotechnology, or pharmaceutical development, recognizing these challenges helps clarify the steps needed to enhance cell characterization.
One significant barrier is the interpretation of data generated from various characterization methods. Different technologies can yield large data sets, but the task of making sense of this information is not straightforward.
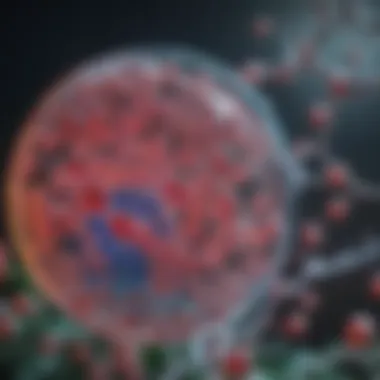
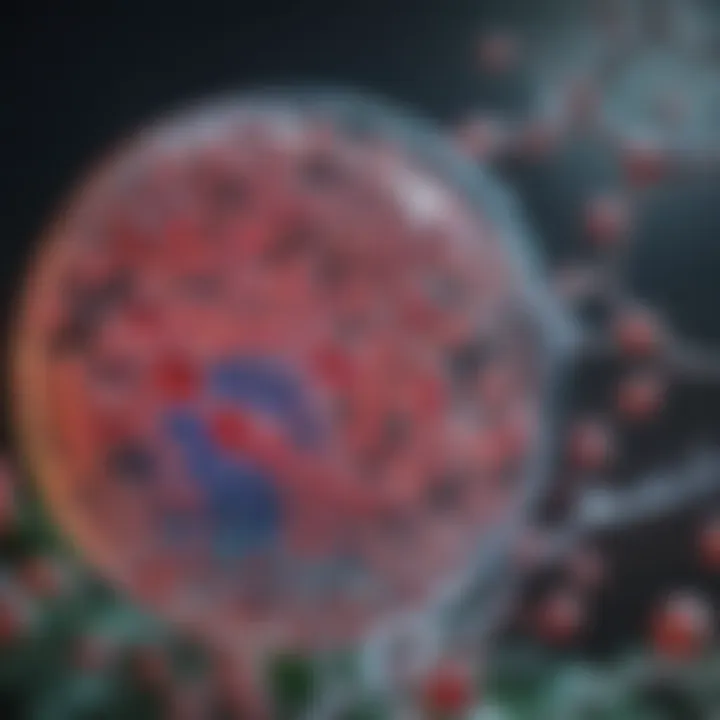
Quantitative data from techniques like flow cytometry or genomic analyses often produce complex results. These results can be confusing due to the noise, variability, and inherent limitations of the techniques employed. For instance, a small change in a cellular parameter can result in substantial differences in population data, leading researchers to inaccurate conclusions. Therefore, careful statistical and computational tools are necessary for data interpretation, ensuring that findings are statistically valid and biologically relevant.
Data Interpretation Issues
Data interpretation presents challenges that can undermine the goals of researchers. Factors contributing to these issues include:
- Variability in Results: Differences in techniques, sample processing, or even the environment can lead to significant variations in data outputs.
- High Dimensionality: Multidimensional data often requires advanced analytical methods, which might not be accessible to all researchers.
- Subjectivity: Certain qualitative assessments introduce bias based on the researcherβs experience or expectations.
Adopting integrative approaches that combine diverse data types can help in mitigating some interpretation issues. By utilizing bioinformatics tools and collaborative methodologies, researchers can arrive at more robust conclusions. This is increasingly important in complex research areas where cell characterization plays a foundational role.
Standardization of Techniques
Another critical challenge is the standardization of techniques used in cell characterization. The lack of universally accepted protocols can lead to inconsistent results across studies. Inconsistencies can arise from variations in sample handling, processing protocols, or even the equipment used.
To address this issue, several key considerations must be taken into account:
- Establishing Protocols: The scientific community needs clear, replicable methodologies that define procedures for sample preparation, analysis, and interpretation.
- Quality Control: Implementing rigorous quality control measures throughout the characterization process is essential. This helps ensure that results are comparable across different laboratories.
- Cross-Validation: Utilizing multiple characterization methods to validate findings can reduce reliance on any single technique's limitations.
Implementing these standardization efforts can significantly enhance the reliability of cell characterization outcomes. Without effective solutions to these challenges, the progress in understanding cellular functions and the development of therapeutics may face considerable hindrances.
Key Takeaway: Addressing data interpretation issues and standardizing techniques are vital for improving the reliability and consistency of cell characterization, ultimately propelling advancements in various scientific fields.
Future Directions in Cell Characterization
As science continues to evolve, the characterization of cells is at the forefront of research in various disciplines. Understanding how to classify and analyze cells with increasing precision is essential for breakthroughs in medicine, biotechnology, and basic biological research. Future directions in this field will undoubtedly reshape our approaches and inventions, leading to significant advancements.
Emerging Technologies
Single-Cell Sequencing
Single-cell sequencing allows researchers to study the genome, transcriptome, or epigenome of individual cells. This method is crucial for identifying cellular heterogeneity within tissues, which helps unravel complex biological systems. A key characteristic of single-cell sequencing is its ability to provide detailed insights into gene expression patterns at an unprecedented resolution. This technology is beneficial in studying rare cell types that may have different functions or states within a population.
One unique feature of single-cell sequencing is the ability to uncover cellular responses to various stimuli in real-time. This capability enables researchers to understand the dynamics of cellular behavior under different conditions. The advantages of this technology include its high-throughput nature and capacity for deep biological insights. However, the challenges include the higher cost associated and the complexities in data interpretation, which must be managed to maximize its effectiveness.
High-Throughput Screening
High-throughput screening represents another pivotal technology to enhance cell characterization. This method allows for the rapid testing of thousands of samples simultaneously. Its key characteristic is the automation and miniaturization of assays, which accelerates the process of identifying active compounds or potential therapeutic agents. High-throughput screening is quite popular due to its efficiency and scalability, making it an attractive option for drug discovery and biomedical research.
A unique feature of high-throughput screening is its integration with various assay technologies, which enables simultaneous measurement of multiple biological responses. This method provides the advantage of speed while still delivering robust datasets for analysis. However, one consideration is the potential for false positives or negatives in data, which calls for careful validation of results to ensure reliability.
Integrative Approaches
Integrative approaches are essential to future advancements in cell characterization. By combining various techniques and methodologies, researchers can foster a more comprehensive understanding of cellular functions and interactions. These approaches emphasize the importance of multi-dimensional data analysis, allowing for the integration of genomics, proteomics, and metabolic profiling. This holistic perspective can lead to more accurate predictions of cellular behavior and improved experimental designs, contributing to enhanced applications in health sciences and biotechnology.
The End
The conclusion serves as a pivotal section within this article, encapsulating the comprehensive examination of cell characterization. As we reflect on the various themes explored, it becomes evident that the understanding of cellular properties is integral to the advancement of scientific knowledge. A thorough analysis of cell types, their distinct features, and analytical methodologies offers a framework for subsequent research and applications.
Summarizing Key Insights
The investigation into cell characterization highlights several key insights that are essential for both academic and practical contexts. Firstly, the differentiation between prokaryotic and eukaryotic cells reveals foundational knowledge required for various biological disciplines. Understanding how cellular structures affect function is crucial for developing new therapeutic approaches.
- Cell types each possess unique attributes that dictate their roles in biological systems.
- Technological advancements, such as microscopy and flow cytometry, have revolutionized our ability to analyze cells in unprecedented detail.
- Insights from genomic and proteomic studies inform researchers about cellular behavior under diverse conditions.
Each of these points underscores the sophistication and variability present in cellular biology.
The Role of Cell Characterization in Scientific Advancement
Cell characterization is not merely an academic exercise; it plays a fundamental role in advancing science and technology. Accurate cell identification and classification have broad implications in biomedical research and therapeutic development. In oncology, for example, characterizing tumor cells helps in designing targeted treatments that are more effective and less harmful to normal tissues.
Furthermore, advancements in biotechnology rely heavily on cell characterization. The ability to modify and engineer cell types allows for innovations in drug development and synthetic biology. As more sophisticated tools and techniques are adopted, the precision in characterizing cells will only improve, leading to better diagnostics and treatments.
"The ongoing evolution of cell characterization technologies will shape the future of biological research and healthcare, reinforcing the need for continual investment in this field."