Cancer Cells vs Normal Cells: Key Differences Explained
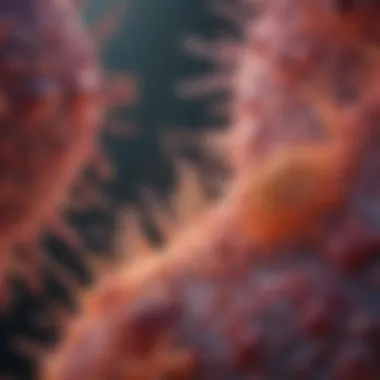
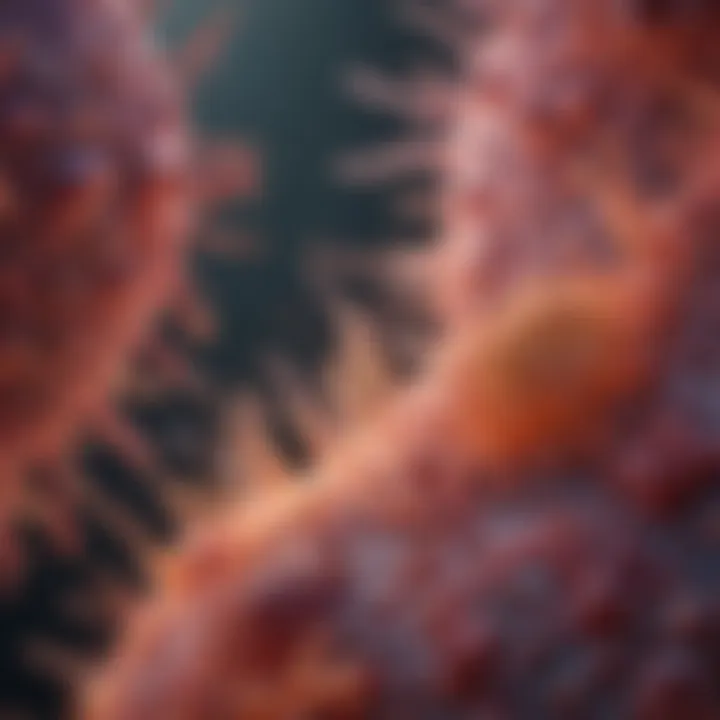
Intro
Cancer cells and normal cells serve as the foundation for understanding a vital aspect of cellular biology. The stark contrast between these two cell types underpins many of the challenges in cancer treatment and therapy. While normal cells play their roles in the body harmoniously, contributing to overall health and function, cancer cells emerge through a series of mutations, leading to a loss of normal regulatory controls. This divergence paves the way for uncontrolled growth, invasion, and often, a resistance to treatments that are effective against normal cells.
The intricacies of this dynamic are immense. Dipping into genetic variations, metabolic functions, and how these cells behave within their environments reveals much about their nature. Moreover, studies show that the tumor microenvironment, comprising various cell types and signaling molecules, significantly influences cancer progression. It is essential to comprehend these nuances, especially when one considers the focus on developing more effective therapeutic strategies.
Through this examination, the goal is to clarify how the differences between cancer and normal cells shape both the field of research and potential treatment pathways. By emphasizing both cellular characteristics and shared biological processes, researchers can devise better approaches to combat cancer, paving the way for innovative therapies in a field that has seen significant advancements in recent years.
Intro to Cell Biology
Cell biology forms the cornerstone of understanding life at its most fundamental level. This field of study reveals how cells, the basic units of life, operate and interact with one another in a complex symphony. A complete grasp of cell biology is particularly vital in areas like cancer research because differences in cellular function and structure can delineate the boundaries between health and disease. Learning how normal and cancer cells can diverge in their behaviors sheds light on critical mechanisms that might inform therapeutic strategies.
Understanding cell biology is not just about recognizing the shapes and components of cells. It encompasses the nuances of how cells communicate, their metabolic activities, and the various roles they play in larger biological systems. This knowledge is essential, especially when contemplating how disruptions in these processes can lead to conditions like cancer. A strong foundation in cell biology aids in decoding the intricate cellular conversations that dictate growth, signaling, and homeostasis.
Moreover, grasping these foundational elements serves several purposes.
- Foundational Knowledge: It lays the framework for comprehending more advanced topics in genetics and molecular biology.
- Clinical Relevance: Insights gathered from cell biology inform clinical approaches to diagnosis and therapy in diseases like cancer.
- Novo Research Directions: It opens up various avenues for innovative research, guiding scientists toward unexplored territories in cellular dysfunction.
Ultimately, diving into cell biology helps illuminate the complexities residing within each cell, thereby setting the stage for a detailed examination of cancer cells versus normal cells.
Defining Cellular Structures
Cells are not mere blobs of protoplasm wading through life; they are intricate structures composed of various parts, each with specific functions. The most prominent structures include:
- Cell Membrane: This thin barrier governs the interchange of substances with the external environment, permitting some molecules to pass while denying others.
- Nucleus: Serving as the command center, the nucleus contains the cell's DNA and orchestrates activities such as growth and reproduction.
- Mitochondria: Often dubbed the powerhouses of the cell, mitochondria are responsible for energy production through metabolism.
- Cytoplasm: This gel-like substance fills the cell, housing organelles and facilitating molecular interactions.
Each of these components is not just a stand-alone entity but rather part of a larger, cohesive system that works in harmony to maintain life. In the context of cancer, understanding these structures becomes crucial. The normal functioning of these cellular structures is often compromised in cancer cells, leading to uncontrolled growth and other aberrations.
The Role of Cells in Organisms
Cells are remarkable building blocks that come together to form tissues, organs, and ultimately, organisms. Each type of cell plays a unique role in maintaining the overall health and sustainability of the organism.
Consider the human body:
- Muscle Cells allow movement.
- Nerve Cells transmit signals for communication.
- Epithelial Cells form protective barriers.
In normal physiological conditions, cells maintain a delicate balance between growth, division, and death. This equilibrium is maintained through regulatory mechanisms that govern the cell cycle and programmed cell death, or apoptosis. In healthy organisms, these processes ensure that cells respond appropriately to their environment and that damaged cells are systematically eliminated. However, when these processes malfunction, as is often the case in cancer, the consequences can be dire.
Understanding the pivotal roles of different cell types provides invaluable context when analyzing how cancer cells hijack normal processes to thrive. This contrast between normal and malignant cells underscores the need for targeted therapies that can selectively dismantle the aberrant behavior seen in cancer while sparing the healthy cells that sustain life.
Normal Cells Explained
Understanding normal cells is akin to knowing the foundation of a building; without it, one cannot comprehend the structures that arise upon it. Normal cells are the basic units of life that perform vital functions, being essential for the growth, repair, and maintenance of organisms. They operate in a harmonious manner, adhering to strict growth control mechanisms that ensure the body functions smoothly. In this section, we will explore the characteristics of normal cells, their regulation during the cell cycle, and the process of apoptosis, which is vital for maintaining cellular homeostasis.
Characteristics of Normal Cells
Normal cells are distinct in several ways, which anchor their identity and functionality. These cells typically exhibit:
- Defined Structure: Surrounded by a cell membrane, normal cells have a nucleus containing genetic material, with varying organelles tailored to their specific functions.
- Organizational Cohesion: They form tissues and organs, working collaboratively with other cells to execute bodily functions. Take, for instance, epithelial cells lining the skin that protect underlying tissues.
- Adherence to Growth Signals: Normal cells respond to external signals, growing only when necessary and stopping when there's enough of them. This orderly growth prevents overcrowding and maintains tissue integrity.
- Limited Lifespan: These cells usually undergo a predetermined number of divisions before becoming senescent or dying, a sort of biological timer that helps maintain healthy cell turnover.
Cell Cycle Regulation
The cell cycle is a tightly regulated series of events that lead to cell division and duplication. It consists of phases: G1 (gap 1), S (synthesis), G2 (gap 2), and M (mitosis). Each phase is regulated by a complex interplay of proteins and checkpoints.
- Checkpoints: These mechanisms ensure that cells only progress to the next stage if conditions are favorable. For example, the G1 checkpoint checks for DNA damage and the presence of necessary growth factors.
- Cyclins and Cyclin-Dependent Kinases (CDKs): These proteins form complexes that drive the cell cycle processes. If issues arise, such as DNA damage, the cell can halt progression, allowing for repair or programmed death.
This regulation is crucial as any disruption might lead to uncontrolled growth, which is a hallmark of cancer cells.
Apoptosis: The Programmed Cell Death
Apoptosis, or programmed cell death, is an essential process in maintaining tissue homeostasis and eliminating damaged or potentially harmful cells. Unlike necrosis, which can result from injury or infection, apoptosis is a controlled mechanism that ensures cellular debris does not trigger inflammation.
- Initiation Factors: Normal cells can enter apoptosis through intrinsic signals (like DNA damage) or extrinsic signals (such as receptor ligand interactions).
- Execution Phase: This is characterized by specific morphological changes, such as cell shrinkage, chromatin condensation, and even DNA fragmentation, leading to the cell being neatly packaged and removed by immune cells.
- Importance of Apoptosis: This process is vital in development, allowing for the removal of unnecessary cells. Notably, in cancer, the evasion of apoptosis allows abnormal cells to survive longer, contributing to tumor growth.
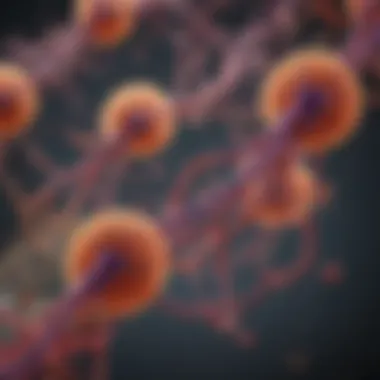
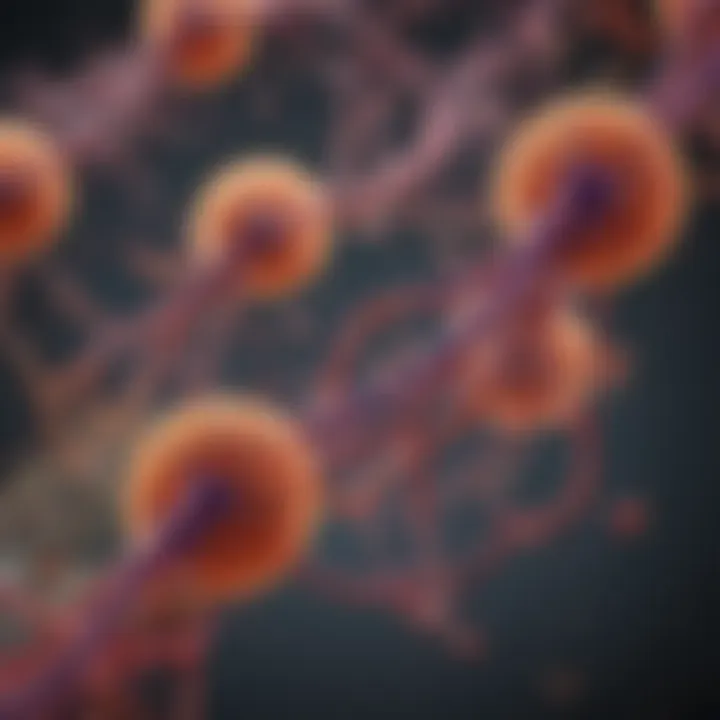
"The balance between cell death and cell proliferation is a cornerstone of healthy tissue homeostasis."
Recognizing the characteristics and functions of normal cells sets the stage for exploring how deviances in these processes contribute to the emergence of cancer cells, a critical point of discussion in this article.
Cancer Cells: A Distinct Entity
Cancer cells stand apart in the realm of biology, carving out a niche that challenges conventional understandings of cellular behavior. Recognizing their uniqueness is paramount, as this understanding not only illuminates the field of oncology but also paves the way for enhanced treatment strategies. The distinct nature of cancer cells manifests through various mechanisms, particularly in how they proliferate, their genetic makeup, and their survival strategies in hostile environments. This section intends to shed light on these aspects, weaving a narrative that highlights the complexities of cancer cells versus their normal counterparts.
Defining Cancer Cells
When we talk about cancer cells, we're referring to cells that have undergone a series of mutations, leading them down a path of unchecked growth and division. Unlike normal cells, which adhere to strict regulatory mechanisms, cancer cells often sidestep these controls. They have the uncanny ability to proliferate indefinitely, largely due to telomere maintenance and evasion of apoptosis.
In essence, cancer cells can be described as malignant entities that can invade surrounding tissues and metastasize to distant sites within the body. For example, a breast cancer cell can migrate to the lungs, forming secondary tumors. This invasive capacity distinguishes them fundamentally from normal cells, which respect territorial boundaries within tissues.
Hallmarks of Cancer Cells
The concept of the "hallmarks of cancer" serves as a framework to understand the essential traits that define cancer cells. These hallmarks are:
- Sustaining proliferative signaling: Cancer cells can continually signal themselves or neighboring cells to keep dividing.
- Evading growth suppressors: They avoid mechanisms that normally inhibit cell division.
- Resisting cell death: Instead of undergoing apoptosis, cancer cells find ways to survive despite damage.
- Enabling replicative immortality: Through mechanisms like the reactivation of telomerase, these cells can replicate indefinitely.
- Inducing angiogenesis: They can stimulate the growth of new blood vessels to nourish the tumor.
- Activating invasion and metastasis: Cancer cells gain the ability to spread to other parts of the body.
These characteristics provide insight into how cancer cells operate differently. It’s a classic case of what one might call 'the great escape,' where cells defy the usual restrictions that normal cells abide by, allowing them to thrive in environments that would typically signal the end of life.
Dysregulation of the Cell Cycle
The cell cycle is the pivotal process through which normal cells divide and reproduce. In cancer, this cycle becomes critically dysregulated.
Normal cells progress through a series of checkpoints that assess whether conditions are favorable for division. However, in cancer cells, these regulatory checkpoints can be bypassed due to mutations in genes that oversee cell cycle control. This results in a frenetic pace of division without the usual hesitations.
What’s often found is a disruption in tumor suppressor genes like p53, which usually function to halt the cycle in response to cellular damage. Instead of enforcing pause, these mutated genes allow cancer cells to barrel onward, often leading to the accumulation of further mutations and instability.
The unchecked division of cancer cells challenges the very fabric of tissue organization, leading to the chaotic structure often seen in tumors.
In summary, the distinct entity of cancer cells is characterized by their abnormal growth patterns, evasion of normal signaling mechanisms, and overall resilience. Understanding these factors equips researchers and practitioners with the knowledge necessary to combat cancer more effectively.
Genetic and Epigenetic Factors
In the realm of cell biology, a thorough understanding of genetic and epigenetic factors is vital when distinguishing between cancer cells and normal cells. What sets apart these two types of cells at the most fundamental level often lies within their genetic blueprint and its regulation. These elements drive not just the growth and function of the cells, but also their response to various stimuli, including therapeutic interventions. Grasping these differences can lead to more effective treatment routes and a clearer picture of how cancer evolves.
Mutations and Cancer Development
Mutations represent alterations in the DNA sequence — they may occur due to several factors, such as exposure to certain chemicals, radiation, or even just errors in DNA replication. These changes can either be harmful or harmless. However, when it comes to cancer, it's generally the transformative mutations that play a pivotal role. Take, for instance, the well-known p53 gene, which usually functions to repair damaged DNA or initiate cell death if the damage is irreparable. When there's a mutation in p53, the cell may continue to function despite having severe DNA damage, leading to unchecked growth.
Moreover, mutations can be classified into two categories:
- Germ-Line Mutations: Passed down through generations and present in every cell. Conditions like hereditary breast and ovarian cancer syndrome stem from these mutations.
- Somatic Mutations: These occur in non-reproductive cells and cannot be passed on. They can arise at any point in a person’s life and are often associated with the development of a specific cancer.
Thus, understanding the types of mutations and their mechanisms is essential for developing targeted therapies that can effectively address the root causes of cancer's progression.
The Role of Oncogenes and Tumor Suppressor Genes
Within the intricate dance of cellular regulation, two key players emerge: oncogenes and tumor suppressor genes. Oncogenes can be thought of as the culprits that promote cell proliferation. When a proto-oncogene undergoes a mutation, it can become an oncogene that mistakenly signals the cell to grow uncontrollably. For instance, the HER2/neu gene is an oncogene that, when overexpressed, can lead to aggressive breast cancer.
In contrast, tumor suppressor genes work in the opposite direction; they act as guardians of the cell cycle. These genes, like BRCA1 and BRCA2, help regulate cell division and repair damaged DNA. When mutations occur in these genes, their protective role diminishes, paving the way for abnormal cell growth.
The balance between these gene types is crucial; a single malfunction can tip the scales toward cancer development. Therefore, research aimed at restoring this balance holds promise in cancer treatment strategies.
Epigenetic Modifications in Cancer
Epigenetics introduces another layer of complexity to understanding cancer. Unlike genetic mutations that alter the DNA sequence itself, epigenetic modifications affect how genes are expressed without changing the underlying DNA. Factors such as methylation of DNA and modifications to histones can silence or activate specific genes, which may significantly influence a cell's fate.
For example, hypermethylation of the promoter region of tumor suppressor genes can lead to their silencing, effectively removing the brakes on cell proliferation. Similarly, aberrant histone modifications can promote the expression of oncogenes.
These modifications are not permanent and can be influenced by environmental factors, lifestyle choices, and even diet. Therefore, epigenetic research has potential implications not only for understanding cancer development but also for devising new therapeutic avenues.
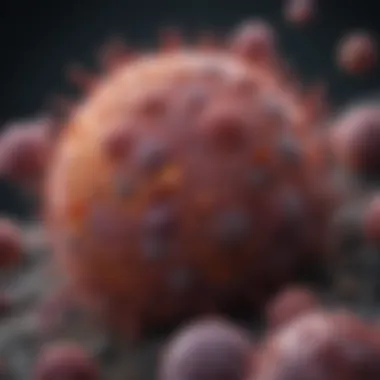
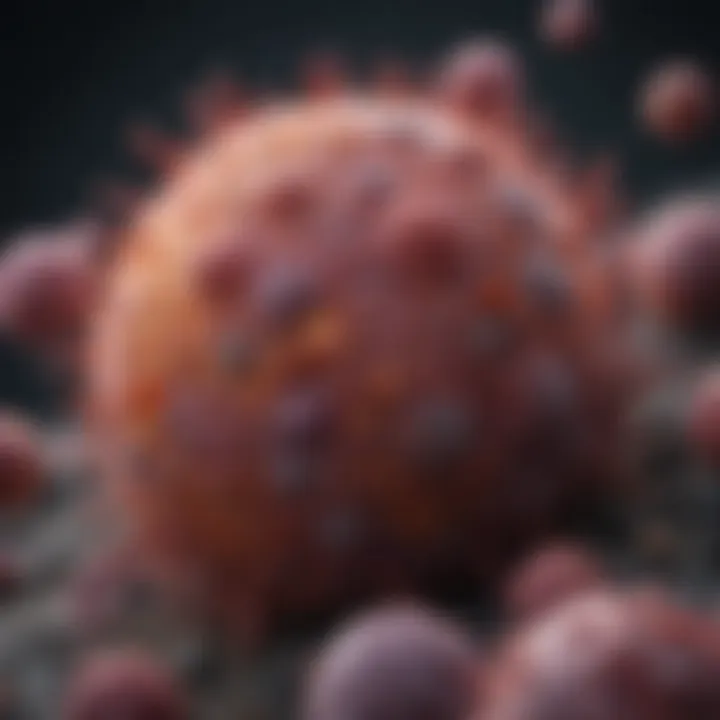
Understanding these genetic and epigenetic dynamics represents a crucial step in discerning how cancer cells differ from their normal counterparts and can greatly impact therapeutic strategies. As such, it marks a significant frontier in cancer research.
Metabolic Variations
Metabolism is the set of life-sustaining chemical reactions in organisms, and understanding it within the context of cancerous and normal cells provides crucial insights into how these cells function differently. The distinction in metabolic processes is a key point in the study of cancer biology. Not only does it highlight the unique characteristics of cancer cells, but it also opens doors for therapeutic innovations.
Normal Cell Metabolism
Normal cells utilize a balanced metabolic profile, ensuring that energy production occurs efficiently to sustain cell growth and repair. The predominant mechanism is aerobic respiration, where glucose is converted into ATP, the energy currency of the cell, under the presence of oxygen. This process is highly regulated and involves glycolysis, the citric acid cycle, and the electron transport chain.
Normal cells tend to prioritize the complete oxidation of nutrients, which yields the maximum amount of energy. Moreover, they rely on regulatory pathways to maintain homeostasis. Factors like nutrient availability and hormonal signaling, including insulin, play significant roles in regulating these metabolic processes.
Consider the process of gluconeogenesis, where non-carbohydrate substrates are transformed into glucose, particularly during fasting. This allows normal cells to adapt to environmental fluctuations, such as nutrient availability. Thus, the metabolic flexibility of normal cells is vital for maintaining overall cellular health and functionality.
Altered Metabolism in Cancer Cells
In stark contrast, cancer cells exhibit altered metabolic pathways often described by the term "Warburg effect." This phenomenon refers to the preference of cancer cells to generate energy mainly through glycolysis, even in the presence of ample oxygen. Instead of fully oxidizing glucose, they convert it to lactate, a process less efficient in energy production but enables the rapid generation of substrates needed for biosynthesis.
This shift isn’t just a random occurrence but a byproduct of the cellular changes that cancer undergoes. Here are some reasons why metabolic alterations occur:
- Rapid proliferation: Cancer cells must divide more frequently, leading to increased demand for nucleotides and amino acids, which the altered metabolism facilitates.
- Hypoxia: Tumors often outgrow their blood supply, resulting in low oxygen levels, triggering a reliance on glycolysis for energy.
- Microenvironmental influences: The tumor microenvironment also shapes the metabolism of cancer cells. Factors like acidosis, hypoxia, and nutrient deprivation lead to extensive metabolic adaptations.
In summary, the metabolic variations between normal and cancer cells not only reflect their unique biological activities but also highlight potential avenues for targeted therapies. As research progresses, understanding these metabolic differences will be paramount in developing interventions that can selectively disrupt the metabolism of cancer cells while sparing normal tissues.
"Understanding the metabolic distinctions between normal and cancer cells may lay the groundwork for creating novel therapeutic options that could ultimately save lives."
The role of metabolism in cancer progression is an area of ongoing research and interest within the field of oncology. By further examining these metabolic pathways, researchers hope to uncover new strategies in cancer treatment that can turn the tide against this formidable adversary.
The Tumor Microenvironment
The tumor microenvironment (TME) plays an indispensable role in understanding how cancer cells thrive and develop, creating a complex interplay of various elements that can promote tumor growth and survival. This environment not only consists of cancer cells but also includes various non-cancerous cells, extracellular matrix components, and signaling molecules that interact dynamically. It's not just a passive backdrop for tumor development; rather, it actively influences how cancer cells behave, respond to treatments, and interact with the host’s immune system.
Exploring the tumor microenvironment can provide insights into potential therapeutic strategies. By understanding the specific elements within the TME, researchers can uncover why certain therapies fail, leading to a more targeted approach in treatment development. It’s clear that the influence of the microenvironment is a double-edged sword; it can either aid in cancer progression or support the host’s defense against tumors. Thus, grappling with the nuances of TME is crucial in the broader context of cancer research and therapy.
Components of the Tumor Microenvironment
The TME is a concoction of various components that collectively drive tumor behavior:
- Cancer-Associated Fibroblasts (CAFs): These are a type of connective tissue cell that is often activated in cancer. They secrete growth factors, cytokines, and extracellular matrix proteins, creating a favorable niche for tumor growth.
- Immune Cells: A mix of immune cells exist within the TME. While some like macrophages can initiate anti-tumor responses, others such as tumor-associated neutrophils can aid in tumor progression.
- Extracellular Matrix (ECM): This provides structural support to the tumor and is crucial for cell signaling. Changes in ECM composition can affect tumor cell proliferation and migration.
- Endothelial Cells: These cells line the blood vessels and are vital for angiogenesis – the formation of new blood vessels to supply nutrients to the tumor.
- Adipocytes: Fat cells can influence tumor behavior, supporting the growth of cancer cells by providing fatty acids.
- Signaling Molecules: Various growth factors and cytokines circulate in the TME, directly affecting cell behavior and communication.
The interactions among these components create a highly adaptable environment that allows tumor cells to continuously evolve, making it imperative to comprehend how they contribute to cancer's dynamics.
Interactions Between Cancer Cells and Surrounding Cells
Understanding the interactions between cancer cells and their surrounding cells is pivotal. These interactions are not just biological; they can dictate the course of tumor development and treatment responses. Cancer cells communicate with their environment through a variety of mechanisms:
- Paracrine Signaling: Cancer cells release signaling molecules that influence nearby cells, modifying their function. This can create a supportive environment that favors tumorigenesis.
- Cell-Cell Contact: Direct physical interactions with neighboring cells can alter cellular behavior and promote invasive capabilities. This is often seen with CAFs and immune cells.
- Extracellular Matrix Remodeling: Tumor cells can degrade the ECM to facilitate migration and metastasis, thereby reshaping their surroundings to suit their needs.
- Immune Evasion: Cancer cells develop strategies to avoid detection by the immune system, often by influencing immune cells to adopt a suppressive phenotype.
These interactions carve out a path that allows tumors to grow, metastasize, and resist therapies. A deeper understanding of these cellular dialogues opens opportunities for innovative treatments aimed not just at the cancer cells but also at modulating the TME to rekindle the body’s defense mechanisms.
"The microenvironment is not just a place where tumors grow; it is a dynamic battlefield of interactions that shape the fate of cancer cells and the host."
Cancer Progression and Metastasis
Cancer progression and metastasis are vital topics within the discourse of cancer biology. Understanding how cancer transitions from a localized malignancy to a potentially lethal widespread condition is crucial for diagnosis, treatment, and prevention strategies. Here, we will dissect the sequential stages of cancer development and delve into the mechanisms that enable tumor cells to migrate from their origin to distant sites in the body.
Stages of Cancer Development
Cancer is not a one-size-fits-all diagnosis. It evolves through several phases, each marked by a distinct set of characteristics:
- Initiation: This initial phase involves genetic changes that can result from a variety of factors, including environmental exposures or inherited mutations. For example, the presence of substances like benzene or UV radiation can light the fuse for these genetic alterations.
- Promotion: This stage sees the selective clonal expansion of initiated cells, which are now primed for growth. Factors exacerbating this phase might include chronic inflammation or certain hormone levels. It is like throwing logs into a fire; while the flame is already there, more fuel encourages it to blaze into a full-blown inferno.
- Progression: Here, the cancerous cells further change, accumulating more mutations that enhance their malignancy. These changes enable them to grow unregulated and to invade surrounding tissues. The process is akin to a weed infiltrating a garden, overtaking the space and choking the life out of surrounding plants.
- Metastasis: This is the grave culmination of cancer progression. It occurs when cells break away from the primary tumor, travel through the bloodstream or lymphatic system, and establish new tumors in different organs. The spreading characteristics of certain tumors mimic a dandelion's resilience. A single puff can release many seeds far and wide, leading to new growths across the landscape.
Mechanisms of Metastasis
Metastasis is not a random act; it follows a distinct path and involves multiple steps that can be categorized into several mechanisms:
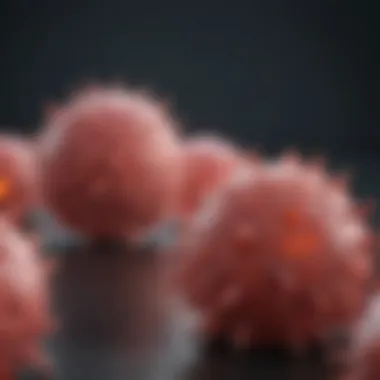
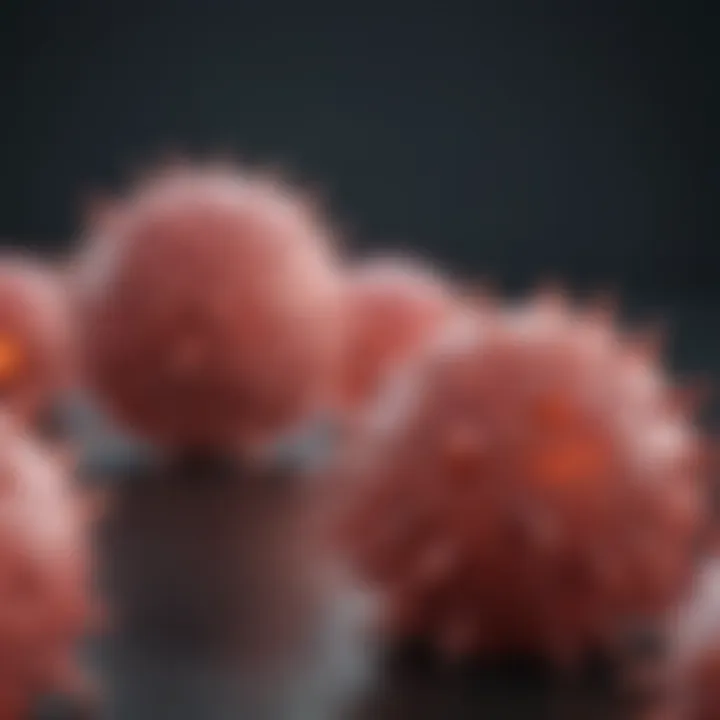
- Local invasion: Tumor cells invade surrounding tissues and prepare to migrate. They might use enzymes like matrix metalloproteinases to degrade the extracellular matrix and create a pathway.
- Intravasation: This involves tumor cells entering the bloodstream or lymphatic vessels. They can hitch a ride with white blood cells or can form aggregates that shield them from the immune system, much like a wolf in sheep's clothing.
- Circulation: Once in circulation, cancer cells encounter various obstacles, including immune clearance. Most cells do not make it through this phase, but those that do exhibit unique traits that allow them to evade destruction.
- Extravasation: Upon arriving at distant sites, some tumor cells exit the bloodstream, effectively landing and seeding themselves into new tissue. They resume their growth with the support of the local tumor microenvironment.
Understanding these metastatic mechanisms can lead healthcare professionals towards innovative and targeted therapies that might prevent or slow down cancer spread.
The journey from normality to a malignant state through stages of development and subsequent metastasis showcases the adaptive capabilities of cancer cells. As researchers dig deeper into these processes, the hope grows for more effective strategies in treating and managing cancer.
Therapeutic Implications
Understanding the therapeutic implications of cancer treatment is multifaceted and critical in navigating the complexities of cancer pathology. This section aims illuminating the pivotal role treatment options play in the battle against cancer cells while forming a stark contrast with normal cells. Breakthroughs in the therapeutic landscape are not just about offering hope but are also reflections on how various treatment paradigms can shape patient outcomes and enhance the quality of life. The specificity and effectiveness of treatments hinge on grasping the differences in cellular behavior between cancerous and healthy cells.
Current Treatment Modalities
Current treatment modalities have evolved with the understanding that cancer cells operate under distinct mechanisms compared to normal cells. Traditionally, the primary treatments available have included:
- Surgery: The removal of tumors and surrounding tissues. This is often the first line of treatment when the cancer is localized.
- Chemotherapy: Utilizes drugs to kill rapidly dividing cells, impacting both cancerous and some normal cells, leading to side effects like nausea and hair loss.
- Radiation Therapy: Applies high doses of radiation to specific areas to damage the DNA of cancer cells, preventing growth and division.
- Immunotherapy: Designed to enhance the body's own immune response against cancer, allowing normal cells to better distinguish themselves from cancer cells. This includes checkpoint inhibitors, which release the breaks on immune cells, enabling a stronger attack on tumors.
Each of these modalities targets the mechanisms unique to cancer cells, focusing on their rapid growth and division patterns. However, they also underscore the balance required to minimize effects on normal cells. Recent evidence indicates that the sequential and combination treatments often yield better results, presenting a two-pronged approach to combating cancer.
"The complexity of cancer treatment lies in distinguishing between normal and cancer cells while maximizing treatment efficiency."
Emerging Therapies Targeting Cancer Cells
The horizon of cancer research has opened doors to emerging therapies that are tailored specifically to target the unique attributes of cancer cells:
- Targeted Therapy: Involves new drugs that specifically target vulnerabilities in cancer cells, such as mutations that enable cancer growth. For instance, drugs like imatinib have transformed treatment for chronic myeloid leukemia by directly inhibiting the abnormal protein produced by the cancerous cells.
- Gene Therapy: This approach aims at correcting genetic abnormalities that lead to cancer. By inserting specific genes into a patient's cells, the modified cells can counteract cancerous growth more effectively.
- CAR-T Cell Therapy: A groundbreaking technique where a patient's T cells are modified to express chimeric antigen receptors. This allows T cells to better identify and destroy cancer cells, demonstrating significant success in certain hematologic malignancies.
- Nanotechnology: Using nanoparticles to enhance the delivery of drugs directly to tumor cells minimizes the impact on healthy cells, potentially reducing side effects and increasing treatment efficacy.
These innovative therapies not only illuminate the precision medicine approach but also highlight a shift towards individualized care. As the research continues, adapting these therapies to the unique genetic landscape of each patient's tumors could redefine cancer treatment paradigms, offering pathways to better outcomes.
Future Directions in Cancer Research
As research in the field of oncology continues to advance, understanding the future directions in cancer research is paramount. It allows students, researchers, educators, and professionals to grasp emerging trends and technologies that could reshape how we diagnose and treat cancer. Investing in innovative strategies holds the potential to not only improve patient outcomes but also contribute significantly to the reduction of cancer incidence and mortality on a global scale.
Innovative Research Approaches
In the quest to understand cancer more deeply, researchers are developing innovative approaches that push the boundaries of what we know. One promising direction involves the use of artificial intelligence and machine learning. These technologies can analyze vast amounts of data at speeds beyond human capabilities, revealing subtle patterns that may inform early cancer detection or identification of novel therapeutic targets.
Another exciting avenue is the application of systems biology. This integrates various biological datasets—genomics, proteomics, and metabolomics—to create a more holistic view of cancer. This comprehensive understanding of how different cellular components interact can lead to the discovery of new biomarkers for early diagnosis or prognosis.
Moreover, advancements in CRISPR and gene-editing technologies allow researchers to precisely alter genes in cancer cells. This can facilitate the study of specific oncogenes and tumor suppressor genes in detail, opening pathways for developing targeted therapy strategies. The ability to visualize and manipulate proteins or other cellular components in real-time increases our understanding of cancer dynamics, leading to potential breakthroughs.
Personalized Medicine and Cancer Treatment
Personalized medicine is rapidly emerging as a cornerstone of modern cancer treatment. This approach tailors therapies to the individual characteristics of each patient’s cancer, taking into account genetic, environmental, and lifestyle factors. For instance, oncologists now utilize genomic profiling to identify mutations that drive a patient’s specific cancer. Drugs can then be selected based on the unique genetic markers identified in a tumor, increasing treatment efficacy and minimizing adverse effects.
The rise of immunotherapy is another dimension of personalized medicine that has demonstrated efficacy in treating certain forms of cancer. By harnessing the patient’s own immune response, researchers develop therapies that can adapt to the unique aspects of each case. This has led to significant advances—some patients experience remarkable responses where conventional therapies fail.
Yet, the journey towards personalized cancer treatment comes with its own set of challenges. Variability in patient response can complicate therapy choices and necessitates ongoing research to uncover why some individuals benefit more than others. To this end, understanding the tumor microenvironment becomes crucial, as it can significantly influence therapy responses.
A key component of successfully implementing personalized treatment strategies involves an enhanced understanding of patient databases, ethical concerns, and ensuring accessibility to cutting-edge treatments. As such, ongoing discourse around these issues will be vital as we forge ahead.
"Personalized medicine is turning the tide in cancer treatment, making it more precise and effective, reducing the one-size-fits-all mentality that has pervaded oncology for decades."
In summary, future directions in cancer research include innovative approaches like artificial intelligence, systems biology, and personalized medicine. Together, these themes signal an exciting era in cancer research, promising enhanced understanding and improved strategies for combating this complex disease.
Closure
As we draw the curtain on our exploration, it's crucial to underscore the significance of understanding the differences between cancer cells and normal cells. This topic doesn't merely scratch the surface of cellular biology; it digs deep into the very mechanisms that govern life and health. This article has aimed to illuminate key elements, including structural and functional disparities, metabolic variations, and the intricacies of the tumor microenvironment, all pivotal in comprehending cancer biology.
Summarizing Key Differences
Understanding the distinctions between cancer cells and normal cells provides a foundational perspective for research and treatment pathways. Here are some noteworthy contrasts:
- Growth Patterns: Normal cells typically adhere to their regulatory cues, growing and dividing only when necessary, ensuring proper tissue maintenance. In contrast, cancer cells often disregard these signals, leading to uncontrolled proliferation.
- Cell Cycle Regulation: Normal cells follow a well-defined cycle, meticulously regulated by checkpoints, while cancer cells may have faulty checkpoints, enabling unchecked division and growth.
- Metabolic Processes: Normal cells utilize oxidative phosphorylation for energy production, whereas cancer cells often engage in altered metabolic processes, like aerobic glycolysis, favoring rapid growth.
- Apoptosis: Normal cells can undergo programmed cell death when damage occurs, whereas cancer cells frequently develop resistance to apoptosis, allowing tumors to persist.
The ramifications of these differences ripple through treatment strategies, influencing how therapies are designed and implemented.
Implications for Future Research
The path ahead in cancer research is full of potential discoveries and advancements. Understanding cancer's complexity compels researchers to focus on a few critical areas:
- Personalized Medicine: Tailoring treatments based on an individual’s cancer profile could revolutionize how we approach therapy. It amplifies the need for more research into genetic variance among patients.
- Targeting the Tumor Microenvironment: The tumor microenvironment's influence on cancer cell behavior poses important questions. Future studies that examine this interplay could yield significant insights, perhaps uncovering new treatment angles.
- Biomarker Development: Identifying concrete biomarkers for early detection and treatment response can enhance our capability to combat cancer effectively.
- Innovative Therapies: From gene editing technologies like CRISPR to immunotherapy advancements, ongoing research is essential in spearheading creative solutions to treat and manage cancer effectively.